Fig. 10.1
Relationship between prostate cancer volume and Gleason score (ERSPC-Rotterdam data). The columns show the number of cases (n) visualizing the percentage of Gleason score 6, 7, and 8–10 for each total volume category. The different colors represent the Gleason scores (From Van der Kwast and Roobol [6])
The use of any definition of pathologically insignificant prostate cancer requires the examination of the entire prostate, ex vivo, since no accurate tumor volume measurement is available at this time point. In vivo, current MR imaging tools may provide an assessment of prostate cancer volume of “visible” tumors, characterized as Gleason 6 by standard and targeted biopsy findings. Tumor volume estimation of visible tumors is relevant not only to the eligibility of men for active surveillance but also for monitoring patients on active surveillance and detecting progression. Strictly monitoring these clinical and pathological characteristics over time would identify risk reclassification that would justify radical treatment [8]. If accurate assessment can be obtained by MRI, eligibility criteria could be adjusted and tumor conspicuity and tumor volume monitoring could reduce surveillance biopsies.
An unmet need is to accurately and robustly assess tumor volume in prostate cancer. In this review, we investigated the current literature focusing on the relationships between MRI volume measurements and the underlying composition of normal and malignant prostate tissue , to determine if the integration of diagnostic MR imaging would improve target delineation and volume assessment for men with prostate cancer on active surveillance.
PI-RADS Version 2 Recommendations for MRI Tumor Volume Estimation
The PI-RADS (Prostate Imaging-Reporting and Data System) standardized image acquisition and reporting is designed to be used by medical professionals in the initial evaluation of patients to assess the risk of clinically significant prostate cancer leading to biopsy and treatment [9]. The longest axis tumor diameter was more strictly introduced in the PI-RADS version 2 [10], with the cutoff of 15 mm between scores 4 and 5 on T2-weighted images. Although this PI-RADS scoring system was not designed for tumor volume assessment, some minimal requirements on tumor volume estimation were introduced, reporting the largest dimension of a suspicious finding on an axial image. If the largest dimension of a suspicious finding is on sagittal and/or coronal images, this measurement and imaging plane should also be reported. If a lesion is not clearly delineated on an axial image, the measurement on the image which best depicts the finding should be reported. If preferred, lesion volume may be determined using appropriate software, or three dimensions of lesions may be measured so that lesion volume may be calculated (max. a-p diameter × max. l-r diameter × max. c-c diameter × 0.52).
Peripheral zone lesions should be measured on apparent diffusion coefficient (ADC) , reconstructed from diffusion-weighted MR images. Transition zone lesions should be measured on T2-weighted images. If lesion measurement is difficult or compromised on ADC (for peripheral zone) or on T2-weighted images (for transition zone), measurement should be made on the sequence that shows the lesion best.
Recently, the size threshold for the highest level of suspicion (score 5) in the PI-RADS version 2 was proposed to be reduced from ≥15 mm to ≥10 mm [11], corresponding the 0.5 cc for significant prostate cancer addressed by Wolters and colleagues. However, a clear size or volume threshold on MRI remains difficult to define to discriminate significant from insignificant prostate cancer in low-grade disease. Visibility on MRI (instead of actual tumor volume) could be of additional value in discriminating significant from insignificant prostate cancer.
PRECISE Recommendations for MRI Tumor Volume Estimation
Tumor volume estimation becomes more important if MRI is used in monitoring patients on active surveillance. In particular, stable disease on clinical parameters and MRI may defer or avoid systematic prostate cancer biopsies. In an international consensus meeting, recommendations have been developed to collect data in men having MRI on active surveillance (PRECISE criteria—Prostate Cancer Radiological Estimation of Change in Sequential Evaluation) [12]. Besides data on MRI suspicion score (Likert or PI-RADS v2) and the likelihood of progression being present, the absolute size of a lesion at baseline and follow-up should be reported, addressing the importance of MRI tumor volume assessment. However, to identify tumor progression on MRI, MRI tumor volume estimation should be robust. Today, no clear recommendations on MRI tumor volume assessment are present.
Index Lesion
It is suggested that all metastatic sites in a single patient may derive from a single monoclonal precursor cell [13], indicating that a single tumor focus is responsible for tumor progression and death, despite the multifocality of prostate cancer. This focus has been labeled the index lesion and has been considered to be defined by the largest tumor focus [14–16].
Increasing data supports the validity of the index lesion as the driver of prognosis and any adverse oncologic outcome and therefore necessitates consistently localizing the index lesion in each patient. MR imaging may offer a more robust evaluation of the entire prostate that can facilitate index lesion localization.
Index lesion identification by MRI has been proven to be well associated with the largest tumor focus in histopathology analysis, with correct identification and localization of 94–98% by MRI [17, 18]. Clearly, these high percentages of correct index lesion identification may be dependent on tumor size and Gleason grade.
Intuitively, one might think that tumors grow expansively in all directions (rounded volume); however, in reality, prostate cancer may spread/diffuse through the tissue or along the prostatic border. This may hamper the volume estimation by maximum diameter measurements on imaging. Planimetric volume measurements (delineation of the tumor at each axial slide) would be most accurate; it will take significantly more time during reporting in radiological practice, and sufficient software should be available. Furthermore, for lesions best seen on DWI/ADC image sequences, a single diameter may be more reproducible than a volume because of the need to use larger voxel sizes in sequence acquisitions.
If volume on MRI could be estimated from an ellipsoid or oval tumor, the axial diameter of a 0.5 cc, 1.3 cc, and 2.5 cc tumor would be approximately ~10 mm, ~14 mm, and ~17 mm, respectively. Robustness of MRI measurements is crucial for MRI tumor volume estimation.
Prognostic Value of Tumor Volume Assessment in Radical Prostatectomies
The clinical significance of prostate cancer tumor volume in radical prostatectomy specimens is controversial [19, 20]. In univariable analysis, prostate tumor volume is of prognostic significance, but most studies report that its prognostic significance is lost in multivariable analysis [21–25]. This may be attributed to the close correlation of tumor volume with Gleason score, T-stage, and surgical margin status of radical prostatectomy specimens. Due to the lack of independent prognostic significance, most pathologists choose not to report routinely an exact measure of tumor volume [26]. Despite this controversial issue and difficulties in accurately measuring tumor volume, pathologists agreed that some form of semiquantitative measurement of tumor volume should be reported, although no consensus was reached regarding method(s) [5].
Tumor Volume Assessment in Radical Prostatectomies
Although there are many methods available to calculate prostate tumo r volume, obviously, computer-assisted image analysis systems (planimetrically) are considered the most accurate means of determining tumor volume [27]. Due to the high labor intensity, these planimetric methods are not commonly used in routine pathology practice. Alternative methods for the estimation of tumor dimensions of tumor volume (or size) rely on calculation of basic tumor dimensions [28–31]. In routine pathology laboratories, the ellipsoid formula (0.52 × length × width × height) (Fig. 10.2) may provide a close estimate of the tumor volume [31], as compared to planimetrically determined tumor volume by computer image analysis, with sensitivity and specificity of, respectively, 94% and 92% for tumor volume >0.5 cc. However, others showed the ellipsoidal method may overestimate tumor volume by approximately 30% [28, 29] and would use the formula 0.4 × length × width × height. This ellipsoid measurement is simple and reproducible and is considered appropriate for pathological tumor measurements. Nevertheless, it is not routinely applied, except in a research setting. Most clinicians do not use the measurement of cancer volume by this technique in clinical decision making, and it is not currently recommended.


Fig. 10.2
Illustration of measurements obtained on pathological dissection. CST cross-sectional thickness, L length, W width (Reprinted from Perera and colleagues [31], with permission from John Wiley & Sons)
MRI Signal Intensities and the Underlying Tissue Composition in Radical Prostatectomies
Relationships between MRI signal intensities and the underlying architectural prostatic tissue are complex. Langer and colleagues showed the association between specific alterations in tissue composition and MR imaging measurements [32]. They have shown that the increased percentage area of nuclei and cytoplasm, in combination with the decreased percentage area of luminal space, within equal stroma, corresponds to a decrease in T2-weighted and ADC signal intensities (Figs. 10.3 and 10.4). Furthermore, these morphological changes also correspond to the increase of the DCE-MRI volume transfer constant [Ktrans] and decrease to the DCE-MRI extravascular extracellular volume fraction [v e] (Figs. 10.3 and 10.4).



Fig. 10.3
Histologic samples from normal and malignant regions. Loose stromata are indicated with +; benign glands, with *; and malignant glands, with arrows. (a) Normal PZ tissue is characterized by a mixture of loose stroma and benign glands. Sparse regions in tumors consist of normal PZ tissue infiltrated by scattered malignant glands. (b) Malignant glands are intermixed with benign glands and loose stroma, and (c) a line of malignant glands traverses through otherwise normal loose stroma. (d–f) In contrast, dense regions in tumors consist of (d) a high proportion of malignant glands, (e) malignant glands mixed with desmoplastic stromata, or (f) dense smooth muscle tissue, uncharacteristic of normal PZ tissue and visible as solid staining. (Hematoxylin-eosin stain; magnification, ×100) (From: Langer et al. [33]. Reprinted with permission from the Radiological Society of North America (RSNA®))

Fig. 10.4
Graphs illustrate mean slopes for MRI signal intensities versus proportion of cellular component (nuclei, cytoplasm, stroma, and luminal space) (Data from Langer and colleagues [32]; see Table 3)
They also determined that the number of cellular components is significantly different between malignant and benign peripheral zone tissue, and thus these mechanisms influence prostate cancer detection with MR imaging. T2-weighted imaging is sensitive to extracellular water; ADC is sensitive to diffusion in the lumen; thus increased cellular texture corresponds to decrease in T2-weighted and ADC signal intensities. Because DCE-MRI-derived parameters are expected to be related to properties of the vasculature (rather than cellular composition), changes in cellular components occur in abnormalities associated with vascular density. Figure 10.4 shows graphically the signal intensity decays and increases of T2w, ADC, Ktrans, and ve, based on their published mean slopes for MRI measurements versus proportion of cellular components. Other groups have also shown this correlation between ADC and cellular density [34, 35] and between contrast MR imaging parameters and microvessel density [36] on radical prostatectomy specimen.
MRI-derived parameters are reflective of pathologically determined characteristics of prostate cancer; however, there is great overlap with benign conditions, such as benign prostate hyperplasia, inflammation, or fibrosis. For example, nucleomegaly and increased cellular density, in addition to indicating prostate cancer, can also be indicative of inflammation and prostatic intraepithelial neoplasia. Luminal size can be altered in cystically dilated glands, regions of atrophy, or fibrosis. These benign abnormalities have been implicated as sources of false-positive MR imaging findings [37–40] or poor radiologic-pathologic volumetric correspondence [41].
In all such studies, MRI measurements have been determined unblinded to pathology results of prostatectomy specimen. Furthermore, in these studies, the impact on tumor detection was not investigated. In clinical practice, this detailed knowledge of tumor distribution obtained from prostatectomy specimen is of course not at hand at the time of prospective patient management. Therefore, it is the relationship between tumor detection and histopathological features that is of clinical relevance.
Visible Tumor Detection on MRI in Radical Prostatectomies
Definitely, tumor size and tumor aggressiveness may have serious impact on tumor detection on MRI. Vargas and colleagues found that the integrated PI-RADS v2 scores resulted in the correct classification of 94% (118/125) peripheral zone tumors and 95% (42/44) transition zone tumors with ≥0.5 cc on pathology with any Gleason grading [42]. This correct classification was limited for the assessment of small tumor volumes of ≤0.5 cc with Gleason ≥4 + 3. The majority of GS ≥4 + 3 tumors with volumes <0.5 cc on pathology were not detectable on MRI; MRI was only able to identify 26% (7/27) peripheral zone tumors and 20% (2/10) transition zone tumors (suspicion scores 4 and 5, PI-RADS v2). This is not surprising: previously this group showed that lesion detectability on MRI was volume dependent and Gleason grade dependent [43]. Nonetheless, this is important to consider the limited ability of MRI to detect small lesions. This was a retrospective analysis biased by the inherent limitations of such a study design. Most likely, without the knowledge of the presence of prostate cancer, prospective studies may show even worse outcomes for MRI lesion detection of <0.5 cc. These findings have implications for the use of MR imaging in the management of patients with clinically low-risk prostate cancer, who now constitute about one-half of all patients diagnosed with prostate cancer in the Western world [44].
Bratan and colleagues found similar results [45]. They explored the tumor detection rates of peripheral and transition zone tumors on MRI as a function of histological parameters. MRI detection rates were 57–63% and 36–41% for all peripheral zone tumors and transition zone tumors, respectively, with small interobserver variation between two readers. They found an increased tumor detection rate on MRI, related to increased histology tumor volume: MRI tumor detection rates of 28%, 38%, 76%, and 96% corresponded to <0.05 cc, 0.05–0.5 cc, 0.5–2.0 cc, and >2.0 cc on planimetry histology analysis. Although the ellipsoid tumor volumes 0.05 cc, 0.5 cc, and 2.0 cc may correspond to an axial diameter on MRI of approximately 5 mm, 10 mm, and 16 mm, most likely these diameters would not always be measured on MRI, as underestimation is known when the tumor phenotype is not represented by dense tissue. Interestingly, this group also investigated the MRI tumor detection rate in relation to histological architecture. Increased MRI tumor detection rates of 35%, 40%, 62%, and 66% corresponded to lobulated, infiltrative, mixed, and dense tissue on histology analysis. Furthermore, increased MRI tumor detection rates of 38%, 76%, and 96% corresponded to increased Gleason scores 6, 7, and 8–9. From these data, we may conclude that prostate cancer location, histological volume, Gleason score, and histological architecture were independent significant predictors of tumor detection on multiparametric MRI.
Non-visible Tumor on MRI in Radical Prostatectomies
In some patients, MRI is not able to visualize an index tumor. In some studies, these tumors proved to be microscopic at histopathology with volumes almost all below the 0.2 cc [17, 46]; however, in other studies, these tumors proved to be larger than 0.2 cc [42, 45, 47]. Although patients with small tumors may be better candidates for active surveillance, irrespective of low-risk or intermediate-risk disease, non-visible tumors are not always small tumors.
Langer and colleagues showed that no significant differences in ADC or quantitative T2-weighted values were present between the surrounding normal peripheral zone tissue and the “sparse” prostate tumors, which contain a high percentage of normal peripheral zone tissue, intermixed with prostate cancer [33]. The presence of regions within prostate tumors that are intrinsically invisible by using T2-weighted and ADC-based tissue contrast may limit accurate determination of tumor volume and target definition for active surveillance but also for MRI-driven targeted biopsies or focal therapy.
Some histological characteristics are predisposed to be more visible by MRI. Rosenkrantz and colleagues described the presence of “solid tumor growth” as a key contributor to tumor detection on MRI that was present in only 58% of their investigated tumors [48]. Although there was a significant difference between the detected and missed tumors on MRI for all assessed histological features (i.e., loose stroma, desmoplastic stroma, solid tumor growth), only size, Gleason score , and the presence of solid tumor growth were independent predictors for MRI visibility on multivariate analysis. Solid tumor growth had a substantially high odds ratio (17.83) after accounting for the effects of other features, supporting the particular importance of the formation of a discrete nodule of continuous tumor growth to facilitate MRI detection. They hypothesized that the inability to visualize a known tumor on MRI may indicate the absence of a distinct nodule of packed malignant glands encompassed by the tumor. In addition, desmoplastic tumor-associated stroma was present in all detected tumors, supporting the important role of stromal as well as epithelial components in tumor detection by MRI [48].
Radiologic-Pathologic Volumetric Correspondence in Radical Prostatectomies
Although MRI has the big advantage of in vivo tumor volume estimation and could therefore be valuable in diagnosis, in monitoring, and in treatment decision-making, critical analysis of the literature shows still some big hurdles to overcome.
High Range of Disagreement in Tumor Volume Estimation
Baco and colleagues indicated that the limit of agreement between MRI and histopathology tumor volume estimation of all prostate cancer lesions ranged from 147 to +135% (Fig. 10.5), which indicates clinically significant inaccuracy for MRI tumor volume estimation in clinical practice, as well as in overestimation and in underestimation [46]. Although there was a positive correlation between estimated MRI and histopathology tumor volume (r = 0.663, p < 0.001) in 135 radical prostatectomy specimens, this should be cautiously interpreted within the clinical context of high range of disagreement. These results were similar to previous results published by Turkbey in also 135 radical prostatectomies [17], Matsugasumi in 81 [49], and Mahazeri in 42 radical prostatectomies [50].


Fig. 10.5
(a) Scatter plot showing correlation between MRI-estimated tumor volume (MTV) and histological tumor volume (HTV ) in 128 patients. The red line indicates the regression line. Data are presented on a logarithmic scale because of asymmetry. (b) Bland-Altman plot showing the limitation of agreement between MTV and HTV. The orange line represents the linear regression line. The percentage difference between MTV and HTV is plotted against the average tumor volume (calculated from both MTV and HTV). All values above the zero line represent overestimation of MTV, and all values below the zero line represent underestimation of MTV. The average underestimation of HTV by MRI is 5.9% (95% CI [S6.4% +18.2%]) and is constant throughout the measurement range. The limit of agreement ranges from S147 to +135%, which indicates clinically significant inaccuracy for MTV. The median (range) is 1.0 ml (0.1–20.0 ml) for MTV and 1.25 ml (0.1–27.1 ml) for HTV. (c) Scatter plot demonstrating correlation between the maximum cancer core length on targeted biopsy and HTV in 128 patients. The red line indicates the regression line (Reprinted from Baco and colleagues [46], with permission from Elsevier)
MRI Tumor Volume Underestimation in Small and Large Tumors
Le Nobin and colleagues reported significant underestimation in advanced 3 Tesla imaging and co-registration software, not only in small tumors (<1 cc) (range,133% to +85% based on T2w images) but also in large tumors (>1 cc) (range,122% to +24%) [51]. Tumor volume estimation by MRI was also underestimated in lesions with an MRI suspicion score of 4 or 5 (mean difference,45%; range, 97% to +7%) more than in lesions with suspicion score of 2 or 3 (mean difference, +2%; range, 107% to +111%). Tumor volume estimation by MRI was even underestimated in lesions with a Gleason score 7 and higher (mean difference, 39%; range, 104% to +26%), than in lesions with a Gleason score 6 (mean difference, 5%; range, 96% to +87%).
Others have also shown an overall but wide variety of underestimation of MRI and histopathology tumor volume estimation, with a large range from overestimation to underestimations between those two measurements [17, 46, 47, 52]. Overestimation could be explained by inaccuracies of imaging as well as shrinkage of the histopathological specimen due to fixation and mounting. Applying shrinkage correction (15%), however, had very little impact on the overall tumor volume agreement due to the very large standard deviations [17, 52].
The overall underestimation of tumor volume on MRI may relate to the findings of Langer and colleagues [33], which observed that prostate tumors contain regions of “sparse” malignant epithelium intermixed with mostly benign glands and stroma. These “sparse” regions were characterized as inherently invisible on MRI and posing limits on the ability to estimate full tumor volume with MRI.
MRI Tumor Volume Underestimation in High MRI Suspicion Scores and High Gleason Grading
This degree of underestimation was even more pronounced for tumors with a higher Gleason score and higher MRI suspicion score ` [51]. Indeed, based on extensive previous literature showing associations between both ADC value and MRI detection with higher Gleason score, MRI would have been expected to be more reliable in estimating tumor volume for more aggressive tumors. These findings, however, were based on studies focusing on the detection of prostate cancer, rather than the determination of tumor volume estimation. Others have also shown poor correlation and agreement of MRI tumor volume estimation of the higher Gleason grades with pathology tumor volume measurements [17, 52].
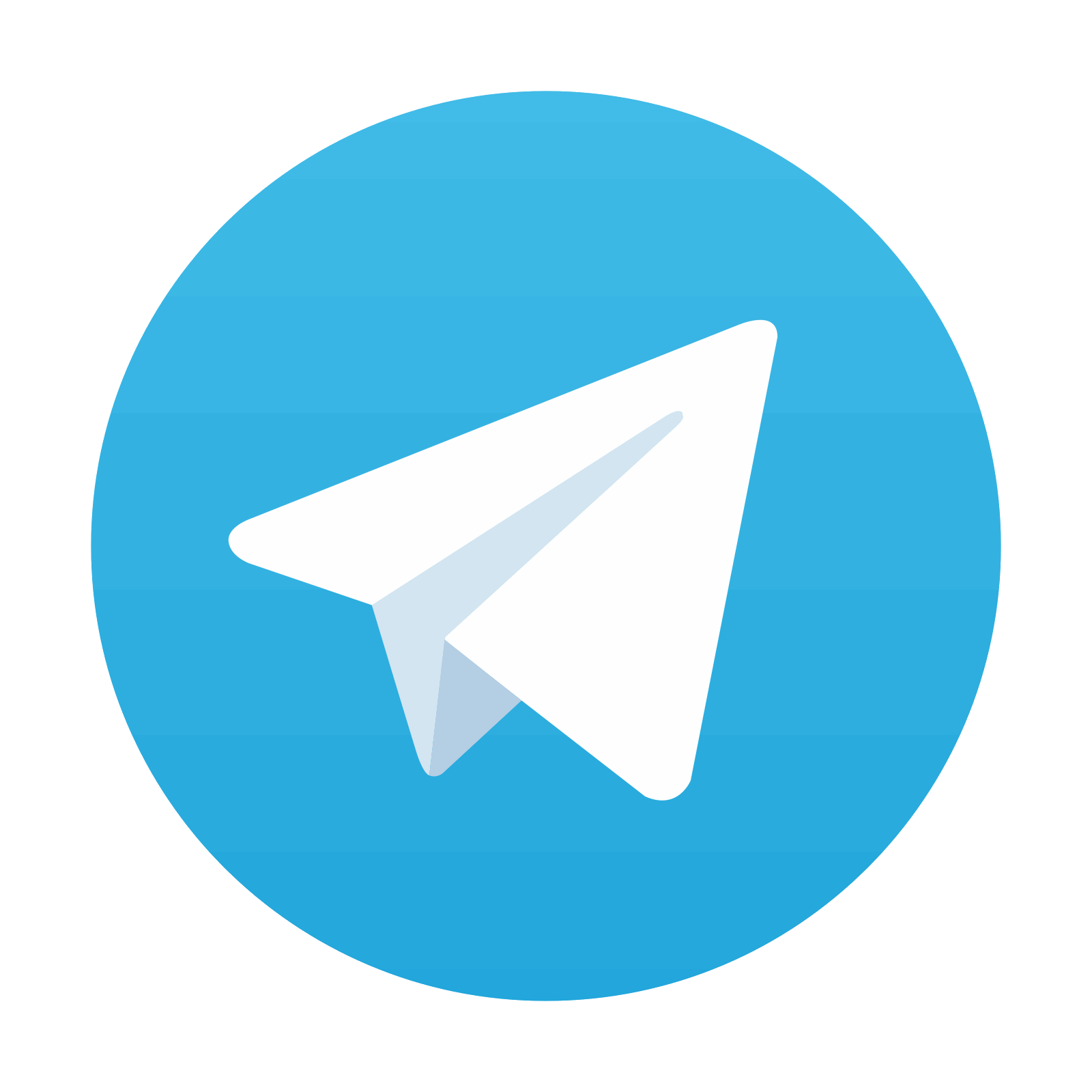
Stay updated, free articles. Join our Telegram channel

Full access? Get Clinical Tree
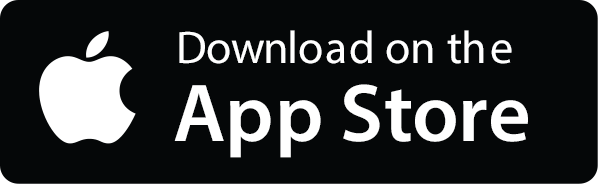
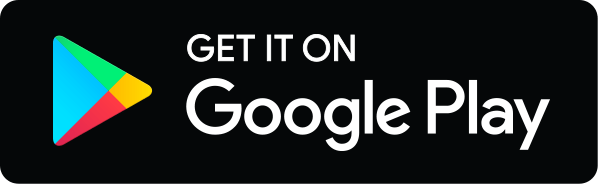