Helicobacter pylori infection plays a crucial role in gastric carcinogenesis. H pylori exerts oncogenic effects on gastric mucosa through complex interaction between bacterial virulence factors and host inflammatory responses. On the other hand, gastric cancer develops via stepwise accumulation of genetic and epigenetic alterations in H pylori -infected gastric mucosa. Recent comprehensive analyses of gastric cancer genomes indicate a multistep process of genetic alterations as well as possible molecular mechanisms of gastric carcinogenesis. Both genetic processes of gastric cancer development and molecular oncogenic pathways related to H pylori infection are important to completely understand the pathogenesis of H pylori -related gastric cancer.
Key points
- •
Helicobacter pylori -related gastric carcinogenesis is associated with interactions between bacterial virulence factors and host inflammatory responses.
- •
Comprehensive analyses of gastric cancer genomes provide us with clues to identifying the molecular pathogenesis of gastric carcinogenesis as well as therapeutic targets.
- •
The expression of activation-induced cytidine deaminase (AID) in gastric epithelium induced by H pylori infection and resultant inflammation have a crucial role in the induction of genetic alterations during H pylori -related gastric carcinogenesis.
Introduction
Gastric cancer is the third leading cause of cancer-related death worldwide. Helicobacter pylori infection is the most common causative factor of gastric cancer among various factors including host genetic and environmental factors. Since its discovery in 1984 by Marshall and Warren, many epidemiologic studies have investigated the relationship between H pylori and gastric cancer. Also, many investigators have revealed complex interactions between the virulence factors of H pylori , resultant chronic inflammation, and gastric carcinogenesis. Recently, advances in sequence technology revealed the whole picture of human gastric cancer genome. These results can contribute to uncovering the molecular mechanisms of gastric carcinogenesis and also to the targeted therapy for gastric cancer. This review focuses on the recent developments in the molecular mechanisms of H pylori -related gastric carcinogenesis.
Introduction
Gastric cancer is the third leading cause of cancer-related death worldwide. Helicobacter pylori infection is the most common causative factor of gastric cancer among various factors including host genetic and environmental factors. Since its discovery in 1984 by Marshall and Warren, many epidemiologic studies have investigated the relationship between H pylori and gastric cancer. Also, many investigators have revealed complex interactions between the virulence factors of H pylori , resultant chronic inflammation, and gastric carcinogenesis. Recently, advances in sequence technology revealed the whole picture of human gastric cancer genome. These results can contribute to uncovering the molecular mechanisms of gastric carcinogenesis and also to the targeted therapy for gastric cancer. This review focuses on the recent developments in the molecular mechanisms of H pylori -related gastric carcinogenesis.
Classifications and characterizations of gastric cancer
Gastric cancer is histologically heterogeneous and classified by various histologic classification systems. The commonly used classifications are those of Lauren and the World Health Organization. The former classification is composed of 2 subtypes, intestinal type and diffuse type, and the latter classification has 4 subtypes, papillary, tubular, mucinous, and poorly cohesive. Intestinal-type gastric cancer shows cohesive groups of tumor cells with a glandular architecture. These types of cancers typically generate from H pylori -infected gastric mucosa with chronic gastritis, atrophy, and metaplastic changes. While intestinal metaplasia has been focused as a precursor to gastric cancer, recently, spasmolytic-polypeptide-expressing metaplasia (SPEM) has also been highlighted as another metaplastic lesion. SPEM is generated through the transdifferentiation of chief cells after parietal cell loss due to H pylori infection and gives rise to intestinal metaplasia in the presence of inflammation. SPEM is thought to be the initial preneoplastic metaplasia predisposing to gastric cancer, although further analysis is needed. Diffuse-type gastric cancer, by contrast, is composed of scattered poorly cohesive cells with poor cellular differentiation. This type emerges in H pylori -infected mucosa with or without atrophic and metaplastic changes as well as the mucosa unrelated with H pylori infection.
On the other hand, recent innovative technologies enable us to classify gastric cancers based on tumor molecular biology. Several reports revealed that gastric cancer is genomically heterogeneous and is classified into several types by comprehensive molecular evaluation, contributing to understanding not only the molecular pathogenesis of gastric carcinogenesis but also the targets for personalized therapy. The Cancer Genome Atlas research network proposes that gastric cancer is divided into 4 subtypes: tumors that test positive for Epstein-Barr virus (EBV), tumors with microsatellite instability (MSI), tumors with chromosomal instability (CIN), and genomically stable (GS) tumors. EBV-positive cancers display PIK3CA mutations, extreme DNA hypermethylation, and amplification of JAK2 , PD-L1 , and PD-L2 . MSI tumors have epigenetic silencing of MLH1 , one of DNA mismatch repair genes, in the context of a CpG island methylator phenotype. MSI can lead to subsequent genetic changes, including small insertions and deletions, in hundreds to thousands of genes. It has been reported that the frequency of MSI was higher in intestinal-type gastric cancer, old-aged women, and distal gastric cancer, and these tumors are usually diagnosed at the earlier stage. Interestingly, early gastric cancer genomes with MSI showed a comparable level of mutations to advanced MSI gastric cancer in terms of the number, sequence composition, and functional consequences of mutations. These results suggest that the genetic or epigenetic alterations characterized as MSI may already be achieved in early gastric cancer genomes. CIN tumors account for 50% of gastric cancers, and most of them are histologically of the intestinal type. These types of cancers typically have TP53 mutations and marked aneuploidy and focal amplification, including receptor tyrosine kinases, VEGFA, and cell cycle mediators ( CCNE1 , CCND1 , and CDK6 ). These genomic amplifications are possible targets for therapeutic inhibitors. Intriguingly, TP53 mutations are frequently seen in noncancerous gastritis mucosa with H pylori infection and various chromosomal aberrations are present in gastric adenoma. These findings suggest that TP53 mutations and various chromosomal alterations are early events during H pylori -related gastric carcinogenesis with atrophy-metaplasia-dysplasia sequence. GS tumors that lack the specific features are predominantly the diffuse histologic subtype, and half of them harbor mutations or fusion in CDH1 or RHO family genes.
In addition, Lei and colleagues classified gastric tumors into 3 subtypes by gene expression profile. Proliferative-type tumors have CIN, TP53 mutations, and DNA hypomethylation, consistent with CIN-type tumors. Tumors of mesenchymal type contain cells with features of cancer stem cells, suggesting that this type of cancer may generate from stem cell regions in gastric mucosa. Furthermore, metabolic-type tumors are likely to express genes characterized in SPEM, a particular kind of metaplasia in the stomach, which has been proposed as an intermediate step during gastric carcinogenesis. This fact suggests that these tumors may generate from metaplastic regions in H pylori -related gastritis.
Taken together, the combination of histologic and genetic analyses is essential for understanding the process of gastric cancer development. Although each cancer has a very different profile, these analyses provide several processes from early genetic events to progressive H pylori -related gastric carcinogenesis ( Fig. 1 ). In addition to these approaches for uncovering gastric carcinogenesis, molecular mechanisms of how H pylori infection induce emergence of cancer cells are also important.
Helicobacter pylori virulence factors on gastric epithelium during gastric carcinogenesis
Roles of CagA in Gastric Carcinogenesis
Among various virulence factors of H pylori that may be involved in gastric carcinogenesis, the cag pathogenicity island (cytotoxin-associated gene [cag] PAI) is a well-characterized molecule. Several genes within this island encode the CagA protein and the type IV secretion system (T4SS) that delivers bacterial agents into gastric epithelial cells. A large amount of clinical data showed that the cagA -positive strains are considered to be more potent in gastric cancer development than cagA -negative strains, although cagA -negative strains also cause gastric cancer. Importantly, the oncogenic potential of CagA was directly demonstrated by the observation that transgenic mice systemically expressing CagA protein spontaneously developed gastrointestinal carcinomas and hematopoietic malignancies. CagA is translocated into the cytoplasm of gastric epithelial cells by the T4SS during bacterial attachment. Once translocated into host cytoplasm, CagA may bind to the inner surface of the cell membrane, be tyrosine-phosphorylated at Glu-Pro-Ile-Tyr-Ala (EPIYA) motif by Src family kinases and Abl kinases, and then interact with a variety of human proteins to lower the threshold for neoplastic transformation. Phosphorylated CagA can specifically bind to the SH2-domain-containing protein tyrosine phosphatase (SHP2) and activate this molecule, leading to the activation of the Ras-Erk pathway. CagA-mediated SHP2 signaling also dephosphorylates focal adhesion kinase, a kinase regulating cell shape and motility, and then inhibits its kinase activity, causing impaired focal adhesions that are associated with an elongated cell shape known as the hummingbird phenotype and elevated cell motility. On the other hand, nonphosphorylated CagA also exerts effects within the cells that contribute to carcinogenesis. CagA inhibits PAR1 activity by binding this protein kinase, leading to the disruption of tight junctions and loss of cell polarity. CagA also interacts with E-cadherin via PAR1, leading to activation of β-catenin. In addition to PAR1, nonphosphorylated CagA can associate with the c-Met receptor tyrosine kinase, the phospholipase C-γ, and the adaptor protein Grb2. Taken together, CagA contributes to gastric carcinogenesis via the interaction with multiple signaling pathways.
Recently, it has been reported that CagA interacts with tumor suppressors, including RUNX3 and p53. Interaction of CagA with RUNX3 induces the ubiquitination and degradation of RUNX3 that impairs its ability as a tumor-suppressive transcriptional factor. CagA also interacts with the apoptosis-stimulating protein p53 (ASPP2) and prevents ASPP2 from inducing apoptosis through activation of p53. Together, CagA can act directly with tumor suppressors, contributing to malignant transformation of gastric epithelial cells.
CagA is also considered to mediate cell reprogramming. It has reported that CagA can deregulate Wnt signaling and then induce Wnt target genes, including CDX1, an intestinal-specific transcriptional factor. Interestingly, ectopically expressed CDX1 transactivates stemness-associated reprogramming factors SALL4 and KLF5 that can convert gastric epithelial cells into tissue stemlike progenitor cells, which then transdifferentiated into intestinal epithelial cells. Considering from the aspect of cancer stem cell theory, these results also suggest that cells dedifferentiated by CagA may become cells of origin of gastric cancer as cancer stem-cell-like cells.
Roles of Vacuolating Cytotoxin A in Gastric Carcinogenesis
The vacuolating cytotoxin A (VacA) is a toxin secreted by H pylori that is associated with increased disease risk. VacA has a variety of effects on epithelial cells, including vacuolation characterized by a collection of large vesicles as well as induction of apoptosis and suppression of T-cell responses, which results in the longevity of infection. VacA and CagA counterregulate the effects of each other on the host cells, contributing to effective mechanisms to promote persistent colonization of H pylori . One mechanism is that VacA induces autophagy-dependent degradation of CagA by triggering reactive oxygen species (ROS) via the binding of VacA m1 to low-density lipoprotein receptor-related protein-1 on host cells. Interestingly, CagA can escape from the ROS-induced autophagy in cells expressing a variant 9 form of CD44 (CD44v9), one of the gastric cancer stem cell markers because CD44v9 increases the level of intracellular glutathione that neutralizes ROS. Therefore, CagA is thought to be able to remain longer in gastric cancer stem cells, perhaps contributing to initiation and progression of gastric cancer. Taken together, H pylori can avoid the induction of excess cellular damage and maintain long-term persistence in host cells as well as increase oncogenic functions in cancer stem cell population even if H pylori is eradicated.
Role of Peptidoglycan in Gastric Carcinogenesis
In addition to CagA, components of H pylori peptidoglycan are also delivered into host cells through T4SS and trigger several signaling pathways related with carcinogenesis. Peptidoglycan is recognized by NOD1, an intracellular pathogen recognition molecule, and then leads to activation of nuclear factor (NF)-κB-dependent proinflammatory responses, such as secretion of interleukin (IL)-8, β-defensin2, and PI3K-AKT signaling, lowering the threshold for malignant transformation by decreasing apoptosis and increasing proliferation and cell migration.
Helicobacter pylori -induced inflammatory responses during gastric carcinogenesis
Inflammation has been well recognized as the key risk factor for many types of cancers. Helicabacter pylori infection causes inflammation of the gastric mucosa with inflammatory cell infiltration, including T cells and activated mononuclear cells. Helicobacter pylori -induced gastritis is characterized by the enhanced production of a variety of proinflammatory cytokines, such as IL-1β, tumor necrosis factor (TNF)-α, IL-6, IL-8, IL-11, and others, in the gastric mucosa, and these cytokines are thought to play important roles in cancer development. Among them, IL-1β has been focused as the factor that links gastric inflammation and gastric cancer. Polymorphisms in the IL-1β gene are linked with the increase of IL-1β production and risk of gastric cancer related with H pylori infection. IL-1β is upregulated by H pylori infection and induces NF-κB activation in both inflammatory and epithelial cells and hepatocyte growth factor.
In addition to IL-1β, TNF-α may increase the risk for gastric cancer. TNF-α polymorphisms that increase TNF-α production are associated with an increased risk of gastric cancer and its precursors. TNF-α produced by macrophages promotes Wnt/β-catenin signaling in gastric epithelial cells through inhibition of GSK3β. Also, the activation of TNF-α/TNFR1 signaling in the tumor microenvironment promotes gastric tumor development through induction of Noxo1 and Gna14, which contribute to maintaining the tumor cells in an undifferentiated state. Importantly, IL-1β and TNF-α enhance NF-κB activation, a key transcription factor mediating inflammation and cancer development. NF-κB activation promotes growth, suppresses apoptosis of epithelial cells, and stimulates the production of growth factors and cytokines such as epidermal growth factor and IL-6, enhances cyclooxygenase (COX)-2 induction, and increases ROS production. The induced COX2 has various functions, including enhancement of cell growth and angiogenesis.
IL-6 levels are also increased in the H pylori -induced gastritis mucosa. IL-6 activates signal transducer and activator of transcription 3 and thereby enhances cell growth and stimulates growth factor production, including RegIα.
Thus, these mediators of inflammation form a complex of regulatory networks and seem to work in concert to enhance cancer development.
Genetic and epigenetic alterations during gastric carcinogenesis
Mechanisms of Induction of Genetic Alterations
Comprehensive cancer genome analyses reveal not only a snapshot of current cancer status but also a footprint of its carcinogenesis process. Recent studies show that the mutation signature that accumulates in tumor tissues provides the clue to identifying the cause of genetic alterations during tumor development. Nucleotide alterations that accumulated in gastric cancer genomes were enriched with C:G>T:A transition, followed by C:G>A:T transversion. C:G>T:A transition mutations typically represent involvement of deamination process, although exogenous mutagens, such as UV light can also induce these mutations. C:G>A:T transversion mutations are considered to be caused by ROS and tobacco. Furthermore, most gastric cancers have chromosomal aberrations.
Deamination
The mechanisms that induce deamination are of 2 types, spontaneous deamination and activation of endogenous deaminase by the apolipoprotein B mRNA editing enzyme, catalytic polypeptide-like (APOBEC) family. Spontaneous deamination is the most frequent cause of spontaneously generated mutations, that is, deamination of 5-methylcytosine in CpG sequences, resulting in C:G>T:A transitions. This mutation signature is correlated with age and seen in almost all cancers of various tissues. Among these cancer types, gastrointestinal cancers, including gastric cancer, particularly have C:G>T:A mutations in the context of CpG. These results indicate that some factors that can promote methylation or deamination are involved in the elevated rate of these mutations in gastric cancer in addition to spontaneous methylation.
On the other hand, the APOBEC family members are cytidine deaminases that are capable of inducing nucleotide alterations by converting cytidine to uracil coupled to repair systems and DNA replication machineries. Among the APOBEC family members, AID can induce genetic alterations in human DNA sequences and plays a key role in generating immune diversity through induction of both somatic hypermutation and class-switch recombination in immunoglobulin genes. APOBEC3A and 3B also have the capacity to induce genetic alterations in human DNA. Although their normal functions are unknown, APOBEC3A and 3B are thought to elicit mutations in several cancer types, such as breast cancer and lung cancer. Similar to AID, these enzymes can deaminate C on target nucleotides to produce U, generating a U:G mismatch. The generated U:G mismatches can usually be repaired to C:G by the high-fidelity repair system. If the mismatch is not repaired before DNA replication, DNA polymerase will insert an A nucleotide opposite the U nucleotide, resulting in a C:G>T:A transition. Alternatively, recognition of a U:G mismatch by uracil DNA glycosylase or mismatch repair protein, such as MSH2 and MSH6, induces various patterns of nucleotide alterations. In contrast, nicks in the near sites of both strand sequences are generated by the repair process of generated U:G mismatches, resulting in DNA double-strand breaks followed by chromosomal aberrations.
While APOBEC3A and 3B favor C residues flanked by 5′-T, AID exhibits a strong preference for deaminating C residues flanked by a 5′-purine (G or A). In whole exome sequencing analyses of gastric cancer, the C:G>T:A mutations in gastric cancer genomes predominantly accumulated in the context of Gp C pX or Ap C pX ( C is the mutated base and X is any base) as well as CpG. In particular, these mutational signatures are more frequent in MSI gastric cancer, consistent with the mechanism that defect of mismatch repair genes enhances the characteristics of AID-mediated cytidine deamination. Furthermore, the predominant pattern of mutations in H pylori -infected gastritis is also C:G>T:A in Gp C pX motifs. These results suggest that AID is deeply involved in the induction of somatic mutations during H pylori -related gastric carcinogenesis.
Under physiologic conditions, AID expression is limited to activated B cells for inducing somatic hypermutation and class-switch recombination in immunoglobulin genes. By contrast, AID protein is aberrantly expressed in a substantial proportion of H pylori -associated human gastric epithelium and gastric cancer tissues, although no AID expression is observed in normal gastric mucosa. In particular, mononuclear cell infiltration and intestinal metaplasia correlate with AID expression. After eradication of H pylori , AID expression is significantly decreased but is still higher than that in H pylori -negative gastric mucosa. Intriguingly, infection with cag PAI-positive H pylori ectopically induces a high expression of AID in human gastric epithelial cell lines, whereas cag PAI-negative H pylori has no effect on AID expression. Also, inflammatory cytokines, such as TNF-α, increase the expression of endogenous AID protein in gastric epithelial cells through the NF-κB pathway. Furthermore, aberrant AID expression in gastric epithelial cells induced by these stimuli causes several somatic mutations in tumor-related genes, including the tumor suppressor TP53 gene. Consistent with this result, TP53 mutations are frequently seen in chronic gastritis mucosa with H pylori infection. These findings suggest that AID is a key molecule for the induction of somatic mutations during H pylori -related gastric carcinogenesis.
Oxidative stress
Generation of ROS and reactive nitrogen species (RNS) in human stomach are considered potential genotoxic factors in gastric mucosa. Helicobacter pylori stimulates the production of ROS and RNS by inflammatory cells and gastric epithelial cells. These agents bind with nucleic acids, resulting in generation of altered nucleotides. These modified nucleic acids could induce putative DNA damage, including single- or double-strand breaks, DNA intrastrand adducts, and DNA protein cross-links. In addition, ROS alters the mismatch repair function and allows mutations to accumulate in microsatellite sequences. One of the common products of free radical attack on DNA, 8-hydroxydeoxyguanine, is considered to be a biomarker of oxidative stress. The typical mutation pattern induced by this product represents C:G>A:T transversions.
Helicobacter pylori CagA also upregulates spermine oxidase (SMO) in gastric epithelial cells. SMO metabolizes the polyamine spermine into spermidine and generates H 2 O 2 . While H 2 O 2 causes apoptosis and DNA damage, a subpopulation of DNA damaged cells are resistant to apoptosis, resulting in increased risk for gastric cancer.
Chromosomal instability
Apart from nucleotide alterations, chromosomal aberrations are the other hallmark of gastric cancer. While it is considered that hypomethylation can induce chromosome breakage events, one notable thing is that CIN-type cancers frequently have TP53 mutations in many cancer types, including gastric cancer. This observation suggests that dysfunction of p53 may induce chromosomal aberration. The fact that TP53 mutation is present in chronic gastritis mucosa with H pylori infection indicates that early mutations in TP53 gene may be involved in CIN during gastric carcinogenesis. On the other hand, AID expression in gastric epithelial cells can cause chromosomal aberrations at various chromosomal loci as well as mutations. In human cases, the relative copy numbers of CDKN2A/CDKN2B are reduced in a subset of gastric cancer tissues compared with the surrounding noncancerous gastric mucosa. In addition, oral infection of wild-type mice with H pylori reduces the copy number of the Cdkn2b – Cdkn2a locus, whereas no such changes are observed in the gastric mucosa of H pylori -infected AID-deficient mice. These findings suggest that aberrant AID expression in gastric epithelial cells with H pylori infection induces chromosomal aberrations as well as mutations.
Mechanisms of Induction of Epigenetic Alterations
In cancer cells, the presence of regional hypermethylation and global hypomethylation has been shown. Regional hypermethylation refers to aberrant DNA methylation of specific promoter CpG islands physiologically kept unmethylated. Recent comprehensive genome analyses revealed that many tumor suppressor genes that have promoter CpG islands, such as MLH1 , MGMT , and CDKN2A , can be inactivated permanently in gastric cancer by aberrant DNA methylation as driver genes. Also, increasing DNA methylation of non-CpG islands is suggested to be involved in induction of mutation during gastric carcinogenesis. Interestingly, methylation levels of passenger genes in gastric mucosa with H pylori infection correlate with gastric cancer risk. This accumulation of aberrant DNA methylation in noncancerous tissues with chronic inflammation is considered to form an epigenetic field for cancerization. Along with the accumulation of genetic alterations, an epigenetic field defect is considered to be deeply related with gastric carcinogenesis. By contrast, gastric cancer as well as gastric mucosa with H pylori infection display global hypomethylation. Global hypomethylation has shown to be causally involved in carcinogenesis by inducing genomic instability.
The mechanisms by which H pylori infection induces DNA methylation remain unknown. Several mechanisms are proposed to explain aberrant promoter methylation in carcinogenesis, such as overexpression of DNA methyltransferases and reduction of DNA demethylation activity. Expression levels of several inflammatory-related genes, such as CXCL2, IL-1β, NOS2, and TNF-α, were seen to be parallel with methylation levels in gastric mucosa of Mongolian Gerbils with H pylori infection. Suppression of inflammation by the immunosuppressive drug cyclosporine A in H pylori -infected Mongolian Gerbils blocks induction of aberrant DNA methylation in gastric mucosa, although the number of H pylori was not changed. Furthermore, a polymorphism of the IL-1β promoter was associated with the presence of the CpG island methylation phenotype in gastric cancers. These findings indicate that chronic inflammation, rather than H pylori itself, is important for induction of aberrant DNA methylation. Interestingly, Niwa and colleagues revealed that a demethylating agent, 5-aza-2ʹ-deoxycytidine can decrease H pylori -induced gastric cancers in Mongolian Gerbils. Removal of induced DNA methylation and/or suppression of DNA methylation induction could become a target for prevention of chronic inflammation-associated cancers.
Mechanisms of Induction of microRNA Alterations
Micro RNAs (miRNAs) are short noncoding RNAs that can regulate the expression of many target genes posttranscriptionally and are related with various cellular functions. Gastric cancer cells have dysregulation of expression of many miRNAs involved in cell cycle progression, inhibition of apoptosis, cell invasion, and so on, thus contributing to carcinogenesis. The alteration of miRNA expression in epithelial cells can occur through various mechanisms such as NF-κB activation and cytokine stimulation following H pylori infection. For example, CagA has been shown to attenuate let-7 expression by histone and DNA methylation and then lead to the activation of the Ras pathway in gastric epithelial cells. Recently, epigenetic silencing of miR-210 has been shown to increase the proliferation of gastric epithelium during H pylori infection, contributing to gastric cancer development. However, mechanisms of how H pylori infection induce epigenetic changes in these miRNAs remain unknown.
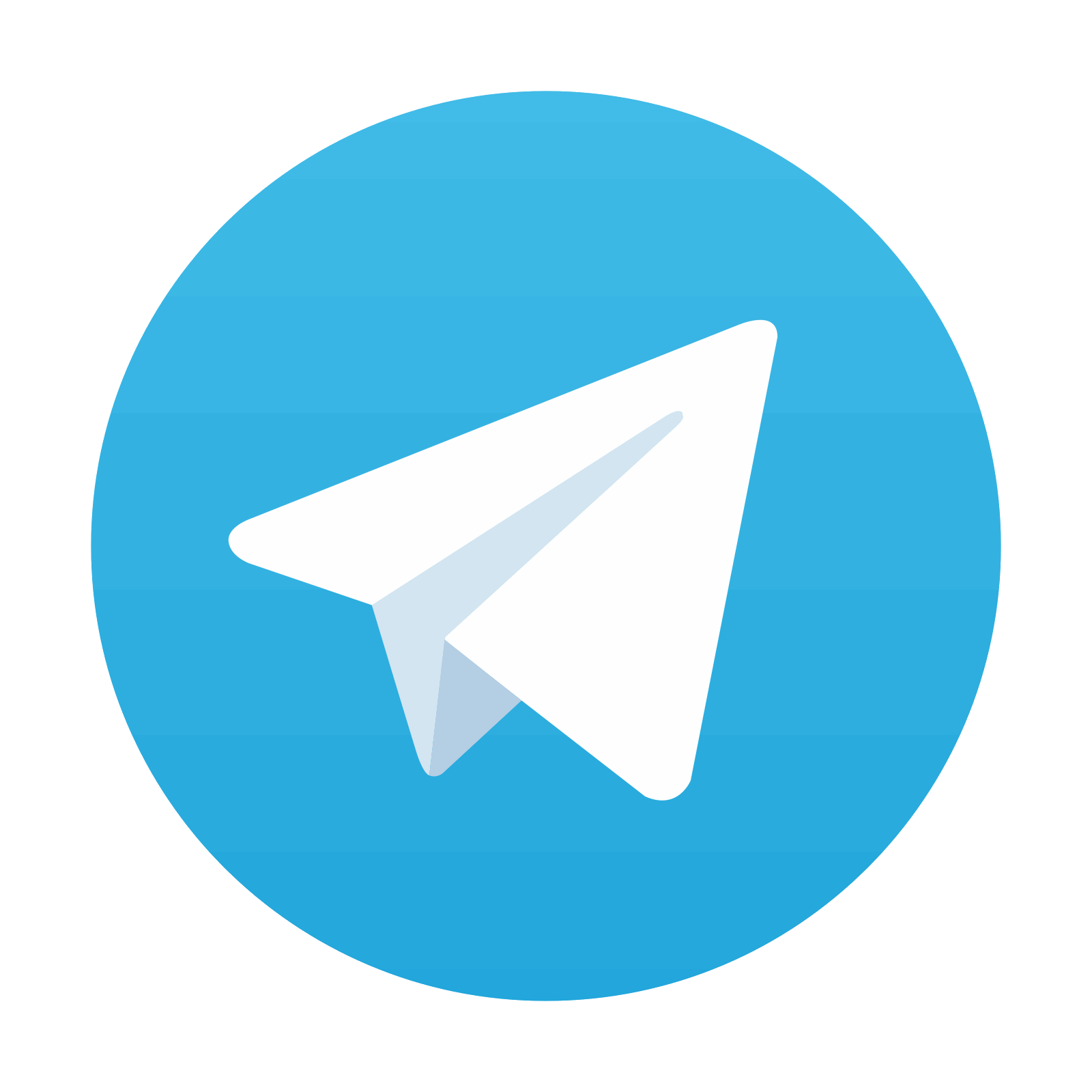
Stay updated, free articles. Join our Telegram channel

Full access? Get Clinical Tree
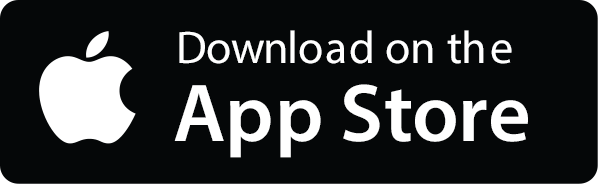
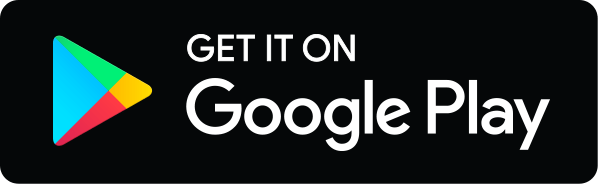