Somatostatin-receptor scintigraphy has become an obligatory molecular imaging method in the management of patients with neuroendocrine tumors when metastatic disease is suspected. Using positron emission tomography and new somatostatin analogues, sensitivity of somatostatin receptor imaging has further increased. With a combination of morphologic imaging methods, such as hybrid imaging by PET/CT, this method represents the method of choice in many centers and efforts are under way to translate somatostatin receptor imaging onto a cellular level by endoscopic confocal microscopy. Other clinically relevant functional pathways in neuroendocrine tumors that are accessible by PET imaging are glucose metabolism and amine precursor uptake and decarboxylation.
Neuroendocrine carcinomas (NECs) represent an inhomogeneous entity with growing incidence. They are classified by origin and by functional activity. Also, according to proliferation activity they are graded between highly differentiated (G1) and poorly differentiated (G3) with strong impact on prognosis and therapy. Whereas poorly differentiated NEC is a fast-growing malignancy with generally poor prognosis, highly differentiated neuroendocrine tumors (NETs) may be curable by operation. However, when classified as carcinoma, they always have the potential to metastasize and in an inoperable metastasized stage, cure is generally not achievable with current therapy modalities. Nevertheless, also metastasized, highly differentiated NECs display distinct tumor biology with consequences to the patients. On one hand, highly differentiated NECs are often exceptionally slow-growing solid tumors with courses of the disease of several years. On the other hand, in a significant fraction of patients, the excretion of neuroendocrine peptides leads to disabling symptoms, such as, among others, flushes and diarrhea. These neuroendocrine symptoms may additionally pose a therapeutic challenge in this patient population.
Increasing understanding of the molecular basis of NETs fostered many new targeted therapy approaches targeting, for example, G-protein–coupled receptors such as the somatostatin-receptor (SSTR), intracellular pathways, or angiogenesis. With regard to SSTR expression, highly differentiated NECs generally display characteristic high expression, whereas poorly differentiated NECs might display reduced expression of SSTR. Additionally, variable expression between different origins of NEC has been documented by immunohistological and imaging methods with, for example, on average higher SSTR expression for pancreatic NEC over ileum, hindgut, and pulmonary NEC.
Clinically, molecular imaging of neuroendocrine tumors is routinely performed with nuclear medicine methods taking advantage of highly specific radioactive tracers. These tracers are able to depict functional pathways and modern imaging acquisition devices deliver high resolution and ever more quantifiable whole body information. Depending on the decay properties of the respective radioisotopes, imaging is performed by either gamma camera imaging and single photon emission tomography (SPECT) or by positron emission tomography (PET). Both methods are increasingly parts of clinical management algorithms in NETs. Generally, PET imaging is associated with higher spatial resolution, shorter time interval between radiotracer application and image acquisition, and with simple and reliable quantification of tracer uptake ( Fig. 1 ). Additionally, hybrid imaging with PET in combination with computed tomography (CT) represents a comprehensive imaging method, increasingly applied in medical centers.
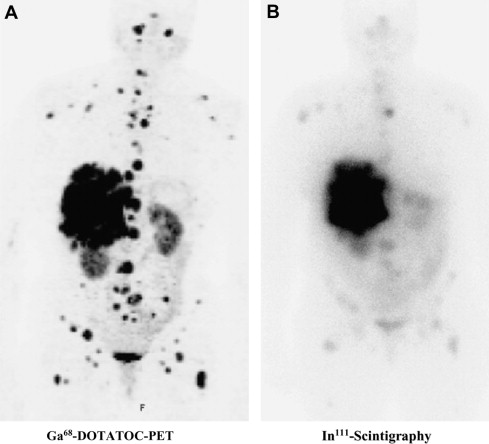
For conventional gamma imaging, the generator nuclide Technetium-99 m (half-life of 6 hours) or the longer-lived isotopes Indium-111 (half-life of 2.8 days) and Iodine-123 (half-life of 13.2 hours) are most commonly used. On the other hand, common positron emitting isotopes are the cyclotron-produced Fluor-18 (half-life of 110 minutes) and Carbon-11 (half-life of 20 minutes) and the generator-produced Gallium-68 (half-life of 68 minutes). With available generator systems, the short-lived isotopes are readily available and the appropriate radiotracers can reliably be produced on site. PET is able to acquire an established quantitative uptake parameter, namely standard uptake value (SUV). SUV is calculated by the absolute radioactivity concentration measured in a distinct volume corrected for patient weight and injected total activity.
Several distinct biological properties of NETs can readily be investigated by clinically available radiotracers. Most importantly, their usually high expression of somatostatin-receptor expression and the development of stable radiolabeled somatostatin analogues has yielded a highly sensitive and specific imaging method by means of receptor imaging. Several somatostatin analogues for PET and SPECT imaging and several suitable radioisotopes are available. Higher sensitivity for PET tracers in contrast to In-111-Octreotid (octreoscan) has been demonstrated.
Other tracers are able to display glucose metabolism ( 18 F-fluorodeoxyglucose), amine precursor uptake and decarboxylation ( 11 C-5-hydroxy-typtophan, 18 F-Flour-DOPA), and the noradrenaline transporter ( 131 I-meta-iodobenzylguanidine, 11 C-hydroxyephedrine). Other radiolabeled peptides have also been proposed for imaging and therapy, for example, among others, the gastrin-releasing peptide receptor, the glucagonlike peptide 1 receptor and neuropeptide-Y receptor.
Additionally, the route of application, eg, intra-arterially versus intravenously, might increase the uptake of radiotracers offering advantages both for diagnosis and therapy.
Selecting imaging methods with highest sensitivity, eg, for screening patients with multiple neuroendocrine neoplasms, with a high likelihood of developing a neuroendocrine tumor, or for sensitive follow-up after initial surgery, is challenging. A variety of emerging morphological methods are available. Highest resolution for detecting liver metastasis is often achieved by MRI or for pancreas tumors by endoscopic ultrasound. Depending on the biologic parameters of the tumors, functional imaging might give more accurate diagnostic results and generally display whole-body information. However, in many cases, functional and morphological imaging deliver complementary information. Thus, hybrid imaging such as PET/CT or PET/MRI will play an important role in the future.
Also, the choice of imaging modalities applicable for therapy monitoring depends on a variety of factors. Because of the slow-growing nature of differentiated NETs and because many therapies are associated with symptom control and disease stabilization, conventional radiologic criteria like RECIST (Response Evaluation Criteria in Solid Tumors) might not be sufficient and other parameters are needed.
Somatostatin receptor–based molecular imaging
Currently, 5 different SSTR subtypes have been identified, with SSTR-2 being the predominantly expressed one. Different somatostatin analogues with different affinity profiles over these receptors are currently in clinical use or in development. Furthermore, because of different chemical properties of radioisotopes, differences in affinity profiles may also arise between, for example, the diagnostic isotope Gallium-68 ( 68 Ga) and the therapeutic beta-emitting isotope Yttrium-90 ( Table 1 ). Somatostatin-receptor targeting is therapeutically used also with nonradioactive somatostatin analogues. To minimize competition for the receptor they should be withdrawn either 24 hours for short-acting somatostatin analogues or 4 to 8 weeks for long-acting somatostatin analogues before diagnostic procedures targeting the same receptor when clinically possible.
Affinity-Profiles of Somatostatin-Analogues (IC50-Values, nmol/L) | |||||
---|---|---|---|---|---|
Peptide | SSR1 | SSR2 | SSR3 | SSR4 | SSR5 |
Somatostatin-28 | 3,8 | 2,5 | 5,7 | 4,2 | 3,7 |
Gallium-68-DOTATOC | >10000 | 2,5 | 612 | >1000 | 73 |
Gallium-68-DOTATATE | >10000 | 0,2 | >1000 | 300 | 377 |
Gallium-68-DOTANOC | >10000 | 1,9 | 40 | 260 | 7,2 |
Indium-111-DTPA-Octreotide | >10000 | 22 | 182 | >1000 | 273 |
Somatostatin Receptor Scintigraphy
With Indium-111-DTPA-Octreotide (octreoscan), one of the first commercially available and broadly applied radiotracers in this indication, sensitivity close to 90% could be achieved more than a decade ago. Subsequently, somatostatin-receptor imaging has improved staging and follow-up of patients with neuroendocrine tumors and now has a key role in clinical management algorithms.
The main indications are detection and localization of gastroenteropancreatic tumors, pulmonary carcinoid tumors, adrenal medullary tumors, medullary thyroid carcinoma, paraganglioma, Merkel cell tumor, and meningioma. Sensitivities depend on tumor type and localization. For gastrionomas, VIPomas, and glucagonomas, the sensitivity was found to be between 75% and 100% and for insulinomas between 50% and 60%. Because of increasing advances in molecular imaging, mainly by 18 F-fluorodeoxyglucose-PET (FDG-PET) CT, other indications like melanoma, small-cell lung cancer, lymphoma, and others are secondary, unless somatostatin-targeted therapy is an option. Additionally, in preparation for peptide receptor radionuclide therapy somatostatin-receptor scintigraphy also plays an important role in defining radiotracer uptake and extent of the disease.
Physiological uptake by somatostatin-receptor binding occurs in adrenals, pituitary, thyroid, and spleen. Urinary excretion and to a much lesser extent hepatobiliary excretion is leading to visualization of liver, kidneys, bladder, and bowel.
The recommended administered activity is 185 to 222 MBq in adults and 5 MBq/kg in children. The amount of peptide injected is typically 10 to 20 μg. Image acquisition is performed at 4 and 24 hours and for more accurate imaging when significant bowl activity is present up to 48 hours. Besides anterior and posterior planar images of the whole body, SPECT imaging for appropriate regions should be included.
The development of Technetium-99 m–labeled somatostatin analogues improved image resolution and availability. However, in contrast to their Indium-111–labeled counterparts, delayed images of 24 hours and 48 hours are impossible, decreasing sensitivity for abdominal lesions. However, with SPECT and especially in combination with CT the sensitivity of gamma-emitting somatostatin analogues can be improved over planar imaging.
In a comparative study of MRI, CT, and somatostatin-receptor scintigraphy, sensitivity for the detection of liver metastasis was highest for MRI over CT over somatostatin-receptor scintigraphy, attributable to the high spatial resolution of MRI. However, somatostatin-receptor scintigraphy performed markedly better when metastasis reached sizes larger than 15 mm. Also, with high specificity of 88%, high availability, and the possibility of whole-body imaging, conventional somatostatin-receptor scintigraphy still plays the key role in diagnosis of metastasis from neuroendocrine tumors.
Positron Emission Tomography with Radiolabeled Somatostatin Analogues
Several Gallium-68–labeled tracers are applicable with differences in their affinity profile toward different somatostatin-receptor subtypes. However, these differences are clinically secondary and application depends on center-specific preferences.
Labeling of PET isotopes like Fluor-18 (T 1/2 = 110 minutes) or Gallium-68 (T 1/2 = 68 minutes) to somatostatin analogues has further advanced somatostatin imaging. It has been demonstrated that the higher spatial resolution leads to higher sensitivity on detection of differentiated neuroendocrine tumors ( Fig. 2 ). Furthermore, the uptake quantification with SUV is a parameter that correlates with somatostatin receptor expression. Although it has not been demonstrated that somatostatin imaging by PET can replace morphological imaging (CT/MRI/ultrasound) when therapeutic efficacy during therapy is assessed, the extent of somatostatin-receptor expression gives complementary information. This holds true especially in the situation when somatostatin-targeted approaches such as octreotide therapy or peptide receptor radio therapy is applied.
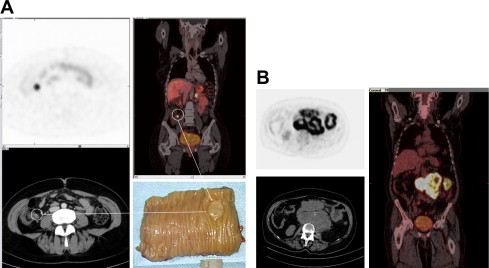
Recommended administered activity for Gallium-68-somatostatin analogues depends on imaging time, detector sensitivity, and time after injection. Typically, activities between 100 and 200 MBq are used with modern PET/CT scanners. Image acquisition is routinely performed 60 minutes after injection, although tracer accumulation is very rapid and some centers use earlier time points starting at 20 minutes postinjection.
Laser Confocal Endomicroscopy with Fluorescein-Labeled Somatostatin Analogues
Recently, laser confocal microscopy has been introduced in clinical routine diagnostics by integrating the miniaturized components of a confocal laser scanner into the tip of a conformité européenne (CE)-certified, Food and Drug Administration (FDA)-approved flexible endoscope. This novel technology allows subsurface histological diagnosis at the cellular and subcellular levels in vivo and provides instantaneous histopathology during ongoing upper and lower endoscopy. Endomicroscopy enables immediate diagnosis of neoplastic and inflammatory lesions of the intestinal mucosa. Previous studies have demonstrated the power of confocal endomicroscopy in screening and surveillance colonoscopy, ulcerative colitis, Barrett esophagus, and gastric cancer and morphodynamic analysis of liver disease in vivo. The integration of a similar miniaturized confocal laser scanner into a handheld device permits straightforward fluorescence confocal microscopy in vivo, allowing exact characterization of cell morphology and dynamic imaging of cellular events identifying various disease states like inflammation, neoplastic transformation, and imaging of microvasculature and perfusion. In studies by our own group, SSTRs were used as targets for in vivo real-time molecular imaging using a miniaturized laser confocal microscopy system. For selective visualization of SSTR, a novel contrast agent for laser confocal microscopy has been specifically developed by conjugating 5-carboxyfluorescein to octreotate. This fluorescein-labeled somatostatin analogue has been demonstrated to specifically bind to SSTR and exerts a functional antiproliferative effect on SSTR-positive tumor cells. After systemic application, it allows specific dynamic in vivo imaging of SSTR-positive neuroendocrine tumor cells as correlated with ex vivo immunohistochemistry ( Fig. 3 ). This not only provides instantaneous high-resolution histopathological tissue evaluation but additionally permits identification and characterization of subcellular molecular structures like somatostatin receptors. In general, the confocal mini-microscopy probe allows the immediate microscopic morphological, functional, and molecular evaluation of all easily accessible organs, thus facilitating screening for and early diagnosis of SSTR-positive neoplasias, because with routine endoscopic diagnostics, intestinal NET can usually not be distinguished from other polypoid structures by macroscopy alone.
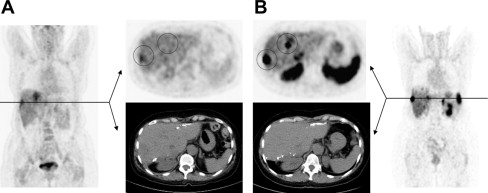
Other molecular targets for functional imaging of NETs
123 I-Metaiodobenzyl-Guanidine-Scintigraphy
Metaiodobenzyl-guanidine (MIBG) is a norepinephrine analogue that is concentrated in sympatho-adrenergic tissue. MIBG is actively transported through the noradrenalin transporter (NAT) into the cells and deposited in intracellular storage vesicles. MIBG is used as a diagnostic and therapeutic tracer when labeled with Iodine-123 and Iodine-131, respectively. MIBG uptake can be expected in 70% of patients with NEC, where better results are obtained for tumors originating from the pancreas compared with, for example, foregut origin. MIBG shows normally high uptake in liver, myocardium, salivary glands, intestines, spleen, bladder, and thyroid. The adrenal medulla is not routinely visualized. However, faint uptake might be detected. Typically, 370 MBq ( 123 I-MIBG) or 37 to 74 MBq ( 131 I-MIBG, when used for diagnostic purpose) of the radiopharmaceutical is administered by slow intravenous injection. Because of pharmacologic action, several side effects, such as tachycardia, hypertension, vomiting, and abdominal cramps, might occur when the administration of the tracer is too rapid. Several drugs, like antihypertensives and antidepressants might interfere with MIBG uptake either by inhibition of uptake or depletion of storage vesicles and therefore should be withdrawn before the study, whenever possible. Usually, interfering drugs should be discontinued up to 5 biological half-lives before the study. Additionally, thyroid blockade with potassium iodide or potassium perchlorate needs to be administered to decrease radiation exposure to the thyroid. Image acquisition is conducted at least 4 and 24 hours postinjection, preferentially including SPECT imaging.
6- 18 F-Fluoro-L-DOPA Positron Emission Tomography
NETs are also referred to as APUDomas because of their capacity for amine precursor uptake and decarboxylation. Thus, amine precursors, such as L-dihydroxyphenylalanine (L-DOPA), may be taken up into the tumor cells where they are decarboxylated by the action of the enzyme aromatic amino acid decarboxylase (AADC), which is strongly expressed in neuroendocrine cells and shows high affinity to 18 F-DOPA. This reaction converts the precursor into their corresponding amine, dopamine. These are stored in intracellular vesicles where it can be visualized for imaging purposes. Based on the APUD concept, 18 F-labeled L-DOPA ( 18 F-L-DOPA), originally used to study patients with movement disorders, has been used in a number of studies to visualize various different types of neuroendocrine tumors, such as medullary thyroid cancer, pheochromocytomas, and paragangliomas, as well as gastro-entero pancreatic (GEP)-NET. Recently, the catecholamine precursor fluorine-18-dihydroxyphenylalanine ( 18 F-FDOPA) has mainly been used as a PET-tracer for imaging of pheochromocytomas and paragangliomas. The advantages of this technique are a quick performance within 2 hours, superior spatial resolution, no interference with medications, and no relevant physiological tracer uptake in normal adrenal medulla. Several smaller studies showed an excellent sensitivity equivalent or superior to the routinely used 123 I-MIBG scintigraphy in patients with known or suspected benign and malignant pheochromocytomas and paragangliomas. A recent study of our own group demonstrated that FDOPA-PET is clearly superior to 123 I-MIBG scintigraphy in patients with extra-adrenal, predominantly noradrenaline-secreting and hereditary types of pheochromocytomas or paragangliomas. Immunohistochemical analysis of vesicular monoamine transporters (VMAT) type 1 and 2, which are responsible for the specific transport and storage into vesicles, demonstrated a lack of VMAT-1 expression in all MIBG-negative tumors. The lack of VMAT-1 expression predicted negativity for MIBG scintigraphy but not for DOPA-PET, which was positive in all cases ( Fig. 4 ). For functional imaging of GEP-NET, DOPA-PET has been used in a number of studies, mainly to evaluate an alternative imaging modality for functioning tumors that are negative on somatostatin-receptor imaging. An initial study by Hoegerle and colleagues reported that DOPA-PET allowed the detection of a higher number of NET lesions in the gastrointestinal tract as compared with SSTR imaging and FDG-PET, respectively. In another study with 22 patients with morphological (CT) and/or biochemical diagnosis of endocrine pancreatic tumors, DOPA-PET allowed the visualization of 50% of the tumors but failed to detect nonfunctioning tumors and small insulinomas. In this study, in 7 patients with biochemical evidence of pancreatic NET, both DOPA-PET and CT failed to detect the tumor. In a recent prospective single-center study by Koopmans and colleagues of 53 patients with metastatic carcinoids, both the region-based and lesion-based sensitivity was higher for DOPA-PET than for SSTR scintigraphy and for the combination of SSTR scintigraphy and CT. In pancreatic endocrine tumors, the sensitivity of DOPA-PET was slightly lower.
