Test
Change from nonpregnant state
Bilirubin (total)
Unchanged (or slightly decreased)
Aminotransferases (AST, ALT)
Unchanged
Alkaline phosphatase
Increases 2–4 fold
Albumin
Decreases
Prothrombin time
Unchanged
Fibrinogen
Increases by 50 %
Globulin
Increases in α and β globulins, decreases in γ globulin
Alpha-fetoprotein
Increases
White blood cell count
Increases
Platelets
Unchanged
Ceruloplasmin
Increases
Cholesterol
Increases twofold
Triglycerides
Increases
Hemoglobin
Decreases (from second trimester)
Cell volume
Decreases
Uric acid
Decreases
Plasma volume increases by approximately 50 % during pregnancy; however, the blood flow to the liver remains constant. In addition to a rise in maternal heart rate and cardiac output, there is also a decrease in blood pressure and systemic vascular resistance (SVR) . These changes may mimic the physiologic changes seen in patients with decompensated chronic liver disease. Spider angiomata and palmar erythema, which are also seen in those with chronic liver disease, are the result of increased serum estrogen levels and are normal findings in pregnancy and usually resolve after delivery.
During a normal pregnancy, alkaline phosphatase levels are elevated due to increased placental isoenzyme activity and serum albumin concentrations fall due to the expansion in plasma volume [2–4]. Serum aminotransferase concentrations (aspartate aminotransferase [AST] and alanine aminotransferase [ALT]) , total bilirubin, and γ-glutamyl transpeptidase (GGT) remain normal throughout pregnancy or are marginally reduced due to hemodilution [2, 3]. Therefore, any elevations of AST, ALT, and serum bilirubin are considered pathologic and require further investigation.
Ultrasonography remains the safest modality to visualize the liver during pregnancy. If additional detailed imaging is needed, MRI without contrast is safe during pregnancy with no harmful effects towards the developing fetus [5, 6]. However, gadolinium-enhanced MRI should be avoided due to the transplacental transfer of gadolinium, whose effects on the fetus are largely unknown [7].
Classification of Liver Diseases in Pregnancy
Liver diseases in pregnancy are broadly categorized as diseases that are unique to pregnancy and those that are not (Table 26.2). Most liver dysfunction that occurs during pregnancy is directly related to the pregnant state and is due to one of five liver diseases unique to pregnancy—hyperemesis gravidarum (HG), intrahepatic cholestasis of pregnancy (ICP), preeclampsia, HELLP syndrome, and acute fatty liver of pregnancy (AFLP). Liver diseases that are not related to pregnancy are further categorized upon the presence or absence of preexisting liver disease (Table 26.2).
Table 26.2
Classification of liver disease in pregnancy
Diseases unique to pregnancy | Diseases not unique to pregnancy | |
---|---|---|
Preexisitng liver diseases | Liver disease coincident with pregnancy | |
Hyperemesis gravidarum (HG) | Chronic hepatitis B and C | Viral hepatitis |
Intrahepatic cholestasis of pregnancy (ICP) | Cirrhosis and portal hypertension | Biliary disease |
Acute fatty liver of pregnancy (AFLP) | Autoimmune liver disease | Budd-Chiari syndrome |
Preeclampsia and eclampsia | Wilson disease | Drug-induced liver injury (DILI) |
HELLP syndrome | Liver transplantation |
Liver Diseases Unique to Pregnancy
Gestational age at the time of onset of signs and symptoms is critical in determining the etiology of liver disease as each condition has a characteristic timing of onset (Table 26.3).
Table 26.3
Characteristic timing of liver diseases unique to pregnancy
Liver disease | Trimester |
---|---|
Hyperemesis gravidarum (HG) | 1, 2 |
Intrahepatic cholestasis of pregnancy (ICP) | 1a, 2, 3 |
Pre-eclampsia | 2, 3 |
HEELP | 2, 3 |
Acute fatty liver of pregnancy (AFLP) | 2, 3 |
Hyperemesis Gravidarum
Definition and Diagnosis
Nausea and vomiting of pregnancy (NVP) , which is often referred to as “morning sickness,” is common and affects nearly 80 % of pregnant women [8, 9]. Hyperemesis gravidarum (HG), which represents a severe end of the spectrum of NVP, occurs in only 0.3–2.0 % of all pregnancies [10, 11]. The diagnosis of HG is clinical and is supported by intractable vomiting resulting in dehydration, electrolyte imbalance, muscle wasting, ketosis, and weight loss of more than 5 % body weight. Symptoms related to HG may manifest as early as week 4 of pregnancy and typically subside by 18–20 weeks of gestation [12].
Etiology
The exact cause of HG remains unclear; however, immunological, hormonal, and psychological factors likely play a role. Risk factors for HG include elevated body-mass index (BMI), hyperthyroidism, underlying psychiatric illness, molar pregnancy, preexisting diabetes mellitus, and multiple pregnancies [13, 14].
Serum aminotransferases are elevated in nearly 50 % of patients diagnosed with HG, the levels of which may rise to more than 20 times the upper limit of normal [14, 15]. Hyperbilirubinemia is uncommon in HG but if present rarely exceeds 4 mg/dL. The degree of elevation in liver biochemical tests correlates with the severity of symptoms. The elevated liver tests quickly normalize upon resolution of vomiting.
Pathology
Given the typical clinical manifestations of HG, a diagnostic liver biopsy is seldom needed. If performed, majority of liver biopsies are normal or with nonspecific findings demonstrating cholestasis with mild centrilobular necrosis [16].
Treatment
The first-line approach to mild presentations of HG typically includes avoidance of environmental triggers with bowel rest followed by reintroduction of small and frequent meals and anti-histamines. Thiamine (vitamin B1) supplement is recommended to prevent Wernicke encephalopathy, especially in women with a protracted course of emesis. Hospitalization is often necessary for more severe presentations and may require intravenous rehydration, nutritional support, and antiemetic therapy. Treatment of HG using intravenous corticosteroids remains controversial and typically reserved for severe refractory cases.
Outcomes and Recurrence
Infants of mothers with HG may be born prematurely, be small for gestational age and have significantly lower birth weights than infants born to mothers without HG [17, 18]. There are no clear associations with HG and congenital anomalies or perinatal death. If untreated, there are reports of consequences related to micronutritent deficiency, namely Wernicke encephalopathy and malnutrition, namely poor wound healing, muscle wasting, and immunosuppression [19, 20].
HG often recurs in subsequent pregnancies. There is a 15 % increased risk of HG in the second pregnancy in women with a history of HG compared to only 0.7 % increased risk in women without previous HG [21].
Intrahepatic Cholestasis of Pregnancy
Introduction and Epidemiology
Intrahepatic cholestasis of pregnancy (ICP) is characterized by pruritus and an elevation in serum bile acid concentrations. While ICP typically occurs during the second or third trimester, rare cases developing as early at 6–10 weeks have been reported [22–24]. The prevalence of ICP has significant geographic variation. The highest prevalence rates of ICP were previously reported in Bolivia and Chile with a prevalence from 11.8 to 27.6 % [25, 26]. The prevalence in the US is estimated at 0.3–5.6 % [27, 28].
Pathogenesis
The exact cause of ICP is largely unknown but recent epidemiologic and clinical studies suggest that hormonal, genetic, and environmental factors are likely involved [29].
Estrogen and progesterone directly inhibit the bile salt export pump and induce cholestasis. Serum concentrations of estrogen during pregnancy peak in the third trimester, which correlate with the onset of ICP. Genetic variants in genes encoding for several hepatobiliary transporters were discovered in patients with ICP that likely explain familial cases and the higher incidence in certain ethnic groups. The multidrug resistance 3 (MDR3) protein, which serves as the transporter for phosphotidylcholine across the canalicular membrane into bile, is associated with ICP. Specifically several mutations in the ABCB4 gene that encodes MDR3 have been identified in patients with ICP. Mutations in the ABCB4 gene leads to loss of function of MDR3, which results in elevated serum bile acids without phospholipids leading to injury of the canalicular membrane and cholestasis [30, 31]. The incidence of ABCB4 gene mutations in Caucasian patients diagnosed with ICP is estimated at 16 % [32].
Clinical Manifestations and Laboratory Findings
The onset of ICP is typically preceded by the development of pruritus. Pruritus predominates on the palms and soles of the feet, but may be generalized and become intolerable. Pruritus is typically worse at night and often disrupts sleep. Pruritus usually resolves within a few days after delivery. Jaundice, which typically presents after the onset of pruritus, develops in less than 20 % of patients [33]. Women with ICP may develop diarrhea and steatorrhea due to severe cholestasis requiring fat soluble vitamin supplementation.
The laboratory hallmark of ICP is elevated serum total bile acid concentrations, which may be the first or only laboratory abnormality [34]. Total and direct bilirubin along with 5′ nucleotidase may also be elevated. Total bilirubin levels rarely exceed 10 mg/dL. Alkaline phosphatase is also elevated, but is not specific for cholestasis during pregnancy due to expression of placental alkaline phosphatase isoenzyme. On the other hand, serum levels of gamma glutamyl transpeptidase (GGT) are often normal or only slightly elevated in ICP, which is atypical in cholestatic liver disease as GGT levels typically parallel other cholestatic markers. Elevated GGT levels may indicate MDR3 mutation or underlying liver disease unrelated to pregnancy. Serum aminotransferase levels may at times exceed 1000 U/L, which should also prompt distinction from concurrent viral hepatitis. The prothrombin time (PT) is usually normal, but it may become prolonged due to vitamin K deficiency in the setting of severe cholestasis with jaundice or recent use of bile acid sequestrants, namely cholestyramine, rather than intrinsic liver dysfunction. For this reason, vitamin K should be administered prior to delivery to prevent postpartum hemorrhage.
Diagnosis
The diagnosis of ICP is based upon the presence of pruritus with elevated total serum bile acid concentrations and/or aminotransferases in the absence of diseases that may produce similar clinical and laboratory findings. A fasting serum bile acid concentration >10 μmol/L is considered diagnostic [35].
Pathology
Given the characteristic clinical and laboratory findings in ICP, liver biopsy is rarely needed to confirm the diagnosis. When performed, histopathology is characterized by cholestasis in the absence of inflammation or necrosis. The portal tracts are unaffected [36].
Medical Management
Ursodeoxycholic acid (ursodiol) is the first-line treatment of ICP and should be given from time of diagnosis until delivery. Ursodiol (10–15 mg/kg/day) provides relief from pruritus, improves liver biochemical tests and is well tolerated by mother and fetus [37]. As in other chronic cholestatic liver diseases, ursodiol, which is a hydrophilic bile acid, may provide some degree of cytoprotection against the hepatotoxic effects of the hydrophobic bile acids. In addition, ursodiol increases bile flow, decreases plasma bile acid and sulphated progesterone metabolite concentrations, increases bile sale export pump expression, and restores proper transport of bile across the placenta, which is impaired in ICP [38, 39].
There are no clear guidelines regarding the optimal time for delivery in patients with ICP [40]. In severe cases of ICP, current consensus opinion supports the practice of induction of labor between 36 and 38 weeks gestation to allow for fetal lung maturity and minimize incidence of intrauterine fetal demise (IUFD) that is associated with ICP [40].
Maternal and Fetal Outcomes
Overall, the maternal prognosis in ICP is good. Pruritus typically disappears within a few days after delivery and is accompanied by normalization of total serum bile acid concentrations and other liver biochemical markers. Recurrent cholestasis occurs in up to 70 % of subsequent pregnancies with variable degrees of severity. In two recent longitudinal population-based cohort studies from Sweden and Finland, women with a history of ICP had a significantly increased risk for developing hepatobiliary disease, namely hepatitis C virus (HCV), fibrosis, cirrhosis, gallstones disease, cholangitis, nonalcoholic pancreatitis, and nonalcoholic liver cirrhosis including cases of PBC cirrhosis [41, 42].
In contrast to a more favorable maternal prognosis, ICP carries significant fetal risk. Fetal complications correlate with serum bile acid concentrations, with a negligible risk if levels remain <40 μmol/L [35, 43]. The main complications of ICP include prematurity and IUFD , which has an estimated incidence between 1 and 2 % [44, 45].
Preeclampsia and HELLP Syndrome
Definition and Epidemiology
Preeclampsia is a common complication of pregnancy with an incidence of 2–7 % in healthy nulliparous women [46]. It is characterized by new onset hypertension and either proteinuria or end-organ dysfunction after 20 weeks of gestation [47]. Preeclampsia itself typically does not have liver involvement; however, if present signifies severe disease. Multiple risk factors for the development of preeclampsia include a personal or family history of preeclampsia, presence of antiphospholipid antibodies, preexisting diabetes mellitus, pre-pregnancy body mass index (BMI) >35 kg/m2, nulliparity, a twin pregnancy, and maternal age ≥ 40 years [48].
HELLP syndrome represents a severe form of preeclampsia and occurs in up to 0.8 % of all pregnancies and 10–20 % of women with severe preeclampsia. HELLP syndrome is characterized by hemolysis (H), elevated liver enzymes (EL), and low platelets (LP). In contrast to preeclampsia, nulliparity is not a risk factor for HELLP syndrome as at least 50 % of patients with HELLP syndrome are multiparous [49].
Pathogenesis
The exact pathophysiology of HELLP syndrome is unknown, but proposed mechanisms include aberrant placental development and function with subsequent vascular remodeling and uteroplacental ischemia. The ensuing thrombotic microangiopathy leads to microangiopathic hemolytic anemia and liver damage.
Clinical Presentation
The clinical presentation of HELLP syndrome varies. The most common symptom is epigastric or right upper quadrant abdominal pain, which is often associated with nausea, vomiting, and malaise. Mistaking these nonspecific symptoms for a viral illness is an unfortunate error that may result in significant maternal morbidity or mortality because severe right upper quadrant pain may suggest imminent hepatic rupture [50].
The majority of cases present between 28 and 36 weeks of gestation. However, postpartum HELLP may occur in up to 30 % of cases, most of which present within 48 h of delivery with some as far out as 7 days after delivery [51].
Diagnosis
The diagnosis of HELLP syndrome relies on the abnormalities comprising its name—hemolysis (microangiopathic hemolytic anemia with schistocytes), elevated liver enzymes, and low platelet count. Elevated serum aminotransferases are seen in up to 10 % of pregnant women with severe preeclampsia, but the frequency and severity of which is much higher in HELLP syndrome than in severe preeclampsia [52]. Serum aminotransferases in HELLP are often >500 U/L. Classification systems for the diagnosis of HELLP have been developed and include the Tennessee [49, 53] and Mississippi [52] classifications (Table 26.4).
Table 26.4
Diagnostic criteria for HELLP syndrome
Tennessee classification | Mississippi classifications |
---|---|
Complete syndrome | Class 1: platelets ≤50 × 109/L |
Platelets ≤100 × 109/L | 1. AST or ALT ≥70 U/L |
AST ≥ 70 U/L | 2. LDH ≥ 600 U/L |
LDH ≥ 600 U/L | Class 2: platelets ≤100 × 109/L |
Incomplete syndrome | 1. >50 × 109/L |
Any 1 or 2 of the above | 2. AST or ALT ≥70 U/L |
3. LDH ≥ 600 U/L | |
Class 3: platelets ≤150 × 109/L | |
1. ≥100 × 109/L | |
2. AST or ALT ≥40 U/L | |
3. LDH ≥ 600 U/L |
The differential diagnosis for a clinical presentation similar to that of HELLP syndrome includes acute viral hepatitis, hemolytic uremic syndrome (HUS), thrombotic thrombocytopenic purpura (TTP), antiphospholipid syndrome, acute appendicitis, gallbladder disease, lupus flare, and AFLP.
Pathology
Liver biopsy remains a high-risk procedure due to thrombocytopenia. When performed, biopsy demonstrates findings typical of preeclamptic livers, which include periportal hemorrhage and fibrin deposition. Moderate amount of lobular macrovesicular steatosis may be present, which is in contrast to the centrizonal microvesicular steatosis that is typical of AFLP [54].
Management
Bed rest and control of hypertension is imperative for all patients with preeclampsia/HELLP. In addition, all women with severe preeclampsia/HELLP should receive intravenous magnesium sulfate to prevent cerebral changes and eclamptic seizures. Urgent delivery is indicated if HELLP syndrome develops after 34 weeks of gestation, or earlier in the setting of multiorgan dysfunction, renal failure, DIC, liver infarction or hemorrhage, suspected abruptio placentae, or fetal distress. Although corticosteroids have been shown to significantly improve platelet counts, their use did not reduce the risk of severe maternal morbidity, maternal death, or perinatal/infant death [55]. Plasma exchange therapy has been used successfully in patients with severe HELLP syndrome with organ failure or refractory disease resulting in significant improvement in abnormal laboratory values, shorter ICU stay, and improved mortality rates [56, 57].
Outcomes and Prognosis
A variety of maternal and fetal morbidities are associated with HELLP syndrome. The risk of serious morbidity correlates with the severity of maternal symptoms and laboratory abnormalities [58]. Maternal signs and symptoms typically improve within 48 h of delivery; however, a protracted course may ensue. Maternal complications include retinal detachment, DIC, abruptio placentae, acute kidney injury, pulmonary edema, subcapsular liver hematoma, and hepatic rupture [51]. Various surgical and interventional radiologic procedures have been described for the management of hepatic rupture, namely hepatic artery ligation, hepatic packing or lobectomy, arterial embolization, and liver transplantation.
Acute Fatty Liver of Pregnancy
Definition and Epidemiology
Acute fatty liver of pregnancy (AFLP) is a rare but potentially fatal complication that occurs in the third trimester. AFLP is rare with an estimated incidence of 1 in 7000 to 1 in 20,000 pregnancies [63, 64]. It is characterized by microvesicular fatty infiltration of hepatocytes. It was first described in 1934 as “acute yellow atrophy of the liver” and at the time was thought to be universally fatal [65]. Fortunately, early diagnosis and prompt delivery have dramatically improved the prognosis [66].
Pathogenesis
There is a strong association between AFLP and fetal deficiency of mitochondrial long-chain 3-hydroxyacyl CoA dehydrogenase (LCHAD), which is an inherited defect in mitochondrial β-oxidation of fatty acids [67]. LCHAD is part of the mitochondrial trifunctional protein (MTP) , which is a complex mitochondrial enzyme alpha subunit. Several mutations have been observed in the gene coding for LCHAD in families with a child with LCHAD deficiency whose mothers developed AFLD [67]. G1528C is the most common mutation associated with LCHAD deficiency. Fetal LCHAD deficiency results in accumulation of hepatotoxic fetal fatty acids to which the mother is exposed via maternal–fetal circulation. These hepatotoxic metabolites deposit in the maternal liver and cause liver injury. LCHAD-deficient fetuses were found in approximately 20 % of women who developed AFLP [68]. On the other hand, an LCHAD-deficient fetus was associated with a 79 % chance of developing either ALFP or HELLP [67]. Most LCHAD-deficient newborns present with a metabolic crisis within the first year of life while others may suffer a sudden and unexpected death as early as a few months of age [69]. Therefore, screening the offspring of women who develop AFLP as early as possible after delivery can be lifesaving [69, 70]. Identifying LCHAD-deficient newborns early is particularly important as treatment with dietary modifications can dramatically reduce morbidity and mortality.
Clinical and Laboratory Findings
AFLP typically occurs in the third trimester. The initial manifestations of AFLP are nonspecific and often include nausea or vomiting, epigastric or right upper quadrant pain, headache, fatigue, anorexia, and jaundice. Hypertension, edema, and ascites may also be present as nearly 50 % of patients with AFLP have associated preeclampsia [71]. Serum aminotransferase levels are elevated with levels typically ≤500 IU/mL. The bilirubin level is almost always elevated. In severe cases, progression may be rapid within hours to days with progression to hepatic failure, renal failure, and severe coagulopathy with hemorrhage leading to death of the mother and fetus [72].
Diagnosis
The diagnosis of AFLP is generally a clinical diagnosis based on the presentation in relation to gestational age and compatible laboratory and radiographic findings. However, there is often a large clinical overlap between AFLP and HELLP that may make it difficult to differentiate between the two. Evidence of hepatic dysfunction such as encephalopathy or hypoglycemia in the setting of coagulopathy is more indicative of AFLP.
Liver biopsy is diagnostic for AFLP showing characteristic microvesicular hepatic steatosis [73]. The fat droplets are small and surround centrally located nuclei, which give the cytoplasm a distinctive foamy appearance. The vacuolated hepatocytes stain red with use of an oil red O stain. These histologic changes disappear within days to weeks after delivery without persistent injury. The Swansea diagnostic criteria have been proposed as an alternative to liver biopsy for the diagnosis of AFLP (Table 26.5) [1].
Table 26.5
Swansea criteria for diagnosis of AFL
Six or more of the following features in the absence of another explanation | |
---|---|
Vomiting | Leukocytosis (>11 × 106/L) |
Abdominal pain | Ascites or bright liver on ultrasound |
Polydipsia/polyuria | Transaminemia (AST or ALT >42 IU/mL) |
Encephalopathy | Hyperammonia (>47 μmol/L) |
Hyperbilirubinemia (>14 μmol/L) | Renal insufficiency (serum Cr >150 μmol/L) |
Hypoglycemia | Coagulopathy (PT > 14 or APTT > 34) |
Hyperuricemia (>340 μmol/L) | Microvesicular steatosis on liver biopsy |
Management
AFLP does not typically resolve before delivery and is considered an obstetric emergency. If delivery is delayed catastrophic complications may develop, namely hemorrhage or intrauterine death. Therefore, treatment includes a combination of maternal stabilization and prompt delivery of the fetus, regardless of gestational age.
Maternal and Fetal Outcome
Liver biochemical and coagulation profiles usually normalize within 7–10 days after delivery [74]. Although most patients recover with no significant clinical sequelae, substantial morbidity and mortality can occur [74]. The maternal mortality rates for AFLP are estimated at 1.8–4.0 % with stillbirth rates as high as 12 % [64, 74]. Some patients with severe AFLP that persisted after delivery were successfully treated with liver transplantation [64, 75, 76] including a case report of a successful auxiliary liver transplant [77]. AFLP can recur in subsequent pregnancies even if the workup for the LCHAD mutation is negative; however, the exact risk in unknown.
Vascular Disorders
- 1.
Do all patients diagnosed with portal vein thrombosis need anticoagulation? If so, for how long?
Anticoagulation for portal vein thrombosis (PVT) depends largely on the time interval since the blood clot was formed because the indication and duration of anticoagulation is different for acute PVT compared to chronic PVT. The goal of treatment of acute PVT is to recanalize or reopen the obstructed veins, which will prevent congestion of venous blood in the intestines that could cause intestinal infarction and portal hypertension. Therefore, anticoagulation should be given for at least 3 months to all patients with acute PVT to allow for recanalization of the obstructed veins or longer in causes when acute PVT is associated with permanent thrombotic risk factors that are not otherwise correctable. On the other hand, the goal of treatment of chronic PVT with anticoagulation is to prevent recurrentthrombosis. Long-term anticoagulation is generally given to all patients with chronic PVT without cirrhosis that have an identifiable, uncorrected risk factor for venous thrombosis as long as there is no major contraindication.
Portal Vein Thrombosis
Portal vein thrombosis (PVT) refers to obstruction of the portal vein (PV) or its branches due to thrombosis or to invasion or constriction by a malignant tumor. The prevalence of PVT in population based autopsy studies was nearly 1 % [78, 79]. From a clinical prospective, PVT is subdivided into acute and chronic. Although acute and chronic PVT represent successive stages of the same disease and share similar causes, their respective management is different. In addition to the stage at which the PVT is recognized, management is also based on the presence or absence of cirrhosis (Portal vein thrombosis in cirrhosis is discussed separately in Chap. 10).
Causes of Portal Vein Thrombosis
Numerous local and systemic risk factors are associated with the development of PVT (Table 26.6). Malignant tumor invasion of the PV and cirrhosis are the most common local risk factors for PVT [80–82]. An inherited or acquired prothrombotic condition is a common risk factor for the development of PVT, regardless of the presence of a local risk factor (Table 26.7) [81, 83–96]. In young adults without malignancy or cirrhosis, PVT is commonly the presenting manifestation of an underlying myeloproliferative disease, namely polycythemia vera or essential thrombocytosis [90, 97].
Table 26.6
Local risk factors for portal vein thrombosis
Malignancy | Injury to the portal venous system |
Any abdominal organ | Splenectomy |
Cirrhosis | Colectomy, Gastrectomy |
Focal inflammatory lesions | Cholecystectomy |
Neonatal | Liver transplantation |
Neonatal omphalitis | Abdominal trauma |
Umbilical vein catheterization | Surgical portosystemic shunting, TIPS |
Diverticulitis, Appendicitis | |
Pancreatitis | |
Duodenal ulcer | |
Cholecystitis | |
Tuberculous lymphadenitis | |
Inflammatory bowel disease | |
Cytomegalovirus hepatitis |
Table 26.7
Prevalence of acquired and inherited risk factors for acute PVT and BCS
Risk factor | Acute PVT (%) | BCS (%) |
---|---|---|
Myeloproliferative disorders | 21–40 | 40–50 |
Antiphospholipid antibody syndrome | 6–19 | 4–25 |
Parosysmal nocturnal hemoglobinuria | 0–2 | 0–19 |
Factor V Leiden mutation | 3–32 | 6–32 |
Prothrombin gene (Factor II) mutation | 14–40 | 3–7 |
Protein C deficiency | 0–26 | 4–30 |
Protein S deficiency | 2–30 | 3–20 |
Antithrombin III deficiency | 0–26 | 0–23 |
Hyperhomocysteinemia | 11–22 | 22–37 |
Recent pregnancy | 1–40 | 6–12 |
Recent contraceptive use | 12–44 | 6–60 |
Systemic diseasea | 4 | 23 |
>1 risk factor | 52 | 46 |
Local factor (Table 26.6) | 21 | 6 |
Acute Portal Vein Thrombosis
Acute PVT refers to the sudden formation of a thrombus within the main PV and/or its branches or involves a variable portion of the mesenteric veins and/or splenic vein. Occlusion can be partial or complete.
Clinical and Laboratory Features
The presentation of acute PVT usually includes acute onset abdominal pain. Rebound tenderness and guarding may be present in the setting of an inflammatory focus as cause for the PVT or when PVT is complicated by intestinal infarction. Although partial thrombosis is often associated with fewer symptoms, rapid and complete obstruction of the portal or mesenteric veins may cause intestinal congestion resulting in severe continuous colicky abdominal pain and diarrhea. Although a transient, moderate increase in serum aminotransferases may be seen in patients with acute PVT, liver function is preserved on account of increased hepatic arterial blood flow that compensates for the decreased portal venous inflow as well as collateral circulation that quickly develops from preexisting veins in the porta hepatis [98].
In the absence of thrombus extension to mesenteric venous arches, all manifestations of acute PVT are reversible by way of recanalization or development of a cavernoma [99]. Intestinal infarction is the most dreaded complication of acute PVT and has been reported in 2–28 % of cases [99–102]. Intestinal infarction occurs after thrombosis extends to the mesenteric veins causing intestinal congestion, ischemia, and eventually infarction. Clinical features suggestive of transmural intestinal ischemia include persistent severe abdominal pain lasting more than 5–7 days, bloody diarrhea, ascites, or multiorgan failure with metabolic acidosis [103, 104]. Intestinal infarction carries a mortality rate of 20–60 % with significant morbidity due to extensive intestinal resection or post-ischemic intestinal stenosis [105–109].
A thrombus may become infected resulting in acute septic PVT, which is referred to as acute pylephlebitis [110]. Blood cultures often grow Bacteroides species with radiographic evidence of multiple, small liver abscesses. Acute septic PVT is almost always associated with an abdominal infection [99, 110, 111].
Radiographic Features and Diagnosis
Sonographic findings of acute PVT include hyperechoic material in the vessel lumen with distension of the PV and its tributaries with Doppler imaging demonstrating absent flow in part or all of the lumen [112]. A CT or MRI can provide additional information regarding the extent of the thrombus, dating of the thrombus, presence of a local factor, or intestinal congestion and/or ischemia [113]. Thinning of the intestinal wall or lack of mucosal enhancement of a thickened intestinal wall after intravenous contrast is suggestive of intestinal infarction [114].
Treatment
The goal of treatment of acute PVT is to allow recanalization of the obstructed veins and prevent portal hypertension and intestinal infarction. Immediate initiation of systemic anticoagulation therapy is generally recommended for acute PVT [109, 115]. Although anticoagulation therapy is of proven benefit in patients with acute DVT [116], there are no controlled studies of anticoagulation therapy in patients with acute PVT. As such, DVT data are extrapolated to patients with acute PVT. However, pooled data from retrospective surveys showed that when started immediately, 6 months of anticoagulation resulted in complete recanalization in 50 %, partial recanalization in 40 %, and no recanalization in 10 % of patients [99]. In the case of pylephlebitis, antibiotics alone may result in recanalization [111, 117].
The optimal duration of anticoagulation for acute PVT is unknown. An international panel of experts recommend that in patients with acute PVT, anticoagulation be given for at least 3 months, while indefinite anticoagulation be given for those with chronic prothrombotic conditions [99]. The published data on other treatment modalities (transjugular intrahepatic portosystemic shunt [TIPS], thrombolysis, or surgical thrombectomy) in the management of acute PVT is limited [118, 119].
Chronic Portal Vein Thrombus
In patients with chronic PVT, the obstructed portal vein becomes replaced by a network of portoportal collaterals that bypass the obstructed venous segment. Collectively, these collaterals are referred to as a portal cavernoma . These enlarged portoportal collaterals can cause compression and deformation of the biliary lumen, termed portal cholangiopathy or portal biliopathy [120, 121]. In adults, chronic extrahepatic PVT is often complicated by recurrent gastrointestinal bleeding related to portal hypertension and hepatic encephalopathy due to extensive portosystemic shunting [101, 102, 122]. However, gastrointestinal bleeding is usually better tolerated than other causes of portal hypertension due to younger age of patients and preserved liver function.
Radiographic Findings and Diagnosis
A diagnosis of chronic PVT with cavernous transformation is often made by abdominal ultrasound, CT, or MRI that demonstrates serpiginous structures while the main portal vain and/or its main branches are not visible.
Treatment
Treatment for chronic PVT is divided into three approaches—prevention and treatment of gastrointestinal bleeding, prevention of recurrent thrombosis, and treatment of portal cholangiopathy. Endoscopic screening for gastroesophageal varices with beta-adrenergic blockers and/or endoscopic variceal ligation should be performed for patients with portal cavernoma as is the practice for patients with cirrhosis [108, 123]. Currently, there are no consensus guidelines for permanent anticoagulation in the setting of chronic PVT. As such, management should be developed on a case-by-case basis with consideration of the thrombotic potential of the underlying condition, the extension of the thrombus, and the increased risk of portal hypertensive bleeding. Retrospective studies including patients with acute and chronic PVT showed that anticoagulation therapy significantly decreased the risk of recurrent thrombosis without increasing the risk of gastrointestinal bleeding [101]. However, on account of the paucity of data, expert opinion recommends anticoagulation therapy for patients with chronic PVT only in those with a documented permanent prothrombotic condition [108]. Endoscopic or percutaneous therapies may be used for symptomatic management of portal cholangiopathy. Biliary surgery without portal decompression is extremely hazardous and best avoided.
Outcomes
The prognosis of patients who are anticoagulated for chronic PVT is good with a 5-year mortality from intestinal infarction or gastrointestinal bleeding of less than 5 % [101].
Budd-Chiari Syndrome
Definition and Etiology
Budd-Chiari syndrome (BCS) describes hepatic venous outflow tract obstruction independent of the level or mechanism of obstruction [115, 124]. Cardiac and pericardial diseases as well as sinusoidal obstruction syndrome are generally excluded from this definition. BCS is typically caused by thrombosis of the hepatic vein (HV) or the terminal portion of the inferior vena cava (IVC). BCS is a rare disorder with an estimated incidence of 0.2–0.8 per million per year [125–128]. Pure IVC or IVC/HV obstruction predominates in Asia, whereas pure HV obstruction predominates in Western countries [128]. BCS is divided into “primary” BCS when related to a primary venous disease (i.e. thrombosis or phlebitis) and “secondary” BCS when related to compression, thrombosis, and/or invasion by a lesion originating outside the veins (Table 26.8).
Table 26.8
Inherited and acquired risk factors for Budd-Chiari syndrome
Primary | Secondary |
---|---|
Myeloproliferative disorders | Hepatocellular carcinoma |
Polycythemia vera | Renal cell carcinoma |
Essential thrombocytosis | Adrenal adenocarcinoma |
Chronic myelogenous leukemia | Primary hepatic hemangiosarcoma |
Inherited thrombophilia | Epitheloid hemangioendothelioma |
Antiphospholipid antibody syndrome | Sarcoma of the IVC |
Parosysmal nocturnal hemoglobinuria | Right atrial myxoma |
Factor V Leiden mutation | Alveolar hydatid disease |
Prothrombin gene mutation | Cysts (parasitic/nonparasitic) |
Protein C deficiency | Abscess |
Protein S deficiency | Large FNH |
Antithrombin III deficiency | Hepatic resection or transplantation |
Factor II mutation | Blunt abdominal trauma |
Hyperhomocysteinemia | Pancreatitis |
Methylene-tetrahydrofolate reductase mutation | |
Acquired disorders | |
Pregnancy | |
Oral contraceptives | |
Systemic disease | |
Inflammatory bowel disease | |
Behcet disease | |
Human immunodeficiency virus | |
Sarcoidosis | |
Idiopathic |
Similar to PVT, BCS is strongly associated with prothrombotic conditions (Table 26.7). Myeloproliferative neoplasms, antiphospholipid syndrome, and oral contraceptive use are among the most common risk factors for BCS. More than 1 thrombotic risk factor may be present in 25–46 % of cases [90, 129]. Thus, routine screening for all thrombotic risk factors is recommended in patients diagnosed with BCS.
Clinical and Laboratory Features
The clinical presentation of BCS is variable and depends largely on the extent and rate of outflow obstruction, as well as the development of collaterals [130]. The clinical presentation ranges from asymptomatic to fulminant hepatic failure, or to cirrhosis with complications of portal hypertension [129, 131]. Nearly 20 % of BCS cases are asymptomatic [132]. Common signs and symptoms include abdominal pain, fevers, ascites, lower extremity edema, gastrointestinal bleeding, and hepatic encephalopathy [126, 133]. Dilated truncal subcutaneous veins are highly specific for IVC obstruction. Liver biochemical profile may be normal or increased. The protein level in ascitic fluid is variable, though ascites protein >2.5 g/dL with a serum-ascites albumin concentration gradient ≥1.1 g/dL is suggestive of BCS or cardiac/pericardial disease. The disease onset may be insidious or rapid. Given these nonspecific clinical and laboratory signs, BCS should be considered in all patients with acute or chronic liver disease [109, 115].
Radiographic Findings
Fluroscopic venography has long been the gold standard for evaluation of the HV. Fortunately, sonographic findings correlate well with venography [134–136] and pathologic examination [137]. Doppler ultrasound by an experienced examiner is often the most effective and reliable means of diagnosis [109]. MRI and CT can confirm the diagnosis if an experienced Doppler ultrasound examiner is not available. Caudate lobe hypertrophy is found in nearly 75 % of patients with BCS [80, 137]. This is due to separate venous drainage of the caudate lobe directly into the IVC that allows for sparing of the outflow with compensatory hypertrophy [138]. Thus, invasive procedures such as venography and liver biopsy are necessary only in patients when the diagnosis remains unclear after noninvasive radiographic procedures [109].
Histopathology
Sinusoidal dilation, congestion, and centrilobular fibrosis are characteristic histopathological features of BCS (Fig. 26.1) [139, 140]. Cirrhosis may ultimately be seen. In the most-advanced cases, thrombosis of intrahepatic portal veins may be seen with fibrous enlargement of the portal tract.


Fig. 26.1
Budd-Chiari syndrome. (a) Low magnification showing perivenular congestion and necrosis with intact perioportal parenchyma in a patient with Budd-Chiari syndrome (H&E). (b) Higher magnification demonstrates centrilobular hemorrhage and necrosis with an acute thrombus (H&E) (Courtesy of Monica T. Garcia-Buitrago, MD, University of Miami)
Management
The conventional management of BCS includes immediate anticoagulation, medical management of the complications of portal hypertension, and treatment of the underlying prothrombotic disease [141, 142]. Table 26.9 summarizes the algorithm for the treatment strategy of primary BCS [115, 124]. Similar to PVT, there are no prospective randomized controlled trials of anticoagulation in patients with BCS. For this reason, guidelines directing anticoagulation for patients with DVT are often extrapolated to patients with BCS, which support the use of indefinite systemic anticoagulation in patients who develop an idiopathic DVT with a permanent risk factor without definitive cure of the underlying thrombophilia [116]. Platelet count should be carefully monitored given the high incidence of heparin-induced thrombocytopenia in BCS patients [143, 144]. Oral contraceptives should be stopped. Percutaneous recanalization of the HV or ICV utilizing thrombolysis, angioplasty and/or stent should be considered in patients with short-length stenosis of main HV or IVC [145, 146]. TIPS has been successfully used in a subset of patients who did not respond to medical treatment or recanalization with excellent long-term survival [147]. Patients who develop cirrhosis from HV obstruction are less likely to respond to these measures and may need to be referred for LT.
Table 26.9
Proposed algorithm for treatment of primary Budd-Chiari Syndrome
1. In all patients with primary BCS |
– Anticoagulation as soon as diagnosis is established |
– Treatment of underlying (hematologic) condition |
– Symptomatic treatment of complications of portal hypertension |
– Look for short-length stenosis of HV or IVC and perform angioplasty/stenting |
2. Consider TIPS if patients are not suitable for or unresponsive to angioplasty/stenting |
3. Consider liver transplantation in patients unresponsive to TIPS |
Outcomes and Prognosis
The natural history of BCS is poorly understood. However, improvement in diagnostic and therapeutic strategies has resulted in 5-year survival rates approaching 90 % [129, 145, 146].
HCC is rare in BCS and is primarily seen in patients with long-standing disease, especially in those with obstruction of the suprahepatic IVC [89, 148–150]. Particularly challenging is differentiating HCC from benign macroregenerative nodules, which frequently develop in patients with well-controlled BCS [151]. Most of these nodules are benign and resemble focal nodular hyperplasia (FNH) on radiographic and pathologic examination.
Patients with splanchnic vein thrombosis and myeloproliferative disease are at increased risk of developing myelofibrosis or acute leukemia [97]. Therefore, despite adequate medical management, long-term prognosis may be threatened more by subsequent neoplastic disease than by liver failure. However, studies have shown that the slow course of myeloproliferative syndromes is not significantly affected by LT, and that LT is an acceptable option in these patients despite the potential negative long-term effects [152, 153].
Sinusoidal Obstruction Syndrome
Introduction
Sinusoidal obstruction syndrome (SOS), previously termed hepatic venooclusive disease (VOD) , is characterized by hepatomegaly, right upper quadrant pain, jaundice, and ascites. The acute form of SOS occurs most commonly as a consequence of myeloablative regimens used in preparation for hematopoietic stem cell transplant (HSCT) . These so-called conditioning regimens are combinations of high-dose chemotherapy drugs with or without total body irradiation (TBI) . A chronic, more indolent form of SOS may develop following toxicity of pyrrolizidine alkaloids from plants that are often ingested in the form of herbal teas, hence the term Jamaican brush tea disease. SOS clinically resembles BCS; however, HV outflow obstruction in SOS is due to the occlusion of the terminal hepatic sinusoids and venules rather than the HV and/or IVC in BCS.
Pathogenesis
SOS begins with injury to the HV endothelium. Specific to HSCT, sinusoidal endothelial cells (ECs) become activated by the conditioning regimen, drugs used during the procedure (i.e. granulocyte colony stimulating factor or calcineurin inhibitors) [154, 155], and the complex process of engraftment [156]. Activation of sinusoidal ECs trigger multiple pro-inflammatory pathways that result in accumulation of cells and debris in the space of Disse, the perisinusoidal space located between the endothelium and the hepatocyte, resulting in narrowing of the hepatic sinusoids. The ECs become detached and embolize to the central area of the lobule where they cause a postsinusoidal outflow obstruction, namely SOS.
Epidemiology and Risk Factors
Including myeloablative conditioning regimens, multiple other risk factors for the development of SOS have been identified (Table 26.10). The reported incidence between HSCT units range from 0 to 50 % [157–162]. An incidence of SOS around 20–40 % is seen more consistently in those who receive more liver-toxic regimens, which has led many centers to abandon high-dose conditioning regimens in favor of the increasingly popular reduced-intensity conditioning regimens that carry little or no risk for SOS. The regimens known to cause the most liver injury are those that contain cyclophosphamide in combination with either busulfan or TBI (greater than 12 Gy), regimens that include N,N-bis(2-chloroethyl)-N-nitrosourea (BCNU) or multiple alkylating agents [109].
Table 26.10
Risk factors for sinusoidal obstruction syndrome
Patient factors | Disease factors | Transplant factors |
---|---|---|
Younger age (in children) | Advanced malignancy | Myeloablative conditioning |
Older age (in adults) | Acute leukemia | Cyclophosphamide with busulfan |
Poor performance status | Neuroblastoma | Cyclophosphamide with TBI |
Preexisitng liver disease | Thalassemia major | Allogenic HSCT (greater than autologous) |
HCV or HBV | Delayed platelet engraftment | Unrelated donor HSCT |
Hepatic fibrosis | Presence of acute GVHD | Mismatched HSCT |
Iron overload | Abdominal radiotherapy | Subsequent transplants |
Positive CMV serology | Prior chemotherapy | Sirolimus GVHD prophylaxis |
Prior fungal infection | 6-mercaptopurine | Norethisterone use |
HFE C282Y genotype | 6-thioguanine | |
Exposure to toxins | Actinomycin D | |
Pyrrolizidine alkaloids | Azathioprine (Imuran) | |
Herbal medications | Cytarabine | |
Cytosine arabinoside | ||
Dacarbazine | ||
Gemtuzumab ozogamicin (Mylotarg) | ||
Melphalan (Alkeran) | ||
Oxaliplatin (Eloxatin) | ||
Urethane |
Clinical and Laboratory Findings
The clinical signs and symptoms of SOS include weight gain with or without ascites, right upper quadrant pain of liver origin, hepatomegaly, and jaundice [109]. Symptom onset is typically between 10 and 20 days after initiation of therapy using cyclophosphamide-containing regimens, but may be as late as 30 days after completing non-cyclophosphamide myeloablative therapy [162–164].
Diagnosis
Diagnostic criteria have been published to define SOS, which include the Seattle and Baltimore criteria (Table 26.11) [160, 162]. Most patients who receive liver-toxic conditioning regimens will develop some degree of sinusoidal injury, even in the absence of clinical signs and symptoms [165]. Confounding diagnoses that are also common in this population include sepsis-induced cholestasis, drug-induced cholestasis, fluid overload from renal failure or congestive heart failure, liver involvement of fungal or viral infections in the setting of immunosuppression, and (hyper)acute graft-versus-host disease (GVHD).
Table 26.11
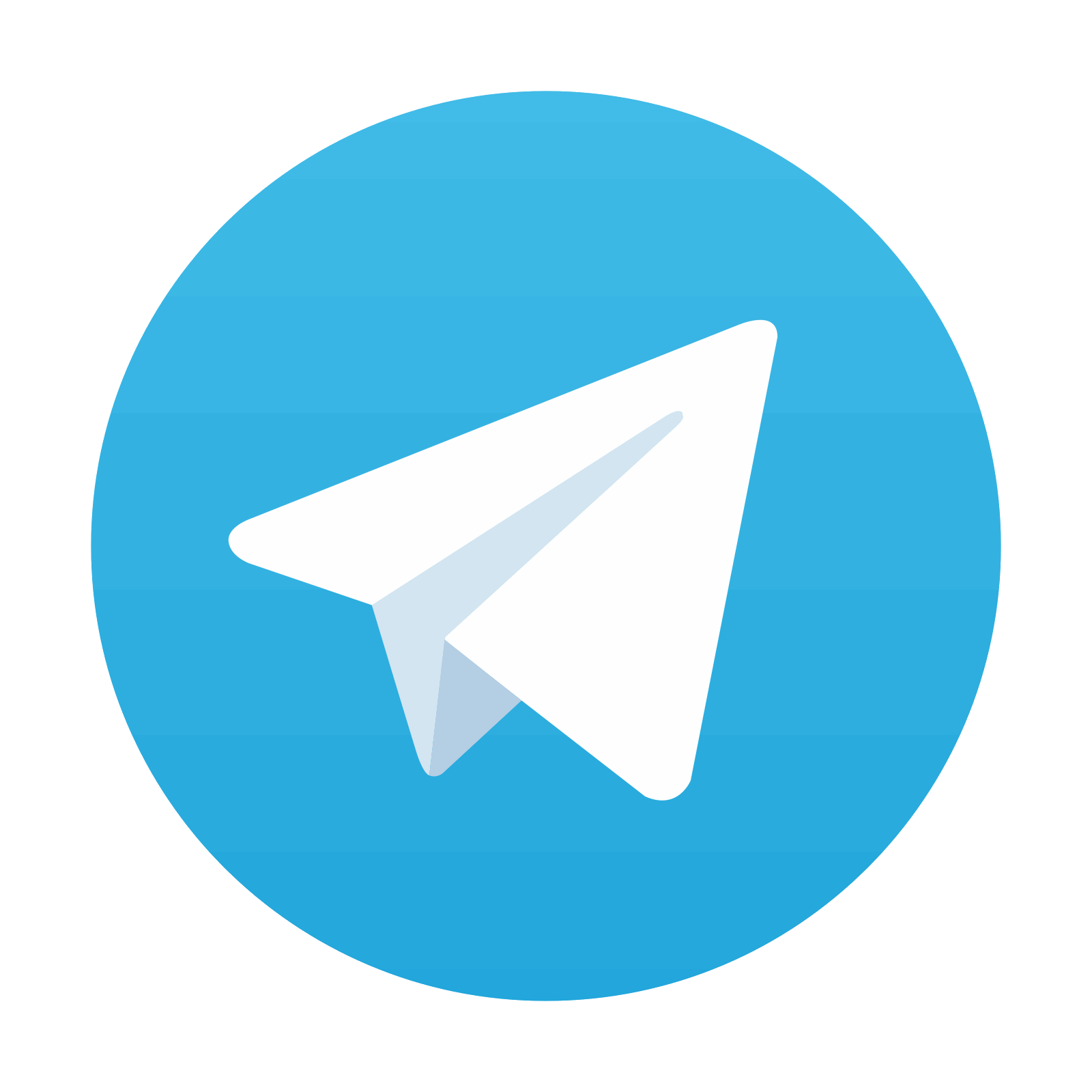
Diagnostic criteria for SOS after hematopoietic stem cell transplantation
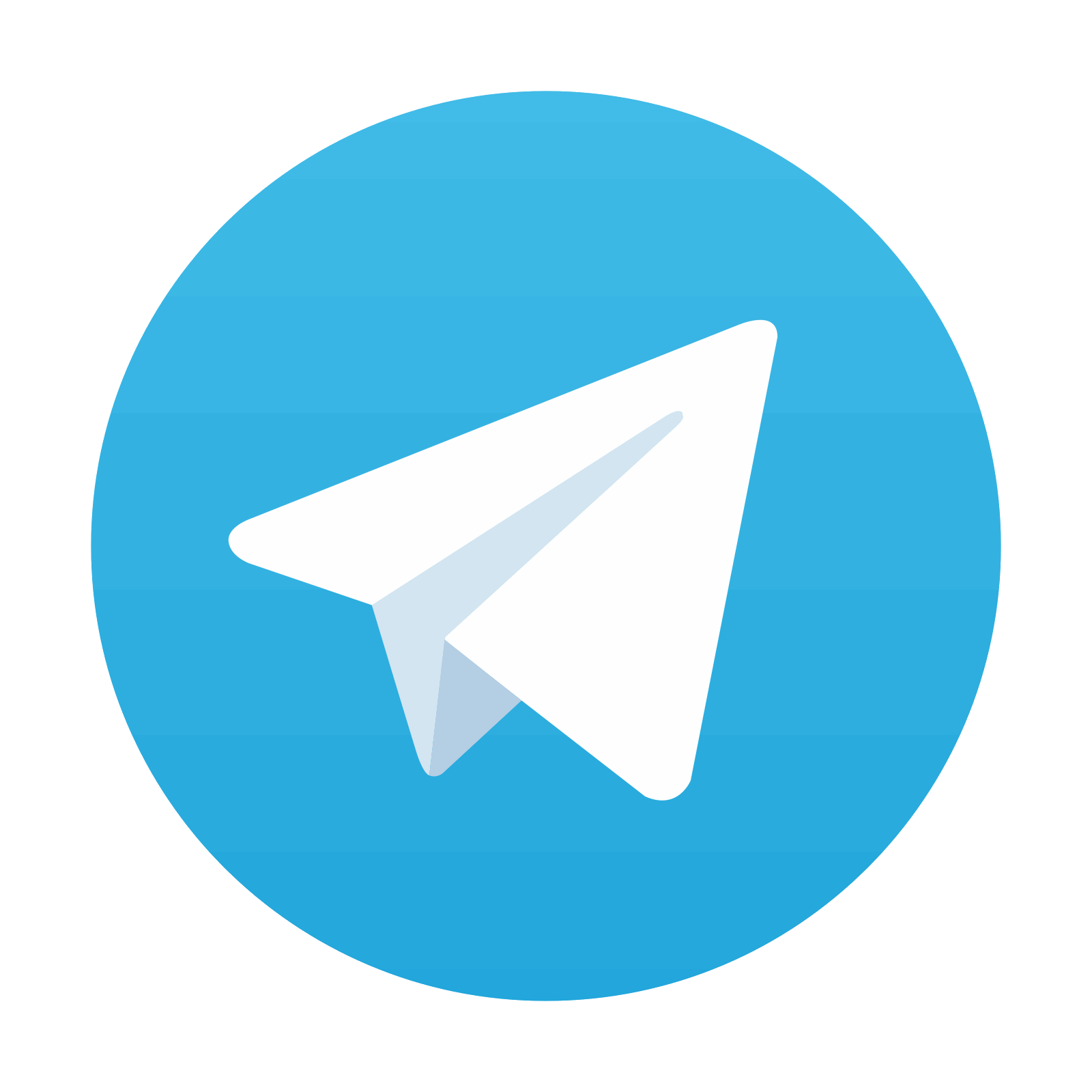
Stay updated, free articles. Join our Telegram channel

Full access? Get Clinical Tree
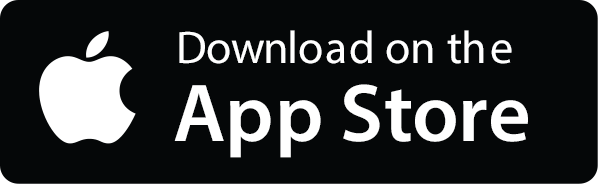
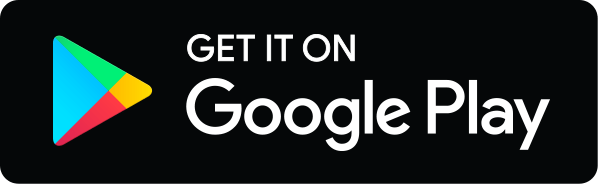
