Outline
Introduction 217
Methods for the Isolation and Culture of Bone Marrow Stem Cells 220
Isolation of the Bone Marrow or Cord Blood Mononuclear Fraction 220
Isolation and Cultivation of Hematopoietic Stem Cells 221
Isolation and Culture of Renal Progenitor Cells 223
Methods for the Isolation and Culture of Mesenchymal Stem Cells 223
Isolation and Culture of Mesenchymal Stem Cells from Human Bone Marrow 223
Isolation and Culture of Mesenchymal Stem Cells from Murine Bone Marrow 224
Isolation and Culture of Mesenchymal Stem Cells from Human Adipose Tissue 225
Isolation and Culture of Human Adipose Tissue-derived Pericytes 226
Acknowledgments 227
Regenerative medicine has benefited from the increasing knowledge of the characteristics of various types of stem cells, provided by a growing number of methods that allow the isolation and culture of these cells. To date, the clinical use of stem cells other than hematopoietic stem cells remains largely experimental, and this is reflected in the field of regenerative nephrology. This chapter will discuss some concepts and describe methods for the isolation and culture of stem/progenitor cells that show promise for use in future clinical protocols aimed at kidney regeneration, with particular emphasis on mesenchymal stem cells.
Introduction
The advances in the methods of isolation and in vitro or ex vivo manipulation of stem cells that have been taking place in the past few decades have changed the perspectives in the field of regenerative medicine. For one thing, these methods have allowed for the understanding that almost all, if not all, tissues contain primitive cells that are able to give rise to mature, tissue-specific cells. In addition, the feasibility of generation of specialized cell types in vitro creates the perspective that cells lost owing to pathological processes or accidents can be replaced by cells produced from these primitive cells.
The natural path for tissue reconstruction would be, therefore, isolating and manipulating stem cells to obtain mature cells. The relative numbers of stem cells in different tissues are, however, intrinsically low, which calls for their expansion in vitro before differentiation so that an adequate number of mature cells is yielded. Consequently, different methodologies focus on the in vitro expansion of stem cells.
Expansion of stem cells in vitro is not always easy. Perhaps unnoticed is the fact that primitive cells are slowly cycling under physiological conditions in vivo, as demonstrated by experiments that focused on label-retaining cells or cell sorting based on metabolic activity . In humans, the lifespan of most cells is limited by progressive erosion of the extremities of chromosomes, which occurs at each cell division cycle owing to the lack of expression of the enzyme telomerase by somatic cells . Therefore, if stem cells underwent as many divisions as required to maintain some tissues that exhibit a high turnover rate such as the blood or skin, replicative senescence would be achieved very quickly and cell replacement would soon cease. This does not happen, only because stem cells do not replicate frequently; instead, they occasionally give rise to less immature cells that also possess some self-renewal capacity which, in turn, give rise to other cells in a more advanced stage of maturation. It is, therefore, the hierarchical structure in which stem cells are intrinsically embedded rather than stem cells themselves that is responsible for the maintenance of the different tissues. However, the existence of such a hierarchy adds complexity to stem cell research because it is sometimes difficult to tell exactly which cells are stem cells. One of the simplest definitions of a stem cell is a cell that is able to self-renew and also give rise to at least one type descendant at a more advanced differentiation state. In that case, many instances of the cellular hierarchy of a given tissue would represent stem cells. As an alternative to this and other stem cell concepts, stem cells could be defined as cells that reside at the top of the cellular hierarchy of the tissues to which they belong.
Research on hematopoiesis has greatly contributed to the current paradigms that concern stem cells. Early studies have shown that the hematopoietic compartment of mice can be ablated by irradiation and rescued by transplantation of bone marrow cells. Later, it was found that some cell populations within bone marrow are able to reconstitute hematopoiesis. However, depending on the cell population used, restored hematopoiesis lasts for shorter or longer periods [see Ref. for a review]. Therefore, it is the bone marrow cell populations able to restore long-term hematopoiesis that contain the cells at the top of the hematopoietic hierarchy, which correspond to hematopoietic stem cells (HSCs). Experiments such as these have contributed to the definition of stem cells by showing that stem cells can not only self-renew and give rise to more differentiated cells in vivo, but also sustain their downstream cell hierarchy for a lifetime.
Although many studies have established that stem cells are present in almost all, if not all tissues, it is not always easy to recognize these cells. Perhaps the most important advance in the identification of stem cells was the development of monoclonal antibody technology, which allowed reproducible detection of molecules present on different types of live cells . Consequently, cell populations could be defined based on the expression of a set of molecules on their surfaces. The conjugation of monoclonal antibodies with fluorescent fluorochromes and the subsequent introduction of fluorescence-activated cell sorting (FACS) systems allowed for the isolation of specific cell populations based on the presence or absence of specific molecules. In spite of this, to date, most methodologies used for the isolation of stem cells fail to provide the whole range of cells that represent the stem cell compartment of a given tissue. When different combinations of surface molecules in addition to the ability to exclude the Hoechst 33342 dye were used to purify HSCs by FACS, it was found that none of the combinations could encompass the entire HSC compartment . A possible explanation for such a finding is that stem cell compartments are heterogeneous.
In contrast to a prospective approach based on the expression of surface molecules, enrichment of stem cells can also be achieved by other means. Mesenchymal stem cells (MSCs), for example, can be enriched by adherence to a plastic surface and propagation in culture . In contrast to HSCs, which tend to differentiate or not proliferate when cultured , MSCs show a marked ability to proliferate under simple culture conditions (see Methods for the Isolation and Culture of Mesenchymal Stem Cells, below). This characteristic is, however, incongruent with the fact that the cellular turnover rate in most mesenchymal tissues is far lower than in others such as blood or skin. The low turnover rate in mesenchymal tissues hinders the use of functional assays to identify which cell is at the top of the hierarchy in their corresponding tissue.
To date, cultured cell populations are operationally defined as MSCs based on their ability to differentiate along osteogenic, adipogenic and chondrogenic pathways in vitro and to form bone when implanted in vivo . One of the oldest criticisms of cell culture, namely, that the conditions are artificial and may therefore skew results, affects MSC research as well. Some mature mesenchymal cells can dedifferentiate in culture and exhibit characteristics of in vitro-defined MSCs , and this undermines the use of markers expressed by in vitro MSCs to seek for MSCs in vivo. Notwithstanding, several studies have found that perivascular cells give rise to mature cells in vivo under physiological conditions or injury situations (reviewed in ref. ). However, a hierarchy for perivascular cells has not been defined yet. It is possible that some of these perivascular cells are bona fide stem cells, whereas others represent progenitor cells. For this reason, the study of the characteristics of subpopulations of perivascular cells shows promise to answer the question as to which cells correspond to MSCs in vivo. The evidence indicates that cells that surround endothelial cells, referred to as pericytes herein, are the cells that most likely fulfill this role. Indeed, CD146 + cells from the bone marrow (adventitial reticular cells) have been shown to transfer the hematopoietic supportive environment in vivo , and fresh CD146 + /CD34 − cells from various tissues have been shown to form muscle fibers when directly injected into cardiotoxin-injured mice . However, although CD146 is a marker for pericytes, it is also a marker for endothelial cells . Further, these studies do not exclude the possibility that the CD146 + cells used represent progenitor cells rather than stem cells.
In an attempt to put studies such as those mentioned above in perspective, the authors have proposed a model for the role of perivascular cells under physiological conditions and later extended it to include the behavior of perivascular cells during tissue injury . In these models, pericytes correspond to stem cells. Under physiological conditions, these cells would physically support blood vessels, secrete some factors important for tissue maintenance and give rise to progenitors only at a very low rate. Under injury conditions, however, some of the pericytes in or at the surroundings of the insulted site would become activated, proliferate and secrete various soluble factors that would contribute to resolution of the lesion. The behavior of pericytes facing tissue injury can be seen, thus, as a process rather than a single action. This behavior can be compared to that of B cells: under physiological conditions, B cells remain as B cells until exposed to a specific context, when they become activated, proliferate and become effector cells called plasma cells.
Primary MSC cultures contain various cell types and mimic some aspects of tissue injury as cells are deprived of specific signals provided by their native environment. It is possible that these conditions allow for pericyte activation as well as changes in other cell types present; consequently, in vitro MSCs can be viewed as cell populations that contain effector cells for tissue injury. Accordingly, the secretion of paracrine factors by in vitro MSCs is a remarkable characteristic of these cells and accounts for most of their therapeutic effects . These unique aspects of MSCs show importance when defining which cells to isolate for use in a therapeutic approach. If the most important cells present in MSC cultures comprise the progeny of pericytes, direct isolation of these cells could improve the efficiency of a therapeutic protocol. In contrast, isolated pericytes that are not exposed to the conditions that lead to the establishment of MSC cultures may not exhibit the full range of trophic and immunomodulatory properties characteristic of in vitro MSCs.
Whereas adhesion to plastic is a simple selection technique that can be used to obtain cell populations with promising therapeutic properties, other isolation procedures may provide more specialized cells. The combination of FACS with the ability of some cells to exclude specific dyes, for example, also provides a means of enrichment for some cell types. This was first demonstrated for the enrichment of HSCs. It was found that bone marrow cells that exclude the Hoechst 33342 dye are enriched for the presence of HSCs by a 1000-fold factor . A FACS strategy based on poor Hoechst uptake has also been found to be effective for the enrichment of slowly cycling kidney cells with tubulogenic capacity . Likewise, the expression of some enzymes associated with a primitive phenotype can be detected by incubation of the cells with substrates that become fluorescent after modification by the enzyme and used as a criterion for isolation by FACS .
Another method that has been used for the enrichment of stem cell-containing populations consists of centrifugation of a stem cell-containing tissue in density gradients, which provides a layer of mononuclear cells devoid of erythrocytes. Although this method is mentioned last in this introductory text, it was one of the first to be used for the enrichment of stem cell-containing populations. More specifically, it has been widely used for obtaining bone marrow mononuclear cells (BMMNCs), which correspond to a highly heterogeneous population that contains at least two different types of stem cell (HSCs and MSCs). BMMNCs obtained by centrifugation in density gradients have been widely used in many different experimental protocols. The main advantages of using BMMNCs include minimal manipulation and presence of different cell types that may be beneficial for therapeutic purposes. The disadvantages of BMMNCs include a low frequency of stem and progenitor cells and the presence of immune cells that can cause complications. Consequently, the use of BMMNCs in experimental therapeutic protocols has declined, and the use of more defined cell populations is usually preferred.
In view of the above, it is clear that each type of stem cell has its own particularities when it comes to history, biology, conceptual problems, isolation and in vitro or ex vivo manipulation. The success of a given experimental therapeutic protocol is dependent, therefore, on the cell population used and the enrichment method utilized to obtain such a population. To date, bone marrow transplantation and umbilical cord blood (UCB) transplantation for hematological diseases (e.g. leukemias, anemias) are the only clinically established applications of stem cells. All other types of stem cell therapy are experimental, and should follow appropriate ethical guidelines. Even for non-hematological diseases, however, BMMNCs or mobilized peripheral blood stem cells collected by leukapheresis are the most usually applied stem cell populations. Purified HSCs are also used, but much less frequently. The methods used to isolate and cultivate some of these cell types will be discussed. Given the growing number of studies that investigate the use of MSCs for cell therapy of many diseases, including kidney regeneration, special attention will be given to methods involved in the processes used for the isolation, enrichment and culture of this cell type.
Methods for the Isolation and Culture of Bone Marrow Stem Cells
Based on the premise that all tissues have their own stem cell compartment, it should be possible to isolate stem cells from any of them. However, as mentioned earlier, our poor knowledge of morphological or molecular stem cell markers hinders their prospective identification in most cases. The stem cell fraction of many tissues may be enriched by different methods, and further cultivation selects cells that are operationally described as stem cells by their properties of proliferation and differentiation into mature cells. It should always be taken into consideration, however, that the in vitro cultivation conditions do not reproduce the in vivo niche, so that we end up with cells that are certainly different from their physiological counterparts. Nevertheless, their “stemness” level is high enough for basic, preclinical or clinical studies to be conducted with consistent results and conclusions .
For most tissues, the isolation of stem cells aims at allowing the investigation of basic biological characteristics. Adult stem cell populations usually employed in clinical trials include mononuclear cells obtained from the bone marrow, cord blood or peripheral blood after mobilization, as well as MSCs isolated from the bone marrow or adipose tissue. The isolation and cultivation of bone marrow-derived stem cells will be presented below.
Isolation of the Bone Marrow or Cord Blood Mononuclear Fraction
The mononuclear fraction of bone marrow includes lymphocytes, monocytes, several types of blast cells, and at least three populations of stem/progenitor cells: HSCs, MSCs and endothelial progenitor cells (EPCs). EPCs were first described as occurring in circulating blood , and their origin in bone marrow was later proposed . However, some studies indicate that bone marrow-derived EPCs do not significantly contribute to neovascularization/angiogenesis, which could be attributed to poor knowledge of the hierarchy of the EPC compartment . The application of EPCs in kidney disease has been reviewed .
It has been suggested that the bone marrow also contains a plethora of versatile tissue-committed, non-hematopoietic stem cells . It is accepted today that the main therapeutic activity of bone marrow cells for non-hematological diseases is due to MSCs . The frequency of MSCs is much more limited in the UCB , so that the mononuclear fraction of cord blood is more appropriate for the treatment of hematological diseases than other pathologies.
The isolation of mononuclear cells from human blood was first reported by Dr Arne Bøyum in 1968 , and has been used successfully ever since. The method is based on the lower buoyant density of mononuclear cells compared to erythrocytes and polymorphonuclear leukocytes (granulocytes). Most mononuclear cells have densities below 1.077 g/ml, so that they can be isolated by centrifugation on an isoosmotic medium with this density. The two types of most widely used reagents are Lymphoprep™ (AXIS-SHIELD PoC AS) and Ficoll-Paque™ (GE Healthcare), which have recently been shown to yield BMMNC fractions equivalent in terms of the composition and quantity of cell types .
For clinical trials, bone marrow is usually collected by aspiration from the anterosuperior iliac crest, performed approximately 3 h before the reinfusion and with the patient under sedation. The volume aspirated is around 80 ml, in a medium containing anticoagulant preservative. When the cells are to be used for research purposes, the procedure may be different. Although some institutions have access to bone marrow samples donated for research, in most cases alternatives to whole bone marrow must be implemented, including the use of bone marrow remnants in bags and nylon meshes used for collecting bone marrow for transplantation . When bone marrow is collected for research purposes, it is usually obtained by a single puncture of the iliac crest using a 20 ml syringe. The quality of the sample depends on some factors. The site where the bone marrow is aspirated from can influence the amount of cells obtained as more or less medullar blood or fat can be present.
Human UCB is collected after clamping of the cord during vaginal deliveries. The blood is collected into sterile blood bags containing anticoagulant. The volume of blood collected varies usually between 70 and 120 ml. In either type of sample, the anticoagulant used is an important factor, which is sometimes overlooked. Heparin is a very popular (and effective) anticoagulant. However, heparin has soluble factor-binding activities that can influence the quality of the cells obtained. Hence, the amount of heparin should be just enough to prevent coagulation. Alternatives to heparin include sodium citrate and ethylene-diaminetetraacetic acid (EDTA), which are Ca 2+ chelating agents and tend not to interfere with the quality of the cells when used at the right concentrations.
Bone marrow or cord blood is then diluted 1:1 with 2 mM EDTA in phosphate-buffered saline (PBS-EDTA), and light-density mononuclear cells are separated by centrifugation on Ficoll-Hypaque 1.077, at 400 × g for 30 min. If the method, which is conventionally used for the isolation of mononuclear cells from peripheral blood, results in contamination with red cells, an enrichment step with 3% gelatin in 0.9% saline may be used before centrifugation on Ficoll-Hypaque. For that, the sample is mixed 1:1 with gelatin solution and incubated at room temperature for 2 h. The mononuclear-enriched supernatant is collected and centrifuged (10 min, 400 × g ) and the pellet is centrifuged on Ficoll-Hypaque.
Cells are then counted using a hemocytometer or Neubauer chamber. Although intact cells can be discerned from damaged cells by counting under a phase-contrast microscope, viable cells can be more accurately identified using a stain such as trypan blue. To assess cell viability, an aliquot of the parent cell suspension is mixed with an equal volume of 0.4% trypan blue in PBS and placed in the hemocytometer. Cells permeable to trypan blue are stained blue and correspond to non-viable cells. These are scored along with the viable cells and cell viability is calculated by dividing the number of viable cells by the total number of cells; red blood cells (RBCs) are recognized by eye and not included.
For clinical studies, the mononuclear fraction is collected, washed in a heparinized saline solution containing 5% autologous serum and filtered for removal of cellular adjunctive. The cells are counted, suspended in saline/5% autologous serum in the concentration determined and administered to the patient by local or systemic injection. A small fraction should be separated for sterility and viability tests, immunophenotyping by flow cytometry to determine the cell subpopulations present, and for functional analyses.
Isolation and Cultivation of Hematopoietic Stem Cells
Human HSCs are characterized as CD34- or CD133-positive, CD38- and lineage-negative cells. For clinical applications, even in cases when the treatment is referred to as hematopoietic stem-cell transplantation, the cells which are used are actually populations enriched, but not purified, for HSCs . A usual method of enrichment for HSCs which avoids the need for bone marrow collection is mobilization with differentiation/growth factors such as the granulocyte colony-stimulating factor (G-CSF), followed by blood collection by leukapheresis . More recently, combinations of growth factors and other molecules, such as plerixafor, have been developed for more efficient concentration of HSCs in the peripheral blood .
With much less frequency, patients receive purified HSCs , which may be isolated from bone marrow, peripheral blood after mobilization or UCB . In these cases, the cells are selected by reaction with anti-CD34 or anti-CD133 antibodies followed by two alternative methods of isolation of positive cells. The first one is FACS, as described below for the isolation of pericytes. The second method, which preserves more of the biological characteristics of the cells, is magnetic cell sorting (MACS). In this case, the cells are collected, washed and incubated with an antibody conjugated to magnetic microbeads at 4°C. The cells are then washed, resuspended in the appropriate buffer and loaded on a magnetic separation column which is placed in a magnetic field. Cells negative for the marker are then eluted from the column, which preferentially retains the positive cells. After removal from the magnetic field, the positive cells are eluted. To increase purity of the sorted cells, the eluted cells may be subjected to an additional purification cycle.
All blood cells are derived, through a series of maturational cell divisions regulated by the hematopoietic microenvironment, from a small common pool of HSCs. HSCs are of interest not only because of their developmental capacity but also because of their therapeutic role in hematological disorders and gene therapy. They share the main characteristics presented by adult stem cells: (i) they constitute a very small compartment, estimated between less than 0.05% and up to 0.5% of cells in the bone marrow; (ii) they are normally quiescent or slowly cycling, as shown for instance by their resistance to treatment with 5-fluorouracil or 4-hydroperoxycyclophosphamide. Estimates of periodicity of mitosis vary widely, and the direct examination of the cell cycle of long-term cells indicates that at any moment only 4% of them are in the S/G2/M phases. Besides bone marrow, HSCs can be found in UCB and in peripheral blood, particularly after mobilization treatments.
When stem cells divide, they may return to the G0 phase of the cell cycle, generating more stem cells, or generate large numbers of committed progenitors with a progressively restricted differentiation potential. Homeotic genes have a fundamental role in the regulation of these and other cellular processes . Intermediate steps, characterized by the progressive loss of the self-renewal capacity and the commitment to a specific cell lineage, result in the transition from stem cells to mature hematopoietic cells.
Despite much effort, the in vitro cultivation of HSCs is still poorly developed . Many different combinations of growth/differentiation factors , or functional parameters , are under investigation to define the conditions allowing expansion of this cell population with maintenance of stemness. However, as different assays detect and analyze hematopoietic cell types specifically stimulated by the experimental conditions used, and the correspondence among the assays is not always easily established, different names have been given to the cell types observed, as detailed below.
Till and McCulloch established, in 1961, the first quantitative assay for cells with a radioprotective effect. Although these cells, which were denominated spleen colony-forming units (CFU-S), do not represent the more primitive stem cell pool, the assay is useful for the investigation of early events in hematopoiesis. In vivo assays involving the engraftment of human hematopoietic cells into immunodeficient mice have shown the existence of pluripotent cells, either by limiting dilution analysis or by clonal integration of a retroviral marker gene . The expression “marrow repopulating ability”, derived from in vivo studies, refers to primitive HSCs capable of repopulating the bone marrow of lethally irradiated mice .
Two different types of cells with marrow repopulating ability have been distinguished in the mouse. Initial engraftment (short-term repopulation) is a characteristic of CFU-S. Long-term engraftment is attributed to a different cell type and is possible only if the animals also receive short-term repopulating cells. A cell type which is more primitive than CFU-S (pre-CFU-S) is considered to be responsible for long-term marrow repopulating ability [reviewed in Ref. ].
The development of in vitro cultivation systems for the study of hematopoiesis allowed the identification and quantification of several different types of hematopoietic precursor cells. The colony formation assay allows the identification and quantification of early progenitor cells, capable of forming colonies when cultured in semisolid medium. In this assay, cells are grown in vitro in methylcellulose or other highly viscous media, containing also different combinations of growth and differentiation factors. These semisolid media reduce cell movement and allow individual cells to develop into cell clones that are identified as single clusters (< 50 cells) or colonies (> 50 cells) of differentiated cells after a culture period of 7–14 days. These colonies are the progeny of single cells called colony-forming cells (CFCs) or colony-forming units (CFUs), and the composition of the colonies determines which CFU is being assessed. These clonogenic assays allow the identification, for instance, of CFU-blasts which give rise to colonies composed of cells with blast-like morphology, of CFU-GEMM which originate multilineage colonies (granulocytes, erythrocytes, monocytes and megakaryocytes), CFU-meg which give rise to megakaryocyte colonies, and so on . Other types of stem/precursor cells identified by this culture system include the high proliferative potential colony-forming cells (HPP-CFC), defined by the ability to form very large colonies (> 5 mm) containing approximately 50,000 cells, and the low proliferative potential colony-forming cells (LPP-CFC), that give rise to colonies smaller than 1 mm. These primitive HSC pools are considered to comprise cell types such as BFU-E (erythrocyte blast-forming unit) and CFU-GM (precursor of granulocytes and monocytes) .
Another culture system currently used for the study of hematopoietic progenitors is the delta assay . In this system, cells are grown first in liquid culture for 1 week and then replated onto semisolid medium. Colonies observed after this period indicate the number of hematopoietic progenitors more primitive than those normally obtained after 14 days of growth in semisolid medium.
The development of hematopoietic cells in these culture systems depends on the continuous presence of colony-stimulating factors (CSFs). The investigation of results obtained with addition of different types of stimulating molecules has been the key to the discovery and characterization of many of the hematopoietic growth factors . Colony-forming assays, therefore, allow the study of the role of growth factors or cytokines in the determination of the lineage along which CFCs differentiate.
Expansion of HSCs is not possible with the use of standard semisolid systems; consequently, alternative culture assays have been developed for the sustained production or self-renewal of clonogenic cells. In the long-term culture (LTC) system originally described by Dexter for murine cells and later adapted for human cells , cells are cultivated at a lower temperature in a culture medium containing high concentrations of horse serum and hydrocortisone. This supportive microenvironment allows the self-renewal of stem cells over a period of several months. The long-term culture of bone marrow cells employs primary adherent layers of stromal cells which include MSCs as important components. These stromal cells provide a complex functional extracellular matrix which allows direct cell–cell contacts between different cell types and secrete cytokines and low molecular weight substances which are required for the controlled differentiation and proliferation of hematopoietic progenitor cells. This system can maintain primitive stem cells, induce early differentiation of a fraction of the primitive progenitors and prevent their terminal differentiation.
A second type of widely used culture system for hematopoietic cells is the Whitlock–Witte long-term bone marrow culture , which was initially developed for murine bone marrow. In this lymphoid culture system, a “poor” culture medium containing 5% fetal calf serum and no cortisone permits the growth of freshly isolated bone marrow cells that develop into a confluent adherent stromal cell layer within 2–3 weeks. Whitlock–Witte cultures can reconstitute the B-lymphocyte compartment in immunocompromised mice, but do not maintain primitive multilineage hematopoietic precursors such as CFU-S . Pluripotent HSCs and early precursors can also be identified by the LTC assays as cobblestone area-forming cells (CAFCs) and long-term culture-initiating cells (LTC-ICs). Limiting dilution analysis is used for the quantification of these cells .
The transduction of CFU cells and LTC-ICs using retroviral vectors indicates that the in vitro progenitor assays currently available measure functionally different, and presumably less quiescent, populations than the long-term repopulating stem cells . However, none of these culture systems is able to maintain hematopoiesis indefinitely, showing that the in vivo niche of HSCs has not yet been adequately reproduced in vitro. It is not probable, therefore, that clinical application of isolated, cultivated HSCs will be available in the near future.
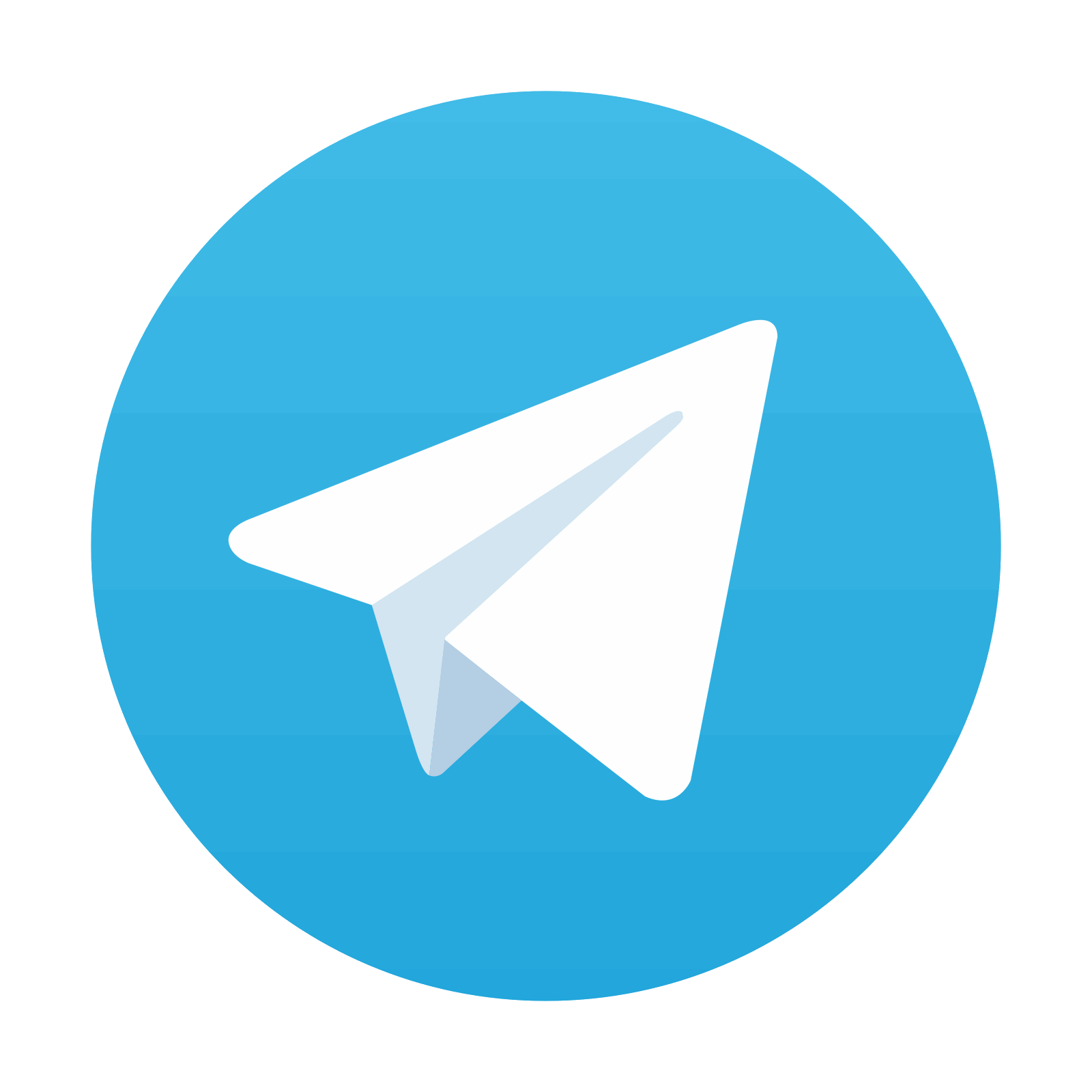
Stay updated, free articles. Join our Telegram channel

Full access? Get Clinical Tree
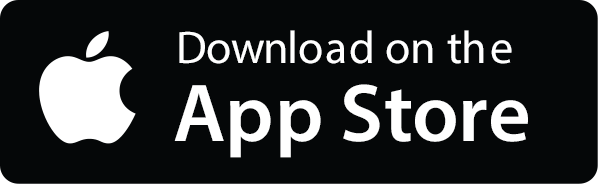
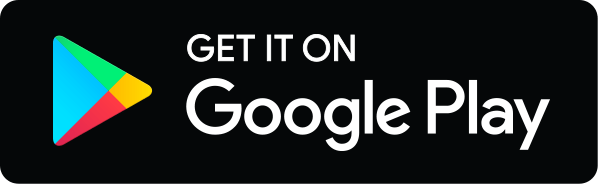