An inherited metabolic disorder is an increasingly common indication for liver transplantation (LT), accounting for more than 15% of pediatric cases worldwide and up to 30% in some specialist centers. Urea cycle disorders are now the most common indication in the United States, followed by alpha-1 antitrypsin deficiency.
In an individual child and family, the therapeutic decision has to incorporate the transplant center’s results and experience, the impact of the metabolic defect on the child and family, the natural history of the defect, and whether any new therapies are available now or in the future. The transplantation team should be supported by metabolic specialists when metabolic disease is the indication and should be able to call on other relevant organ-based specialists, as required. An individual perioperative protocol should be prepared that incorporates instructions for immediate pre-operative preparation and investigations, which individuals and teams to contact, and a summary of intraoperative and early post-transplantation management.
In considering the indications for and outcomes of LT in metabolic disease, two independent factors need to be considered: first, whether or not the metabolic defect causes significant liver disease; and second, whether or not the defect is confined to the liver. This results in four categories of disorders to consider ( Table 39.1 ).
Category | Liver Disease a | No Significant Liver Disease |
---|---|---|
Defect confined to liver (A) | Alpha-1 antitrypsin deficiency (PiZ) Tyrosinemia type 1 Wilson disease PFIC types 2, 3, and undefined Urea cycle disorders: ASLD (arginosuccinic aciduria) GSD types I (adenoma, HCC) and IIIb Indian childhood cirrhosis Bile acid synthesis disorders Hereditary hemochromatosis | Crigler-Najjar syndrome type 1 Primary hyperoxaluria type 1 (preemptive) Urea cycle disorders (arginosuccinic aciduria excepted) Citrin deficiency Familial hypercholesterolemia Hemophilia Factor VII deficiency Protein C and S deficiencies Factor H deficiency Fatty acid oxidation defects Acute intermittent porphyria Familial amyloid polyneuropathy Afibrinogenemia |
Extrahepatic defects (B) | GSD types Ib, IIIa, and IV Erythropoietic protoporphyria Lysosomal storage diseases Niemann-Pick disease Cystic fibrosis Respiratory chain disorders PFIC types 1 and 4 Cholesterol ester storage disease | MSUD Methylmalonic acidemia Propionic acidemia Primary hyperoxaluria type 1 (with renal failure) S-Adenosylhomocysteine hydrolase deficiency Ethylmalonic encephalopathy Mitochondrial neurogastrointestinal encephalomyopathy |
Category 1: Intrinsic Liver Disease With Defect Confined to the Liver
In this group, the indications for transplantation depend on the severity of the liver disease rather than that of the intrinsic metabolic defect. If the defect causes a significantly higher risk of developing hepatocellular carcinoma (HCC), such as in tyrosinemia type 1 (HT1), transplantation maybe indicated at an earlier stage than if it were based on the severity of liver disease alone.
For this category, the outcome of LT will reflect the success of the transplantation program, the clinical status of the child at transplantation, and possibly the age at transplantation. LT for acute liver failure (ALF) is less successful than elective transplantation. The effect of age at transplantation is more unpredictable. LT in early infancy is less successful, but considering that these are very ill children with ALF, and that waiting times are usually longer for this age group, this is hardly unexpected. Beyond 6 months old, age at transplantation has little impact on outcome. Children with established liver disease usually have some degree of portal hypertension, tend to develop collateral circulation, and hence tolerate the effect of intraoperative portal vein clamping relatively well.
Post-transplantation, there is lifelong correction of the metabolic defect, with the possible exception of progressive familial intrahepatic cholestasis type 2 (PFIC2), in which functional recurrence is possible, and the long-term prognosis is similar to that of contemporary LT for other indications.
Progressive Familial Intrahepatic Cholestasis Type 2
PFIC2 is caused by deficiency of the canalicular bile salt export pump (BSEP) as a result of mutations in ABCB11 . Mutations that result in some expression of BSEP have a less severe phenotype than those resulting in no expression of BSEP. The most common clinical presentation of the severe phenotype is neonatal jaundice and subsequent pruritus. Biochemical tests show normal or low serum gamma-glutamyltransferase (GGT) activity, and liver histology typically demonstrates a severe giant cell hepatitis.
Treatment consists of supportive nutritional and medical care, with timely partial external biliary diversion in those with preserved BSEP expression. Unfortunately, progression to cirrhosis is common and, when this is established, or biliary diversion fails, LT is the only lifesaving option.
Overall, LT has been very successful and, in most cases, provides functional correction of the defect. However, as many as 8% to 10% develop recurrence of cholestasis between 3 months and 10 years post-transplantation. This has been shown to be the result of de novo anti-BSEP antibodies, which inhibit bile acid transport. This phenomenon is confined to those with severe mutations in which there is little or no BSEP expression. In this setting, BSEP protein escapes normal immune autotolerance and becomes an immune target when expressed in the allograft.
Treatment options include manipulating immunosuppression, plasmapheresis, rituximab, and, in intractable cases, bone marrow transplantation. When repeat LT has been required, the incidence of recurrent disease is very high.
Tyrosinemia Type 1
HT1 is caused by a deficiency of the enzyme fumaryl-acetoacetase (FAA), resulting in the formation of toxic intermediate metabolites, including succinyl-acetone (SA), which is pathognomonic. HT1 most commonly presents with liver failure in the first 6 months of life, but may present later with chronic liver disease, rickets, and failure to thrive. Survivors have a high risk of developing HCC. HT1 is diagnosed by the detection of urinary SA and confirmed by detection of two pathogenic mutations. Increasingly, HT1 is being diagnosed preemptively by universal newborn screening initiated in some countries.
The natural history of the disease has been transformed by the use of nitisinone, which, in combination with phenylalanine and a tyrosine-restricted diet, corrects liver failure in more than 90% of cases, abolishes porphyria-like crises, and improves renal and liver function. Treatment following early diagnosis at newborn screening results in normal liver function and structure until at least adolescence. The earlier the treatment is commenced, the lower is the risk of developing HCC, which has not been reported in those treated preemptively.
Current indications for LT include the following : ALF not responding to nitisinone (no improvement of coagulopathy or progressive jaundice after 1 week of treatment) or proven or suspected HCC 2 .
LT results in a lifelong functional cure, allowing a normal diet with improved quality of life. Renal production of SA persists following LT (see Figure 39.1 ), but this does not appear to have pathological consequences. SA production is not correlated with renal or liver function, and porphyria-like neurological crises have not been reported post-transplantation. Continued nitisinone treatment post-LT will abolish renal SA production, but at the cost of increased tyrosine levels, raising the issue of whether reintroduction of dietary restriction would be necessary. Thus this cannot be routinely recommended.

Category 2: Intrinsic Liver Disease With an Extrahepatic Defect
The indication for transplantation in this category will usually be as in category 1—that is, primarily based on the severity of the liver disease. However, in some disorders, the timing of transplantation may be affected by the extrahepatic defect, for which there may be a relatively short time window in which transplantation is feasible (e.g., in cystic fibrosis). The metabolic correction of the defect will be immediate and lifelong, but the ultimate outcome will reflect not just the outcome of transplantation but also the nature and progression of the extrahepatic disease. When counseling families, the fact that LT will not be “curative” should be highlighted. In this group of diseases, preemptive transplantation may not be appropriate, depending on the nature of the extrahepatic defect. A careful, individualized, multidisciplinary transplantation assessment process should be undertaken, and the outcome of transplantation in this group of disorders should be continuously audited.
Cystic Fibrosis–Related Liver Disease
Approximately 5% to 10% of young people with cystic fibrosis develop cirrhosis and portal hypertension, and some of them develop end-stage liver disease. LT has been shown to be very successful in selected cases, with excellent medium-term survival. Transplantation results in an initial stabilization of lung function and some slowing of the natural rate of deterioration in the first 4 years. In the longer term, respiratory deterioration continues to be the most common cause of death.
In cystic fibrosis, LT needs to be undertaken while there is sufficient pulmonary reserve to support the operation. Ideally, the FEV1 (forced expiratory volume over 1 second) should be greater than 50% predicted and certainly more than 40% predicted. Occasionally, preemptive LT, before the development of end-stage liver disease, may be appropriate if pulmonary function is deteriorating, as there is a risk of missing the window of opportunity. However, if LT is undertaken too early, end-stage liver disease may never have developed before the eventual onset of pulmonary insufficiency. In this situation, LT may not result in improved survival. However, the improved quality of life following successful LT also needs to be considered in the setting of this complex equation.
Mitochondrial Liver Diseases
Mitochondrial liver diseases may be the result of inherited disorders of respiratory chain proteins or because of abnormalities of mitochondrial DNA assembly. Unfortunately, these disorders are usually multisystemic, and when the presentation is with ALF, the outlook is very poor. LT for ALF resulting from a multisystemic defect does not prevent, and may even hasten, neurological deterioration. This is not just an individual tragedy but, in an era of organ shortage, results in the denial or delay of transplantation to someone else. The challenge is to exclude untreatable disorders as quickly and accurately as possible without denying lifesaving LT to those who may benefit.
Rapid and accurate characterization of the underlying defect is increasingly feasible, and more is known about the natural history of this group of disorders. LT is contraindicated when liver failure is because of valproate-induced ALF, in children who have pathogenic mutations in polymerase γ, Twinkle, or deoxyguanine kinase enzymes with neurological involvement. On the other hand, children presenting with chronic liver disease because of an isolated respiratory chain defect may have an excellent outcome following LT. Similarly, occasional children with mitochondrial DNA depletion owing to mutations in MPV-17 have good-quality, long-term survival, albeit often complicated by peripheral neuropathy. When the underlying defect in mitochondrial DNA cannot be defined, a systematic multidisciplinary assessment, including evaluation of extrahepatic involvement ( Fig. 39.2 ), is important so that individualized advice can be given to affected families. In general, the benefit of any genuine doubt should be given to the child.


Progressive Familial Intrahepatic Cholestasis Type 1
Progressive familial intrahepatic cholestasis type 1 (PFIC 1—FIC1 disease, Byler disease) is caused by a deficiency of FIC1, a P-type ATPase that is required for ATP-dependent amino phospholipid transport and encoded by the ATP8B1 gene. FIC1 is expressed in the liver, but also in the intestine and pancreas. FIC1 disease encompasses a continuum comprised of at least three disease phenotypes: (1) the severe Byler disease, which generally presents in infancy and leads to progressive cholestasis, often associated with severe pruritus; (2) benign recurrent intrahepatic cholestasis (BRIC) type 1, which gives rise to recurrent episodes of intrahepatic cholestasis, beginning in childhood or adulthood that can last days to months and that resolve spontaneously without causing detectable lasting liver damage; and (3) intrahepatic cholestasis of pregnancy (ICP) type 1, a transient cholestasis limited to pregnancy, with complete resolution after delivery, which also occurs in other cholestatic diseases. Systemic manifestations may include diarrhea, pancreatitis, short stature, and sensorineural deafness, suggesting a ubiquitous cell function for the FIC1 protein.
The diagnosis is usually suspected by normal or low serum GGT activity and cholesterol in the setting of cholestasis. Liver histology typically shows a bland canalicular cholestasis, with varying degrees of hepatocellular ballooning and giant cell transformation; portal fibrosis and eventually cirrhosis may be seen later in the course of the disease. The liver histology of patients with BRIC-1 and ICP-1 is typically normal.
Treatment options are similar to those for PFIC2 with a focus on symptomatic improvement in pruritus, optimization of nutritional status, and management of complications of chronic liver disease. External biliary diversion has been shown to be well tolerated and generally, although not uniformly, results in improvement of pruritus and cholestasis. However, the presence of cirrhosis at the time of diversion has been associated with poor outcomes, and recurrent, self-limited cholestasis episodes can occur. Without intervention, disease often progresses to end-stage liver disease, usually in the second decade of life. For these children, LT is lifesaving.
Despite excellent survival, the extrahepatic manifestations of the disease limit the benefit of transplantation. Diarrhea is usually worsened, catch-up growth is rare, and pancreatitis persists. A unique complication is the development of microvesicular steatohepatitis in approximately two-thirds of cases within months of transplantation. This is usually accompanied by progressive fibrosis and may lead to end-stage liver disease requiring retransplantation. Steatosis is also highly associated with those who develop post-transplantation diarrhea.
The mechanism of this complication is unclear but is presumably related to the interaction of normal bile from the allograft in an affected intestine with abnormal bile acid absorption. Interestingly, the recurrence of steatosis appears to be associated with the severity of the underlying mutation. Using bile acid sequestrants is logical but of uncertain benefit. Post-transplantation biliary diversion appears to have the potential to reverse steatosis and prevent fibrosis progression.
As a result, in severe PFIC1, alternatives to transplantation such as biliary diversion should be maximized and transplantation reserved for end-stage liver disease. Post-transplantation biliary diversion should be considered at an early stage if steatohepatitis develops.
Glycogen Storage Disease Type I
Glycogen storage disease (GSD) types Ia and Ib results from the deficiency of a two-component enzyme system involved in the transport of glucose 6-phosphate (Glu-6-P) from the cytosol into the endoplasmic reticulum (ER) by Glu-6-P translocase (encoded by the ubiquitously expressed SLC37A4 gene, GSD type Ib) and subsequent cleavage of Glu-6-P by glucose-6-phosphatase (Glu-6-Pase, encoded by the G6PC1 gene, GSD type Ia), located on the luminal side of the ER. The clinical phenotype with respect to liver disease is similar in the two forms; however, patients with GSD type Ib have intermittent severe neutropenia and neutrophil dysfunction, making them prone to recurrent episodes of severe bacterial infections and Crohn-like gastrointestinal disease. No correlation has been found between the severity of bacterial infections or degree of neutropenia and the molecular defect in humans with GSD type Ib, suggesting that other as yet unknown factors, such as modifying genes, define the ultimate disease phenotype.
In both disorders, LT immediately corrects the metabolic defect and reduces the frequency of hospitalization. Neutropenia persists in type Ib, albeit often milder, and granulocyte-colony stimulating factor (G-CSF) requirements decrease. In addition, the manifestations of inflammatory bowel disease usually decrease post-transplantation.
Category 3: No Intrinsic Liver Disease With Defect Confined to Liver
This category uses transplantation as a highly effective and realistic form of gene therapy. In individual disorders, the decision on LT should be assessed by a multidisciplinary team considering the success of transplantation, the prognosis of the underlying metabolic defect, the quality of life associated with treatment of the disease for the child and family, and the risk of irreversible disease in other organs, including kidney, brain, and heart.
In children without liver disease, the technical aspects of transplantation, especially removal of the native liver, are usually straightforward. However, these children have not had preexisting portal hypertension and are sensitive to the hemodynamic effects of portal vein clamping; as a result, they are more susceptible to early liver dysfunction and hepatic artery thrombosis. It follows that surgical expertise and selection of suitable, high-quality donor organs are particularly crucial to success. For this group of diseases, the transplantation results in a lifelong correction of the metabolic defect, and the long-term prognosis is similar to that of contemporary LT for other indications, unless irreversible disease-related complications have occurred.
Urea Cycle Disorders
Urea cycle defects (UCDs) are a result of defects in any one of six enzymes involved in the detoxification and elimination of ammonia. The clinical manifestations of UCD include encephalopathy attributed to hyperammonemia, although acute liver dysfunction is increasingly recognized. The most severe defects present in the newborn period and have a poor prognosis, with a high risk of severe neurological injury, even with prospective treatment. Those who present later have milder defects but are still at risk of suffering neurological sequelae.
LT for UCD results in a complete functional correction of the metabolic defect, allowing a normal diet, abolishing the risk of hyperammonemia, and transforming quality of life for affected children and their families. Because of the persistent intestinal defect, citrulline levels remain low in carbamoyl-phosphate synthetase (CPS) and ornithine transcarbamylase deficiencies (OTCDs) and high in arginine succinate synthase deficiency (citrullinemia). Low citrulline levels post-LT in CPS and OTCD have no obvious clinical consequences but, if treatment is thought necessary, they do respond to citrulline supplementation. Persistently elevated citrulline levels following LT in citrullinemia appear to have no clinical consequence.
There is a protection against further neurological damage, and there may even be some recovery of preexisting impairment, when transplantation is undertaken early, especially if carried out before 1 year of age.
LT should be considered early when there is severe disease and, ideally, before any neurological insult. The so-called honeymoon period before further acute decompensation is usually shorter than previously thought. As a result, transplantation should be planned after 3 months, which allows maximization of medical therapy and aggressive vaccination and avoids the higher technical risks of neonatal LT. For milder later-onset cases, the timing is less urgent. Each case should be reviewed on its merits, taking familial preferences into account.
Decision making in argininosuccinic aciduria (ASA) is more nuanced. Clinically, the metabolic defect is milder, but children frequently develop progressive liver disease. It is, however, rare for transplantation to be indicated solely because of the severity of liver disease during childhood. Another concern is that the enzyme is expressed in the brain, and hence the impact of LT on the neurological outcome is less predictable. However, the limited clinical experience with transplantation has been encouraging, with excellent outcomes to date.
Primary Hyperoxaluria Type 1
This condition is caused by a deficiency of hepatic alanine glyoxylate transaminases and shows considerable phenotypic variability. The defect causes overproduction of oxalate and results in dramatic nephrocalcinosis and urolithiasis, which may in turn cause renal insufficiency. As renal insufficiency progresses, and in particular as the glomerular filtration rate (GFR) falls to less than 40 mL/min/1.73 m 2 , oxalate starts to accumulate, setting the seeds of systemic oxalosis. Conventional dialysis is relatively inefficient at removing oxalate, and renal impairment marks the transition from a disease curable by LT into a multisystemic disease with long-term cardiovascular, ocular, renal, urological, and orthopedic manifestations. In the most severe phenotype, renal failure develops in infancy, and for the remainder, approximately 50% will develop end-stage renal disease during childhood.
LT completely corrects the primary biochemical defect and ideally should be undertaken electively when there is evidence of progressive renal disease, despite maximal medical treatment, but before the GFR falls below 40 to 50 mL/min/1.73m 2 . For this preemptive group, the medium-term outlook is excellent, with long-term preservation of native renal function, although some will eventually require renal transplantation. Even if they do require subsequent renal transplantation, they will avoid the morbidity associated with systemic oxalosis.
For those with established renal failure, a combined liver-kidney transplantation is required for survival. The presence of systemic oxalosis increases the immediate perioperative risks and, subsequent to transplantation, the huge accumulated oxalate load must be excreted via the grafted kidney. This is associated with a significant risk of recurrent renal oxalosis. In severely affected infants with renal failure, combined transplantation may be unrealistic. A staged procedure, with early LT to arrest oxalate, followed by renal transplantation when feasible (~ 10 kg), may be the only option. However, cardiovascular complications of systemic oxalosis may still develop following LT while on dialysis, which may preclude future renal transplantation and hence prove ultimately fatal.
Category 4: No Liver Disease and Extrahepatic Defect
The indications for liver transplantation are similar to those of category 3. The long-term outcome will be related not just to the success of transplantation but to the severity of the extrahepatic defect.
Organic Acidemias
The organic acidemias (OAs) are a group of severe disorders in which children are at risk from recurrent metabolic instability and face a significant cumulative risk of developing systemic complications, particularly neurological damage, but also including renal and cardiac disease, despite intrusive medical and nutritional support. These defects are expressed in the liver but also in the muscle and other organs. Although over 15 OAs have been identified, most experience with LT is with propionic acidemia (PA), methylmalonic acidemia (MMA), and maple syrup urine disease (MSUD).
PA and MMA are both autosomal recessive inborn errors of metabolism. PA is the result of mutations in the PCCA or PCCB genes encoding the alpha and beta subunits, respectively, of propionyl-CoA carboxylase (PCC). MMA is caused by complete or partial deficiency of MUT ( mut 0 enzymatic subtype or mut – enzymatic subtype, respectively), a defect in the transport or synthesis of its cofactor, adenosyl cobalamin ( cblA , cblB , or cblD -MMA), or deficiency of the enzyme methylmalonyl CoA epimerase.
For PA and MMA, the clinical presentation is that of the so-called intoxication type of neurological distress, a consequence of accumulating toxic compounds that are produced secondary to the metabolic block. Generally, following an initial symptom-free period ranging from hours to days after birth, affected neonates will present with a spectrum of symptoms, including food refusal, vomiting, progressive weight loss, generalized hypotonia, and abnormal posturing and movements. Progression to lethargy, seizures, and coma can occur, resulting in severe brain damage and death within a few days if not promptly treated. Biochemical and laboratory investigations reveal a combination of increased anion gap metabolic acidosis, leukopenia, thrombocytopenia, elevated lactate levels, anemia, ketonuria, and hyperammonemia. The diagnosis is made by elevated C3 carnitine levels in combination with specific urine and blood metabolites and confirmed by enzymatic or molecular studies.
Treatment strategies are reflective of the clinical state; they aim to address the disease-specific complications of the initial acute presentation, long-term management, and intermittent metabolic decompensations that can occur from various triggers. Ultimately, these management strategies have improved survival but have not modified the poor neurodevelopmental prognoses for children affected by these disorders. Other long-term complications include selective organ impairment from renal failure (MMA > PA), pancreatitis (PA > MMA), cardiomyopathy (PA > MMA), and brain basal ganglia infarctions (MMA > PA).
LT results in a clinically important partial correction of the metabolic defect in PA and MMA. Given that the enzymes responsible for PA and MMA are expressed in all tissues of the body, it is not expected that transplantation would provide a metabolic cure; rather, LT acts to stabilize metabolically fragile patients, minimizing the risk of further decompensations and improving quality of life. Early experience of transplantation was, however, associated with a high morbidity and mortality in young children, which may well have been, at least partly, an era effect. Recent experience has been much more positive, with long-term survival rates of greater than 80% and excellent quality of life; however, perioperative and long-term complications are common, highlighting the medical complexity of these diseases. Additionally, LT may provide additional societal benefit by increasing the quantity and quality of life years while decreasing cost over a patient’s lifetime. When children with PA and MMA are being considered for LT, it should be done relatively early because the risk of systemic complications is progressive.
MSUD is an OA caused by a deficiency in the branched-chain keto acid dehydrogenase enzyme complex (BCKDH). Neurotoxic accumulation of branched-chain amino acids (e.g., leucine, isoleucine, valine) and their intermediate metabolites (e.g., alpha-ketoisocaproic acid) leads to episodes of life-threatening ketoacidosis, cerebral edema, and brain damage. Treatment strategies primarily consist of dietary protein restriction to limit branched-chain amino acid consumption, combined with specialized formula supplementation to ensure adequate growth and development. Unfortunately, despite optimized dietary approaches, metabolic decompensations can occur often and require prompt treatment.
BCKDH activity is primarily located in the muscles (~ 60%), with hepatic activity being a minority (~ 9%–13%). Nevertheless, LT achieves a near-complete functional correction, with excellent control of amino acids, from dietary sources and/or muscle turnover. Conversely, because most BCKDH activity is extrahepatic, MSUD livers have been shown to be ideal candidates for domino liver transplantation donation. In contrast with PA and MMA, living related transplantation from a heterozygote donor, although effective, is associated with a residual risk of metabolic decompensation and should be avoided where possible.
Following transplantation for OA, management is often transformed, enabling dietary restriction to be minimized (PA and MMA) and/or eliminated (MSUD), and there is complete protection against metabolic crises. In MSUD, transplantation has been shown to prevent new neurological damage and improve neuroimaging appearances. In PA and MMA, disease-specific complications such as cardiomyopathy (PA) and kidney disease (MMA) can improve or stabilize.
S-Adenosylhomocysteine Hydrolase Deficiency
Methyl group donation is crucial to a wide range of cellular processes that are concentrated in the liver but are present in all tissues. S-Adenosylmethionine (SAM) is the universal methyl donor, forming S-adenosyl-homocysteine (SAH). This in turn is metabolized to homocysteine by S-adenosyl-homocysteine hydrolase (SAHH). SAHH deficiency (SAHHD) results in an abnormal SAM/SAH ratio, which inhibits transmethylation throughout the body. Clinically, this presents in infancy with a multisystemic disorder, including microcephaly, developmental delay, growth failure, and coagulopathy.
In a single-case report, LT resulted in resolution of the coagulopathy, a significant increase in hepatic methylation products, and improvement of the SAM/SAH ratio. This was accompanied by accelerated developmental progress, increased head growth, and an improvement in brain magnetic resonance imaging (MRI) appearance. This provided strong indirect evidence that not only was the hepatic defect corrected, but that normalization of the SAM/SAH ratio stimulated cerebral methyltransferase activity. This represents a novel way to cross the blood–brain barrier by using LT to change the systemic metabolic status, which may indirectly impact on cerebral intracellular processes.
Mitochondrial Disease With a Specific Enzyme Defect
LT has largely been ineffective in the treatment of systemic mitochondrial disorders of energy protection. In a rare group of mitochondrial disorders, in which a single enzyme defect results in accumulation of toxic intermediaries, successful LT may change the natural history of the disease.
Ethylmalonic Encephalopathy
This disorder is caused by a deficiency of the mitochondrial enzyme sulfur-dioxygenase, which results in the accumulation of gut-produced hydrogen sulfide and thiosulfate. This results in secondary mitochondrial dysfunction, with lactic acidosis, recurrent cerebral infarctions, seizures, and severe psychomotor delay. Antibiotics modify the disease somewhat, but affected children die before the age of 10 years. In a single-case report, living related LT at the age of 7 months had a dramatic biochemical defect. There was a rapid normalization of thiosulfate, combined with a significant improvement in plasma lactate and urinary methylmalonate levels. After 1-year follow-up, the infant was still globally delayed but showed a significant developmental catch-up, and no new brain MRI lesions developed.
Mitochondrial Neurogastrointestinal Encephalomyopathy
Mitochondrial neurogastrointestinal encephalomyopathy (MNGIE) is a fatal disease caused by thymidine phosphorylase deficiency resulting in a toxic accumulation of thymidine and deoxyuridine and causing secondary mitochondrial DNA depletion. The clinical phenotype encompasses severe gastrointestinal (GI) and neurological dysfunction. The GI involvement usually consists of chronic intestinal pseudo-obstruction, often requiring parenteral nutrition. Neurological involvement is more variable but may include ptosis, ophthalmoparesis, peripheral neuropathy, and myopathy. Those most severely affected present by the second decade of life and rarely survive beyond middle age. Hematopoietic stem cell transplantation has been shown to allow some functional recovery. However, the stem cell treatment carried a high mortality and, because thymidine phosphorylase is present in the liver, LT seems logical. In a single-case report, cadaveric LT was undertaken in a severely affected 25-year-old man who was immobile, severely undernourished, and parenteral nutrition- and G-CSF–dependent. Transplantation had a rapid biochemical effect, with normalization of plasma thymidine levels. At 1-year post-transplantation, he could walk, and his oral intake improved, but he remained dependent on parenteral nutrition. Repeat muscle biopsy showed a trend toward improved mitochondrial DNA copy numbers, while a brain MRI scan showed reduced cerebral lactate levels.
Domino Transplantation
When the recipient does not have intrinsic liver disease, there is a possibility of using the explanted liver for a domino transplantation. Here, the explanted liver is used to treat another recipient, with the expectation that the disease would not be clinically expressed or, if it is expressed, that it would be manageable and would not cause short-term clinical sequelae. Advantages include that the donor is hemodynamically stable, graft ischemia times are short, and there is no impact on the overall donor organ pool (see also Chapter 14 ).
However, the need to use the domino organ immediately after the original transplantation produces logistic difficulties. Thus this is better undertaken in a high-volume, well-resourced center, where parallel operating is feasible. Organs from patients with MSUD have been used successfully, even in pediatric recipients, with excellent long-term outcomes. The most common use of domino transplantation has been from donors with familial amyloid polyneuropathy. Although recipients express the disease, it takes many years to become clinically relevant, and this does not affect overall survival when used for older recipients. Domino organs from patients with familial hypercholesterolemia and methylmalonic acidemia have also been used successfully. However, using organs from patients with acute porphyria and oxalosis results in the rapid onset of severe disease in the recipient and should not be encouraged.
Living Donor Liver Transplantation
Access to cadaveric liver donation varies widely throughout the world, and its availability is the main driver for living donor liver transplantation (LDLT). LDLT has mainly used a left lobe donation from parent to child, although adult-to-adult donation is also very successful in experienced hands. The long-term outcome of LDLT is slightly superior to that of cadaveric transplantation, but the major advantage in unstable metabolic disease is that the procedure can be scheduled, ensuring that the recipient is in ideal condition (see also Chapter 8 ).
Most inherited metabolic diseases are autosomal recessive; hence parents are obligate heterozygotes and typically have 50% of normal enzyme activity. This does not seem to affect the risk to the donor parent or the efficacy of metabolic control in the recipient for most disorders.
In PiZ alpha-1 antitrypsin deficiency (A1ATD), the great majority of heterozygous parents will be clinically normal, but the heterozygous MZ state is a known cofactor for other chronic liver diseases. When cadaveric MZ donors have been used inadvertently, alpha-1 antitrypsin inclusions could be found on follow-up biopsies. These MZ living donors might be at greater risk from hepatic resection, and their recipient children might be receiving a less than ideal graft. However, these are theoretical concerns, and several live related transplants have been undertaken in A1ATD without reported adverse outcomes for donor or recipient.
In MSUD, because cadaveric transplantation only corrects approximately 10% of the defect, the use of an obligate heterozygous donor might result in an incomplete correction. However, when LDLT has been used, it has significantly ameliorated the disease, but with some residual risk of decompensation. Another example is the X-linked inherited disorder ornithine transcarbamylase deficiency. Although there is rarely a metabolic risk associated with a paternal donor, a maternal donor is very likely to be a carrier and might be partially affected. This would normally preclude maternal donation if there were alternatives, but the use of a partially affected donor results in significantly improved metabolic control as the recipient develops the phenotype of the donor.
Auxiliary Liver Transplantation
In auxiliary liver transplantation, not all of the recipient liver is removed, and a partial donor graft is placed orthotopically. This may be considered when there is no primary liver disease and a partial correction is likely to be effective. The major advantage of auxiliary liver transplantation is that the native liver is retained as a “safety net” if the graft fails or if gene therapy become available, whereupon immunosuppression could be withdrawn. The major disadvantage of the procedure is that the surgery is more complex, with the need to divert portal venous blood flow preferentially to the graft by a partial native portal vein banding (see Chapter 14 ). In experienced hands, this can be very effective, and long-term stable graft function can be achieved.
In practice, the use of auxiliary LT has largely been confined to Crigler-Najjar syndrome type 1 and to occasional cases of urea cycle defects or propionic acidemia. The description of the successful use of a domino graft from a patient with propionic acidemia as an auxiliary implant into a patient with Crigler-Najjar syndrome may extend the role of the technique. The recipient had effective clearance of bilirubin, without manifestations of propionic acidemia. This raises the possibility of patients with differing metabolic diseases undertaking partial liver exchanges without any impact on the donor pool.
References
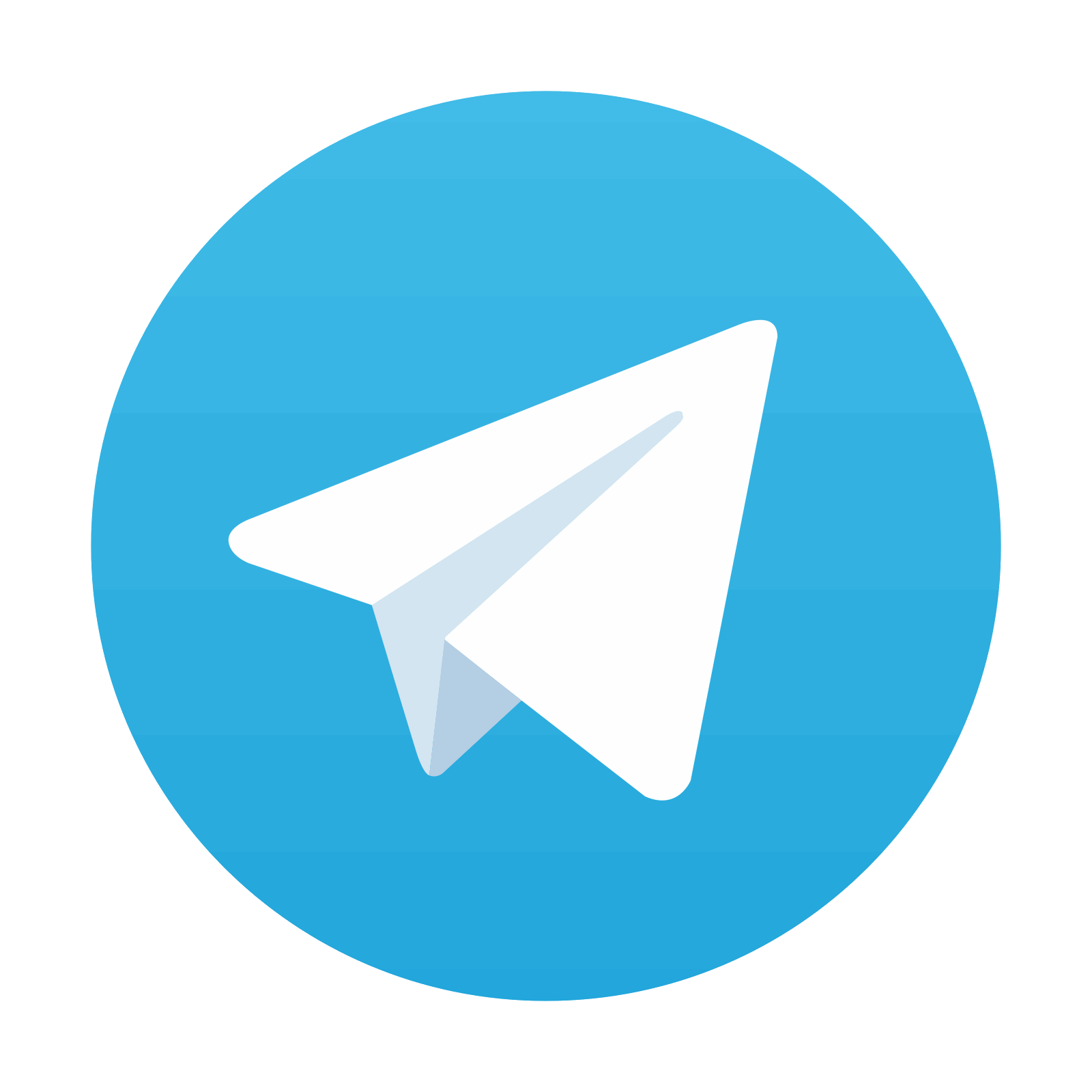
Stay updated, free articles. Join our Telegram channel

Full access? Get Clinical Tree
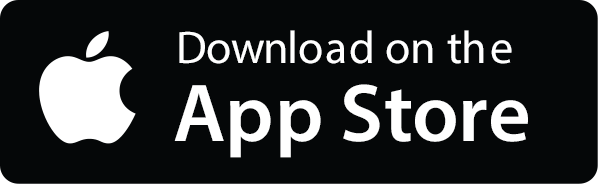
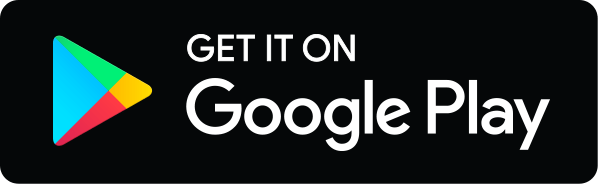
