Abstract
Metabolic complications are commonly encountered in urologic practice. To facilitate the diagnosis, management, and prevention of such complications, the urologist must maintain a firm understanding of the pathophysiology involved. This chapter provides an in-depth discussion of metabolic complications commonly encountered as sequelae of urologic surgery, including hyponatremia, transurethral resection (TUR) syndrome, postobstructive diuresis (POD), and metabolic complications of urinary diversion. Additionally, special consideration is given to the perioperative management of patients with chronic kidney disease (CKD).
Keywords
Chronic kidney disease, Hyponatremia, SIADH, TUR syndrome, Postobstructive diuresis, Urinary diversion, Electrolyte derangement
Chapter Outline
Perioperative Management of the Patient With Chronic Kidney Disease
Definition of Chronic Kidney Disease
Management of Comorbidities and Complications
Fluid and Electrolyte Considerations
Syndrome of Inappropriate Antidiuretic Hormone Secretion
Transurethral Resection Syndrome
Metabolic Complications of Urinary Diversion
[CR]
Key Points
- 1.
Unless the patient is severely symptomatic, correction of hyponatremia should proceed slowly so as to avoid the development of central pontine myelinolysis.
- 2.
The mechanism of transurethral resection (TUR) syndrome likely involves a combination of hyponatremia, hyperammonemia, and/or hyperglycinemia. Fluid resuscitation, possibly with hypertonic saline (3% NaCl), is appropriate.
- 3.
Brisk diuresis (>200 mL/hr) following the relief of urinary obstruction constitutes postobstructive diuresis (POD). Patients with POD should be monitored and treated to ensure that volume depletion and electrolyte disturbance do not occur. Most often, however, the diuresis is physiologic and will cease spontaneously without significant sequelae.
- 4.
The frequency and severity of metabolic complications following urinary diversion depend on the segment of bowel used, surface area, contact time, urine composition, renal function, and liver function. Severe metabolic disturbance is most common with the use of jejunum. This segment should only be used if ileum or colon is either unavailable or inadvisable. Complications with continent urinary diversion can be minimized if this form of diversion is limited to patients with a serum creatinine <2.0 mg/dL and a normal urine protein concentration.
Perioperative Management of the Patient With Chronic Kidney Disease
In the United States, the prevalence of chronic kidney disease (CKD) is estimated to be 13% of adults over the age of 20 years and the lifetime risk of developing CKD is >50% for adults living more than 65 years. It is associated with numerous complications and comorbid conditions that together pose a definite morbidity and mortality risk. Fortunately, adverse outcomes from renal insufficiency can be delayed or prevented through appropriate monitoring and early treatment. As such, it is essential that urologists maintain a basic understanding of CKD, particularly as it applies to perioperative management.
Definition of Chronic Kidney Disease
In 2002 the Kidney Disease Outcome Quality Initiative (K/DOQI) defined CKD according to the presence of objective renal damage (e.g., proteinuria >300 mg/day) or a glomerular filtration rate (GFR) below 60 mL/min/1.73 m 2 for >3 months. GFR, the best measure of overall renal function, represents the product of the number of functioning nephrons and single-nephron GFR. GFR can be estimated using the Cockcroft-Gault equation for creatinine clearance. Normal reference levels of GFR vary with age, gender, and body size. A GFR of 60 mL/min/1.73 m 2 represents roughly half the normal adult GFR. The K/DOQI definition allows for the normal age-related decline in GFR (~1 mL/min/1.73 m 2 per year) that occurs after the age of 20 or 30 years.
Management of Comorbidities and Complications
The numerous complications and comorbid conditions associated with CKD may negatively impact patient outcomes independent of the renal dysfunction itself. Each deserves individual consideration and management so as to minimize the potential for adverse events in the perioperative period.
Diabetes mellitus and hypertension represent the leading causes of end-stage renal disease (ESRD). New-onset or worsening hypertension, secondary to hypervolemia and stimulation of the renin angiotensin system, also represents a common complication of CKD. Strict glycemic control and optimization of blood pressure are obvious goals in the perioperative period. Angiotensin-converting enzyme (ACE) inhibitors and angiotensin-receptor blockers (ARBs) are first-line antihypertensive agents used in this setting; their use is based on studies that demonstrate effective blood pressure control and reduced risk of progression to ESRD in both diabetic and nondiabetic CKD patients. Diuretics, followed by calcium channel or beta-blocking agents, may be added if blood pressure remains high despite ACE inhibitors or ARBs. Loop diuretics are preferred over thiazides if the GFR is <30 mL/min/1.73 m 2 .
Cardiovascular disease is a common complication of CKD. Relative to the general population, the cardiovascular risk is threefold higher among patients with mild renal insufficiency and 65-fold higher in patients with ESRD. Reasons for this include extraskeletal calcification (e.g., valvular, atherosclerosis) secondary to calcium-phosphorous dysregulation, ventricular hypertrophy secondary to chronic anemia, and congestive heart failure (CHF) exacerbated by hypervolemia, as well as hypertension, diabetes, and dyslipidemia. Patients with longstanding CKD or a history of myocardial ischemia warrant preoperative cardiac evaluation, particularly if invasive surgery is planned.
Anemia is common in patients with a GFR <60 mL/min/1.73 m 2 . It is typically normochromic normocytic and reflects insufficient production of erythropoietin. Most patients with CKD are symptomatic (e.g., fatigue) at hemoglobin values <11 g/dL. Chronic anemia may promote left ventricular hypertrophy, CHF, and myocardial ischemia if untreated. Supplemental iron and vitamins are usually ineffective, and repeated transfusion risks the development of immune sensitization, which may compromise future transplantation. Recombinant erythropoietin is recommended as primary treatment. Maintaining the serum hematocrit at 33% to 36% or hemoglobin at 11 to 12 g/dL has been shown to reduce cardiovascular mortality by 30%.
Patients with renal insufficiency may develop a bleeding diathesis late in the course of disease as demonstrated by a prolongation in bleeding time. The primary defect involves an abnormality in von Willebrand’s factor (vWF)-related platelet aggregation and platelet–vessel wall interaction. Measures to correct this include administration of cryoprecipitate, factor III, or DDAVP (1-deamino-8- d -arginine vasopressin), all of which raise circulating levels of vWF. Correction of anemia with either erythropoietin or red blood cell transfusion also may improve the bleeding profile. These strategies are recommended as both prophylaxis and treatment in uremic patients undergoing major surgery.
Fluid and Electrolyte Considerations
Most patients with CKD are able to maintain fluid and electrolyte homeostasis until the renal function decreases to a GFR of 15 mL/min/1.73 m 2 . Homeostasis occurs because an adaptive increase in both single nephron GFR and tubular function causes an increase in the fractional excretion of water and electrolytes. Such adaptation can only maintain homeostasis under the conditions of a normal diet, however. Extreme changes in diet, as may occur perioperatively, often lead to fluid and electrolyte imbalance.
Patients with CKD progressively lose the ability to both conserve and excrete sodium. When the GFR falls below 15 mL/min/1.73 m 2 , sodium excretion becomes relatively fixed and the kidneys are no longer able to compensate for changes in sodium intake and nonrenal sodium loss. At a GFR of 5 mL/min/1.73 m 2 , the excretion of 120 to 140 mEq of sodium per day allows for a daily sodium intake of 5 to 10 g. Dietary intake below 2 g per day risks sodium depletion, whereas intake above 10 g risks total body sodium excess. Sodium depletion secondary to nonrenal loss is not uncommon in the perioperative period. Risk factors for sodium depletion include nasogastric suction, vomiting, diarrhea, and fistula drainage. Perioperative diuretic therapy may worsen the situation by augmenting renal sodium excretion. Patients without risk factors for sodium depletion require 5 to 10 g of sodium per day (diet or intravenous [IV]) to maintain homeostasis, whereas those with established risk factors require additional supplementation. In the setting of severe peripheral (or pulmonary) edema or hypertension, sodium intake should be limited to 2 g per day in order to avoid further fluid overload.
As renal failure progresses, the kidneys also lose the ability to concentrate and dilute urine. The concentration defect results from an impairment in the medullary solute gradient, which often overshadows the dilution defect until renal failure is quite advanced. Typically, water loads are well tolerated until the GFR approaches 15 mL/min/1.73 m 2 . In conjunction with the inability to regulate sodium excretion, the concentration defect predisposes patients with CKD to dehydration. The solute load generated by the intake of 5 to 10 g of sodium per day results in an obligate urine volume of 1.5 to 2.0 L. Consideration of perioperative fluid replacement must take into account obligate urine output in addition to the customary loss that occurs through hemorrhage, drainage, or insensible routes. Failure to do so may result in severe dehydration and an acute worsening of renal function through inadequate renal perfusion (pre-renal failure). Fluid intake should be maintained at a minimum of 1.5 L per day unless the patient has ESRD, in which case intake should match urine output plus insensible losses.
Hyponatremia is common in patients with severe CKD due to the impaired excretion of free water (i.e., dilution defect). Fluid restriction to less than 1 L per day is generally sufficient.
Hyperkalemia is uncommon in CKD until the GFR falls below 25% of normal. Patients with severe CKD are unable to fully excrete the daily potassium load; thus they are exquisitely sensitive to changes in intake. Exogenous intake of potassium, be it through diet or medications, is the most common cause of hyperkalemia in CKD. As such, potassium restriction is an important component of therapy.
Preoperative Evaluation
Patients with CKD require a careful preoperative determination of overall health. The basic evaluation includes a careful history and physical examination, complete metabolic profile, coagulation times, electrocardiogram, and plain radiograph of the chest. The presence of comorbid or complicating conditions should be determined preoperatively and managed accordingly. A detailed list of prescribed medications should be made because polypharmacy is common. Medications that adversely affect renal function or whose clearance depends on adequate renal function may require substitution or a reduction in dose. Nephrology consultation is advised for both perioperative and long-term care if the GFR is below 30 mL/min/1.73 m 2 .
Hyponatermia
Hyponatremia is the most common electrolyte disturbance encountered in clinical practice. Mild hyponatremia defined as a serum sodium of 130–135 mEq/L is seen in nearly 30% of admitted patients while moderate to severe hyponatremia (<125-129 mEq.L) is seen in up to 7% of patients.
Etiology
Hyponatremia may be due to loss of total body sodium, dilution of sodium in serum, or a false lab test. True hyponatremia consists of a dilution of sodium by water leading to a decreased serum concentration of sodium. This results in a hypo-osmolar serum (<280 mOsm/kg water). Sodium may also be diluted due to elevated levels of glucose or mannitol. As glucose levels rise, water is drawn into the extracellular space by osmosis thereby diluting sodium without changing total body water (TBW) or total body sodium. The patient’s serum is hyperosmolar (>295 mOsm/kg water). A correction factor of 2.4 mEq/L of sodium per 100 mg/dL of glucose over 100 mg/dL can be used to correct for this dilution. Patients with hyponatremia but a normal serum osmolality (280 and 295 mOsm/kg water) have pseudohyponatremia. Normally, water and electrolytes account for 93% of serum volume, while fat and protein account for the other 7%. If a patient develops hyperlipidemia or hyperproteinemia to the point that water accounts for <80% of the serum volume, the concentration of sodium is under estimated. This occurs because standard lab equipment reports a value based on liter of serum not liter of water assuming that the serum is made up of 93% water. The machine samples the same volume of fluid but the increased lipids or protein means that less water and sodium is taken up so the concentration is underestimated.
True hyponatremia is organized into subcategories based on the patient’s fluid status: hypovolemic, euvolemic, and hypervolemic states. These can be further delineated based on urine sodium concentration (U Na ). See Table 5.1 for common etiologies of hyponatremia based on fluid status and urine sodium concentration.
Urinary Sodium Concentration | Hypervolemic States | Euvolemic States | Hypovolemic States |
---|---|---|---|
Urine sodium >20 mEq/l | Renal failure | Addison’s disease (glucocorticoid) SIADH | Jejunal conduit Diuretic overuse Renal salt wasting (RTA) Nephrogenic diabetes insipidus Mineralocorticoid insufficiency |
Urine sodium <20 mEq/l | Congestive heart failure Hypoalbuminemia (cirrhosis, nephrotic syndrome) | Psychogenic diabetes insipidus | Excessive fluid losses (GI losses, heat stroke) |
Presentation
Mild hyponatremia is commonly asymptomatic. Moderate or severe hyponatremia can present with headaches, nausea, vomiting, muscle cramps, lethargy, seizures, and coma secondary to cerebral edema. To prevent excess swelling, the brain adjusts intracellular solutes to accommodate for serum electrolyte changes. In acute settings, cells do not have time to adapt so the swelling and symptoms are more pronounced. When onset of hyponatremia is slow, the brain is able to adapt to prevent swelling and minimize central nervous system symptoms.
Management
Management of hyponatremia is based on the acuity of onset and severity of symptoms. Rapid correction of hyponatremia can result in osmotic demyelination syndrome (ODS), previously called central pontine myelinolysis. This devastating neurologic complication is usually reported in the context of chronic hyponatremia; however, it also may occur following the overly rapid correction of acute hyponatremia. Unless the patient is severely symptomatic, correction of hyponatremia should proceed slowly to avoid ODS.
For patients with acute onset, severely symptomatic hyponatremia, a 100 mL bolus of 3% NaCl over 10 minutes may be used up to three times until symptoms subside. The sodium load of 100 mL of 3% Na is equal to 51 mEq Na. For milder acute cases, infusions of 3% NaCl at 0.5–2 mL/kg/hr may be used. Central venous access is recommended for the administration of continuous hypertonic saline; however, in emergency situations 3% NaCl can be given through a large bore peripheral IV without risk of a limb- or life-threatening event.
For patients with chronic hyponatremia, the management is based on a slower controlled rate of correction. In asymptomatic mild hyponatremia, the first line of treatment is fluid restriction. If this is ineffective, salt tabs or loop diuretics may be added. In mildly symptomatic hyponatremia, serum Na should be corrected at a rate of 1 mEq/L/hr. The 24 hour goal is to increase serum Na by at least 4–8 mEq/day but to not exceed 10–12 mEq/L in any 24-hour period. Additionally, an increase in Na greater than 18 mEq/L in any 48-hour period should be avoided. In contrast, patients with chronic hyponatremia and severe neurologic symptoms (e.g., seizures, coma) require rapid correction to a serum sodium level of 120 mEq/L over 1 to 2 hours, or until symptoms regress.
Guidelines for hypertonic saline administration are provided in Table 5.2 . Calculation of the sodium deficit may serve to guide replacement. Serum electrolytes (sodium) should be monitored frequently to guide treatment every 1 to 2 hours, initially.
Treatment Level | Indication | Sodium Solution | Dosage | Duration |
---|---|---|---|---|
1 | Asymptomatic plus serum Na >120 mEq/L | 0.9% NaCl | Based on Na + deficit | Until serum Na normal |
2 | Serum Na <120 mEq/L or symptomatic | 3% NaCl | 1–2 mL/kg/hr | Until symptoms improve |
3 | Severe symptoms | 3% NaCl | 4–5 mL/kg/hr | 1–2 hr |
Notes | ||||
Administer hypertonic saline via central venous catheter is preferred route for continuous replacement | ||||
If furosemide is added, it may double the rate of SNa rise | ||||
Measure SNa q 1–2 hours in early stages | ||||
Regress through levels of treatment as SNa and symptoms improve |
Syndrome of Inappropriate Antidiuretic Hormone Secretion
Etiology
Syndrome of inappropriate antidiuretic hormone secretion (SIADH) is a euvolemic form of hyponatremia due to excess antidiuretic hormone (ADH), also called vasopressin. In normal physiology, endogenous vasopressin is released from the posterior pituitary in response to increasing serum osmolality to increase free water absorption. In pathologic states, abundant release of vasopressin may be the response to CNS disturbances (stroke, trauma, infection), drugs (antiepileptics, chemotherapeutics, antipsychotics, opioids, Ecstasy), pulmonary disease (asthma, pneumonia, atelectasis), and hormone deficiencies (hypothyroidism, hypopituitarism). Extra-pituitary sources of vasopressin that may also cause SIADH include malignancies producing ADH (small cell carcinoma [SCC] of the lung, extra-pulmonary SCC, head and neck cancer, olfactory neuroblastoma) or drug administration of vasopressin, desmopressin, or oxytocin.
Mechanism
Vasopressin activates V 2 receptors in the collecting duct of the kidney to increase free water uptake. Activated V 2 increases cAMP via adenyl cyclase, which causes the transportation of intracellular vesicles containing aquaporin-2 to the apical plasma membrane along with up-regulation of aquaporin-2 synthesis. Aquaporins allow water reabsorption through the plasma membrane of the collecting duct. Total body sodium stays the same but TBW is increased, resulting in hyponatremia.
Presentation
SIADH presents similarly to other forms of hyponatremia ranging from asymptomatic to seizure and coma; however, it is important to note that these patients will be clinically euvolemic.
Evaluation
In 1967 Bartter and Schwartz developed the criteria for diagnosis of SIADH which is still used today. These are listed in Box 5.1 .
- ▪
Hyponatremia (Na <135 mEq/L)
- ▪
Clinically euvolemic
- ▪
Elevated urinary sodium excretion (U Na >20 mmol/L)
- ▪
True plasma hypo-osmolality (P osm <275 mOsm/kg H 2 O)
- ▪
Inappropriate urinary concentration (U osm >100 mOsm/kg H 2 O)
- ▪
Normal renal function and absence of diuretic use
- ▪
Normal salt and water intake
- ▪
Absence of other etiologies of euvolemic hypo-osmolality: hypothyroidism, glucocorticoid deficiency
Na, serum sodium concentration; U Na , urine sodium concentration; P osm , plasma osmolality; U osm , urine osmolality
Management
The management algorithm of SIADH, as with other hyponatremia patients, is based on acuity and severity. For most patients this is a chronic problem requiring slow correction. Asymptomatic patients are initially treated with restriction of fluids, not just free water. Thirsty patients may be offered hard candy or very small volumes of ice chips. Loop diuretics, not thiazides, may be added. Severely symptomatic patients may require hypertonic saline prior to other therapies.
Vasopressin receptor antagonists (vaptans) can be used as adjuvant treatment for SIADH. They prevent excess activation of V 2 receptors by competitively blocking the binding site of vasopressin. This results in diuresis even in the presence of excess vasopressin. Currently in the United States, the only two V 2 antagonists available are conivaptan (IV) and tolvaptan (oral). Both should be started in the inpatient setting, and use is at the discretion of the prescribing physician. These medications are not recommended as a single agent therapy but rather in combination with fluid restriction and sodium replacement.
Transurethral Resection Syndrome
Etiology
TUR syndrome is a constellation of signs and symptoms caused by the intravascular absorption of hypotonic fluids during endoscopic surgery. It is most commonly reported following transurethral resection of the prostate (TURP), but it may complicate any endoscopic procedure in which hypotonic irrigation is used, including cystoscopy, bladder tumor resection, percutaneous nephrolithotripsy, and transcervical endometrial resection. Intravascular absorption of irrigant occurs primarily through direct infusion into open venous channels; however, reabsorption of extravasated fluid following inadvertent perforation of the prostatic capsule or bladder wall also may occur. This distinction is important because TUR syndrome secondary to direct intravascular absorption presents acutely, whereas TUR syndrome associated with extravasation may be delayed up to 24 hours.
Early reports of TUR syndrome noted reddish discoloration of the serum, progressive oliguria, azotemia, pulmonary edema, and even death following the absorption of large amounts of sterile water during TURP. In initial reports of TUR syndrome, rapid water absorption resulted in severe hemolysis and the resulting sequelae. Nonelectrolyte solutions were subsequently utilized with osmolalities similar to serum (275–290 mOsm/kg H 2 O). These solutions included glycine, mannitol, and sorbitol. Although the risk of hemolysis with such solutions is negligible, TUR syndrome remains a concern, albeit less common, because while using monopolar electrocautery, modern irrigants are hypo-osmolar relative to serum (1.5% glycine = 200 mOsm/kg). The incidence of TUR syndrome among patients undergoing TURP with glycine is 1% to 10%. Mortality ranges from 0.2% to 0.8%.
In recent years, alternatives to monopolar TURP have become increasingly popular for the management of benign prostatic hyperplasia (BPH). These include bipolar electrocautery, green light vaporization, and holmium laser enucleation of the prostate. Standard TURP uses monopolar electrocautery, which requires a nonionic fluid to prevent conduction though the irrigant. Alternatively, newer methods utilize normal saline as they do not require electrical conduction through the patient to work. While fluid overload from absorption of fluid is possible, TUR syndrome has not been reported postoperatively in patients undergoing these procedures.
Mechanism
The mechanism of TUR syndrome is not universally agreed upon. Leading theories include the following:
- 1.
Intravascular fluid absorption
- 2.
Hyponatremia
- 3.
Hyperammonemia
- 4.
Hyperglycinemia.
Although hyponatremia is the primary cause of TUR syndrome, multiple factors may be responsible resulting in a heterogeneous presentation.
It is estimated that 20 mL of irrigation fluid is absorbed per minute of resection during TURP. Rapid or prolonged intravascular absorption causes a transient hypervolemia as demonstrated by an initial rise in central venous pressure (CVP), which plateaus within 15 minutes. Hypertension and reflex bradycardia are common early; however, as the syndrome progresses, a hypokinetic hemodynamic phase characterized by hypotension and bradycardia often develops. This hemodynamic shift reflects a decline in both cardiac output and intravascular volume, which begin once irrigation is discontinued. Factors responsible include natriuresis, osmotic diuresis, intracellular uptake of water, hyponatremia, hypocalcemia, and hypothermia among others.
Hyponatremia results from (1) expansion of the extracellular compartment by the infusion of hypotonic fluid (i.e., dilutional hyponatremia), and (2) increase in the urinary excretion of sodium (i.e., natriuresis). The relative contribution of dilution and natriuresis toward hyponatremia depends on the amount of irrigation absorbed and the timing of serum sodium analysis. Early in the course of absorption, sodium dilution predominates because the majority of fluid remains extracellular. As the osmotic gradient widens, water is progressively drawn into the intracellular space such that hyponatremia reaches its nadir when hypotonic irrigation is stopped. Less than 50% of the absorbed fluid remains extracellularly 30 minutes after infusion has ended.
Serum sodium is commonly used to quantify extracellular fluid (ECF) volume expansion; however, it is only at the completion of surgery that such an estimate is accurate. The degree of hyponatremia is an inaccurate measure of extracellular hydration at all other points in the postoperative period. Cases of irrigant extravasation represent an exception to this rule because hyponatremia in this setting is most pronounced 2 to 4 hours after surgery due to delayed reabsorption. The systemic absorption of glycine also stimulates renal sodium excretion.
Severe acute hyponatremia secondary to TUR syndrome presents similarly to other forms of acute hyponatremia. Cerebral edema leads to nausea, vomiting, confusion, and even seizures and coma if not treated promptly. Hypertension and bradycardia (i.e., Cushing reflex) also may arise as a consequence of heightened intracranial pressure. Radiologic studies demonstrate that cerebral edema may occur following the absorption of as little as 1 L of hypotonic irrigation. By comparison, irrigation with normal saline causes a greater expansion of the intravascular space, but cerebral edema is uncommon.
The most common irrigant used during TURP today is 1.5% glycine. Independent of volume and electrolyte imbalance, glycine and its metabolites retain properties that directly contribute to TUR syndrome in a dose-dependent manner. Glycine is metabolized by the liver into ammonia and glyoxylic acid. A small proportion (10%) is excreted unchanged by the kidneys and promotes an osmotic diuresis and natriuresis. Within the retina and central nervous system, glycine functions as an inhibitory neurotransmitter. Visual disturbance is reported in 10% of patients absorbing more than 500 mL of 1.5% glycine solution. With continued absorption, this may progress to transient blindness, which generally resolves within 24 hours and requires no specific treatment. Depending on the volume of glycine absorbed, a spectrum of mental status changes may be noted. The spectrum includes confusion, depressed consciousness, and coma. Glycine also impairs cardiac conduction and contractility through the generation of subendocardial hypoxic lesions.
Ammonia represents an intermediate product in the hepatic metabolism of glycine. Normally, ammonia is further metabolized by the liver into urea, which is then excreted by the kidneys. In cases of renal or hepatic insufficiency, however, the metabolism of ammonia is impaired and hyperammonemic encephalopathy may occur. Serum ammonia levels tend to correlate with the incidence and severity of neurologic symptoms. Hyperammonemic encephalopathy secondary to glycine absorption is self-limited and will gradually correct once the infusion is stopped. Only supportive measures need be provided.
Risk Factors
Potential risk factors for the development of TUR syndrome are listed in Box 5.2 . Although the extent of resection correlates with the volume of irrigation absorbed and the incidence of TUR syndrome, the relationship between TUR syndrome and irrigation pressure is not as precise. Two large studies have failed to demonstrate any correlation between bag height and fluid absorption during TURP. Smoking has been shown to be an independent predictor of large-scale fluid absorption (>1 L) in patients undergoing TURP. This is thought to reflect an increase in prostatic micro-vessel density among smokers.
- ▪
Extensive resection
- ▪
>45 grams
- ▪
>90 minutes
- ▪
Open venous sinus
- ▪
Capsular perforation
- ▪
High pressure irrigation
- ▪
>40 cm H 2 O
- ▪
Intermittent flow resection
- ▪
Smoking history
Presentation
The presentation of TUR syndrome is heterogeneous and depends on the volume, rate, route, and type of fluid absorbed. Mild cases usually present 30 to 45 minutes after the completion of surgery and may be overlooked. Symptoms are usually neurologic or cardiovascular in origin ( Table 5.3 ). During the intraoperative and early postoperative period, nausea, vomiting, restlessness, confusion, and visual disturbance are common as are the sensations of facial prickling and warmth. Blindness, seizures, and coma may occur in extreme cases. Although transient hypertension and bradycardia can be seen initially, hypotension secondary to gradual ECF volume contraction is more common and problematic. Pulmonary edema may develop in patients with left ventricular dysfunction. In the event of extravasation, abdominal pain radiating to the shoulder is common.
Cardiovascular | Neurologic |
---|---|
Bradycardia | Nausea |
Hypertension | Vomiting |
Hypotension | Restlessness |
Shortness of breath | Confusion |
Chest pain | Depressed consciousness |
Headache | |
Blurred vision | |
Blindness |
Evaluation
Suspicion of TUR syndrome intraoperatively warrants prompt termination of the procedure after hemostasis is attained. Serum sodium and osmolality should be measured at the completion of surgery because it is only at this time that they accurately quantify ECF volume expansion. Most patients are not symptomatic until the serum sodium concentration falls to <125 mEq/dL.
Supplementary measures of fluid absorption include volumetric fluid balance (Volume irrigation − Volume recovered = Volume absorbed), patient weight, CVP, and ethanol breath measurement; however, these are not in widespread clinical use. In addition to laboratory evaluation, hemodynamic stability and intravascular volume status should be determined. This may involve central venous or pulmonary capillary wedge pressure monitoring in unstable patients.
Management
The treatment of TUR syndrome must address both volume status and hyponatremia. On one hand, hypertension secondary to volume overload is usually transient and will resolve spontaneously as the osmotic diuresis and intracellular transfer of fluid proceed. Cardiovascular collapse, on the other hand, requires urgent treatment. Unstable patients with profound hypotension and bradycardia are treated with atropine, epinephrine, and IV calcium.
Fluid restriction and loop diuretics can be used to correct volume overload and hyponatremia; however, plasma volume expansion and sodium supplementation should be the goal. This can be achieved using IV normal saline (0.9% NaCl) in patients with mild symptoms and serum sodium >130 mEq/L or, in severe cases, hypertonic saline. The primary indication for use of loop diuretics is acute symptomatic pulmonary edema that fails to resolve with spontaneous diuresis. The combination of hypertonic saline and furosemide may be appropriate in severe cases (e.g., seizure, obtundation, or coma) wherein rapid correction of hyponatremia is necessary. The addition of furosemide (20–40 mg) in this manner accelerates the rise in serum sodium twofold over hypertonic saline alone.
Postobstructive Diuresis
POD refers to the dramatic urine output that may occur following the relief of bilateral ureteral obstruction (BUO) or obstruction of a solitary kidney. The true incidence of POD is not known; however, it does appear to be an uncommon event. Factors necessary for its development include an accumulation of TBW, sodium, and urea or an impairment of tubular or collecting duct reabsorptive capabilities. Most cases of POD involve chronic urinary obstruction; however, significant changes in glomerular and tubular function are evident within 24 hours of complete ureteral obstruction. Although ipsilateral renal function is compromised in all cases of ureteral obstruction, clinically significant POD is uncommon in patients with a normal contralateral kidney. This reflects the continued maintenance of fluid and electrolyte homeostasis by the contralateral kidney. For the purpose of this review, only the pathophysiology of BUO (or obstruction of a solitary kidney) as it pertains to obstructive uropathy and POD is discussed.
Etiology
Urinary obstruction may be anatomic or functional (e.g., neurogenic bladder) in etiology and intrinsic or extrinsic in location. A multitude of etiologies exist, the most common of which include BPH or prostate cancer in males and cervical cancer or pregnancy in females. Although nephrolithiasis is a relatively frequent cause of acute obstruction, most cases of POD involve chronic and progressive urinary obstruction as may occur with bladder outlet obstruction (e.g., BPH) or extrinsic bilateral ureteral obstruction (e.g., cervical cancer).
Pathophysiology of Obstructive Nephropathy
Obstructive nephropathy refers to the functional and pathologic damage sustained by the renal parenchyma during urinary obstruction. The severity and consequences thereof depend on the degree and duration of obstruction as well as the presence or absence of an unobstructed contralateral kidney. Our present understanding of the involved pathophysiology comes mainly from animal models of complete unilateral or bilateral ureteral obstruction for 24 hours or more.
Upon the initiation of complete obstruction, profound changes in renal hemodynamics and glomerular filtration take place. In the case of BUO, such changes follow a biphasic pattern. Within the first 2 hours of obstruction, GFR begins to fall as a consequence of elevated tubular hydraulic pressure (P T ). Beyond this period, P T continues to rise and, more importantly, renal blood flow (RBF) falls dramatically as a result of intense efferent arteriolar vasoconstriction caused by angiotensin II, thromboxane A 2 , and neural input. Intrarenal blood flow is also preferentially directed toward the outer cortex in BUO, leaving many glomeruli nonperfused.
The end result is a profound reduction in single nephron and whole kidney GFR by 24 hours. Animal models report ipsilateral GFR at roughly 25% of normal following 1 week of complete unilateral ureteral obstruction. Glomerular filtration appears to be better maintained in BUO, likely on the basis of elevated levels of atrial natriuretic peptide (ANP). Secreted in response to intravascular volume expansion, ANP improves RBF and GFR through the following mechanisms:
- 1.
Afferent arteriolar vasodilation
- 2.
Tubulo-glomerular feedback inhibition
- 3.
Renin-angiotensin system (vasoconstrictive) inhibition
- 4.
Glomerular filtration coefficient (Kf) increase.
Along with its direct natriuretic properties, the actions of ANP are thought to contribute to the diuresis, natriuresis, and rapid return to maximal renal function observed following the relief of BUO. Persistent obstruction also exerts deleterious effects on renal tubular function, primarily through the down-regulation of important transporter and co-transporter activity along the entire nephron. This impairs the reabsorption of sodium (Na + ), potassium (K + ), hydrogen (H + ), and water, which, in turn, dissipates the medullary solute gradient and limits the generation of concentrated urine. Together, glomerular and tubular dysfunction manifest as profound natriuresis and diuresis following the relief of BUO.
Mechanism
POD may involve a water diuresis, a solute diuresis, or a combination thereof. In most cases, POD represents an appropriate and self-limited physiologic response to volume and solute overload. On occasion, however, the diuresis may extend inappropriately beyond the euvolemic state, usually as a result of excessive free-water loss caused by collecting duct insensitivity to antidiuretic hormone (i.e., nephrogenic diabetes insipidus). Similar to physiologic POD, this form of diuresis is often self-limited and easily managed. True pathologic POD usually refers to the inappropriate excretion of both water and solute as reflected by a urine osmolality >250 mOsm/kg water ( Table 5.4 ). The inability to concentrate urine in pathologic POD stems from the defective distal tubular sodium reabsorption and loss of the medullary solute gradient. Reasons for this include the following:
- 1.
Decreased reabsorption of sodium chloride in the loop of Henle and distal tubule
- 2.
Decreased reabsorption of urea in the collecting tubule
- 3.
Medullary solute washout caused by increased medullary blood flow
- 4.
Increased tubular flow rate and solute concentration in the distal tubule.
Diuresis | Uosm (mOsm/kg Water) | Uosm : Posm |
---|---|---|
Pure water | <150 | <0.9 |
Solute | ≥250 | >0.9 |
Mixed | ≥250 | <0.9 |
Presentation
The manner of presentation of urinary obstruction depends on the etiology, the time over which it develops, whether it is unilateral or bilateral, and whether it is complete or partial. In the context of chronic BUO, the signs and symptoms are often nonspecific. Although most patients with complete obstruction describe anuria or oliguria, some patients with partial obstruction may report polyuria secondary to impaired renal concentrating ability.
Volume expansion is common and may present as weight gain, peripheral edema, or even shortness of breath if CHF is a complicating factor. Patients with long-standing obstruction may present with uremic symptoms including mental status change, tremor, and gastrointestinal (GI) bleeding. Volume expansion or azotemia in the setting of BUO should raise suspicion for possible diuresis following the relief of obstruction. As such, these patients should be monitored and treated accordingly. In clinical practice, a urine output in excess of 200 mL/hr over 12 consecutive hours constitutes POD. It is important to note, however, that significant derangement in fluid and electrolyte status can take place during the initial 12 hours and, as such, appropriate investigation and treatment should not be delayed.
Evaluation and Management
Upon presenting with BUO, patients should undergo a thorough baseline evaluation including a complete blood count (CBC) and complete metabolic profile. Elevations in serum blood urea nitrogen (BUN) and creatinine may be identified as well as hyperkalemia and metabolic acidosis, which require correction. The development of a brisk diuresis (>200 mL/hr) following the relief of obstruction constitutes POD and warrants appropriate monitoring, investigation, and, when appropriate, treatment.
Most cases represent physiologic POD and, as such, the diuresis is allowed to proceed until the euvolemic state is reached as determined by clinical parameters such as orthostatic vital signs, breath sounds, jugular venous distention, and peripheral edema. During this time, serum electrolytes should be evaluated every 6 to 12 hours because electrolyte imbalance, particularly hypokalemia and hypomagnesemia, may develop.
If the diuresis persists beyond the euvolemic state, a pathologic concentrating defect or salt wasting nephropathy should be suspected. In this case, urinary diagnostic indices are useful to determine the type of diuresis (water versus solute versus mixed) and to guide fluid replacement (see Table 5.4 ). With excessive free water loss, plasma osmolality will rise while urine osmolality remains inappropriately low (<150 mOsm/kg water).
Most patients with an intact thirst mechanism and free access to water can compensate for this defect. Patients in whom oral replacement is not an option because of mental status, nil per os (NPO) status, or limited access to water require IV supplementation with hypotonic saline solutions (0.45% NaCl).
Most recommendations call for the replacement of half the urine output at 2 hourly intervals so as to not perpetuate the diuresis. However, animal models suggest that maintenance of a volume-expanded state may improve ultimate renal recovery. This has led some centers to replace the entire urine output until renal function plateaus. Contraindications to excessive hydration include CHF and hypertensive crisis. Fluid replacement may be withheld in these cases until such time that the heart failure or hypertension resolves with diuresis and appropriate medical management.
Pathologic POD secondary to salt-wasting nephropathy is a rare, yet potentially life-threatening, event. Dehydration and electrolyte imbalance (Na + , K + , magnesium [Mg 2+ ]) are common as the persistent natriuresis promotes the loss of water, potassium, and magnesium. Urine osmolality (≥250 mOsm/kg water) is often slightly higher than that of plasma. These patients warrant careful hemodynamic monitoring, possibly including CVP, as well as frequent monitoring of both serum and urine electrolytes.
Sodium and volume replacement is accomplished using normal saline (0.9% NaCl) supplemented on occasion with hypertonic saline (3% NaCl) if the sodium deficit is profound. Serum and urine electrolytes serve as a guide in this regard. Volume replacement should match urine output in a 1 : 1 fashion until vital signs and renal function stabilize. Hypokalemia and hypomagnesemia are common and require correction. Hyperkalemia and acidosis also may occur, particularly in the event of profound dehydration and consequent worsening of renal function.
Metabolic Complications of Urinary Diversion
Metabolic complications are common to all forms of bowel urinary diversion as a result of continued solute transport by the interposed segment. These include electrolyte and acid–base abnormalities, altered sensorium, drug toxicity, osteomalacia, urinary calculi, and GI malabsorption syndromes. Factors that influence the type and severity of such complications include the following:
- 1.
Segment of bowel used
- 2.
Surface area (length of bowel)
- 3.
Contact time with urine
- 4.
Urine composition (solutes, pH)
- 5.
Renal function.
These factors are particularly important with regard to the development of electrolyte and acid–base disturbance, the focus of this discussion.
Gastrointestinal Transport of Water and Electrolytes
A basic understanding of GI transport facilitates the management of potential complications. The primary functions of the GI tract are digestion, absorption of water and nutrients, and excretion of solid waste. Water and electrolyte transport occurs through the paracellular and transcellular pathways.
A Na + /K + ATPase located on the basolateral membrane drives the transport of water and solute across the intestinal mucosa by generating an electrochemical gradient. This gradient favors the absorption of sodium through luminal Na + /H + exchange or noncoupled Na + channels. Variable expression of luminal transport proteins helps to explain differences in the type, incidence, and severity of electrolyte abnormalities amongst the different bowel segments used for urinary reconstruction. For example, the jejunum has very loose intracellular junctions, allowing for the rapid movement of fluid and electrolytes through paracellular pathways. It also lacks a Na + /H + antiporter but has a Cl − /HCO 3 − antiporter, which allows for movement of bicarbonate and chloride.
Sodium rapidly follows the existing concentration gradient such as that between plasma (140 mEq/L) and urine (20–40 mEq/L). The frequent association of hyponatremia and hypovolemia with jejunal conduits reflects this fact. Chloride absorption is linked to bicarbonate secretion throughout the small bowel and colon. Bicarbonate, generated by intracellular carbonic anhydrase, is secreted in a 1 : 1 ratio for chloride. In contrast, gastric parietal cells generate bicarbonate for systemic absorption in the process of acid (H + ) secretion in exchange for potassium. The ileum and colon have less permeable intracellular junctions and can exchange both Na + /H + and Cl − /HCO 3 − . For the most part, the intestinal transport of potassium is passive as dictated by the electrochemical gradient and movement of water. The luminal concentration of potassium is an important determinant of net potassium secretion or absorption.
The majority of water transport and a minority of solute transport occurs paracellularly. GI osmotic permeability, as determined by mucosal tight junctions, decreases caudally such that the permeability of proximal small bowel is four to six times that of the distal ileum and colon. The heightened permeability of jejunum to both solute and water explains the frequent association of electrolyte abnormalities with use of this segment.
Stomach
The interposition of gastric mucosa in the urinary tract has been performed more commonly in pediatric patients than in adults. Purported advantages to the use of stomach include the prevention of short gut syndrome, less mucus production, a relative reduction in the risk of electrolyte or acid–base disturbance, recurrent infection, and urolithiasis. The most frequent electrolyte abnormality is a hypochloremic, hypokalemic metabolic alkalosis, which occurs as a result of hydrogen, chloride, and potassium secretion ( Table 5.5 ).
Segment | Electrolyte Abnormality | Treatment | Prevention |
---|---|---|---|
Stomach | Hyperchloremic, hypokalemic metabolic alkalosis |
|
|
Jejunum | Hyponatremic, hypochloremic, hyperkalemic metabolic acidosis |
|
|
Ileum or colon | Hyperchloremic metabolic acidosis ± Hypokalemia | Alkalinization (IV/PO) ± KCl replacement |
|
Patients are also at risk for dehydration secondary to the loss of water through the gastric mucosa. Rarely does this metabolic abnormality achieve clinical significance except in cases of severe renal dysfunction or acute dehydration. Although patients with normal renal function are able to minimize the development of alkalosis through an increase in renal bicarbonate excretion, those with renal insufficiency cannot.
Hypergastrinemia also may be important. Secreted by gastrin cells in response to distention (among other stimuli), gastrin stimulates proton secretion by parietal cells through activation of the luminal H + /K + ATPase. This mechanism becomes clinically relevant in cases of gastrocystoplasty wherein bladder distention caused by chronic outlet obstruction may stimulate the secretion of gastrin and precipitate this syndrome.
Symptomatic patients may present with lethargy, weakness, altered mental status, seizures, respiratory depression, and cardiac dysrhythmia. Treatment begins with rehydration using IV normal saline (0.9% NaCl) and correction of hypokalemia as outlined in Table 5.5 . Long-term supplementation with oral salt or potassium chloride also may be of benefit. Patients with persistent mild metabolic alkalosis warrant treatment with histamine-2 (H 2 ) receptor antagonists, which competitively inhibit histamine-mediated gastric acid secretion. Proton pump inhibitors, which directly inhibit the gastric H + /K + ATPase, are useful in cases refractory to H 2 blockade.
Jejunum
Of all bowel segments used for urinary reconstruction, electrolyte abnormalities occur most commonly with the use of jejunum. As a result, this segment is only used when ileum or colon are either unavailable or inadvisable. The typical metabolic changes that occur when jejunum is used include hyponatremia, hypochloremia, hyperkalemia, volume contraction, and metabolic acidosis, all of which reflect the propensity of jejunum for sodium and chloride secretion and potassium and hydrogen ion absorption (see Table 5.5 ). Significant dehydration is common because the osmotic gradient generated by sodium secretion results in the net loss of water and sodium. Hypovolemia stimulates the secretion of aldosterone, which, in turn, stimulates renal sodium reabsorption and potassium secretion. This produces a urine low in sodium and high in potassium. When exposed to such urine, jejunum loses sodium and absorbs potassium as driven by the electrochemical gradient. This further perpetuates the metabolic disturbance. At least 25% or more of patients may develop metabolic changes, although only 4% are severe if short segments are used. The incidence and severity of this syndrome also appears to be less if distal rather than proximal jejunum is used.
In severe cases, patients may present with lethargy, nausea, vomiting, dehydration, and weakness. Treatment involves rehydration with IV normal saline and correction of metabolic acidosis using sodium bicarbonate (NaHCO 3 ). In the acute setting, standard management of hyperkalemia includes the use of insulin or glucose infusion, bicarbonate infusion, and orally or rectally administered potassium-binding resins such as sodium polystyrene sulfonate (Kayexalate). In the normovolemic state, most patients are able to excrete excess potassium if renal function is normal. If this is not the case, thiazide diuretics may be used to augment the renal excretion of potassium in the long term. Likewise, vigilant oral hydration and sodium chloride supplementation are recommended as prophylactic strategies.
Ileum and Colon
Ileum and colon, the bowel segments used most commonly in urinary diversion, possess similar transport properties. When exposed to urine in which the concentration of potassium and hydrogen is typically higher and the concentration of sodium is lower than intestinal contents, these segments secrete sodium and bicarbonate and absorb hydrogen, chloride, and ammonium.
Ammonia, generated by the kidneys, buffers free urinary hydrogen ions through the formation of ammonium. Ammonium, in turn, competes with sodium for absorption through the intestinal Na + /H + antiporter, whereas bicarbonate is excreted in exchange for chloride absorption. The net result is a hyperchloremic metabolic acidosis. It appears that the absorption of ammonium chloride accounts for the majority of the acid load.
Hypokalemia and total-body potassium depletion also may occur. Most commonly, this reflects intracellular potassium depletion secondary to chronic metabolic acidosis in combination with renal potassium wasting and intestinal potassium secretion. Hypokalemia is less common with ileal versus colonic diversions because ileum demonstrates a higher capacity for potassium absorption than does colon.
Hyperchloremic metabolic acidosis is not uncommon following urinary reconstruction with ileum or colon (see Table 5.5 ). In patients that have diversions with ureterosigmoidostomies, although now uncommon, the severity and frequency of this complication are much higher. In most cases, however, the metabolic disturbance is quite mild because of renal and hepatic compensation. Metabolic acidosis is minimized through an increase in acid excretion by the kidneys, whereas the toxic effects of hyperammonemia are avoided through the hepatic metabolism of ammonium to urea. As discussed, the incidence and severity of metabolic disturbance depend on numerous factors including length of bowel segment (surface area), contact time with urine, and renal function. Commensurate with these risk factors, the incidence of hyperchloremic metabolic acidosis is higher with continent diversions (50%) than with conduit diversions (10–15%) using ileum or colon. This highlights the importance of normal renal and hepatic function at baseline in patients considered for continent urinary diversion. Basic requirements for continent diversion include a serum creatinine <2.0 mg/dL and a normal urine protein concentration. Patients with a GFR >35 mL/min and minimal urine protein who are able to generate a urine pH <5.8 following ammonium chloride loading and urine osmolality >600 mOsm/kg following water deprivation also may be suitable candidates. Otherwise, conduit urinary diversion is more appropriate. There are no clinically significant differences in metabolic derangements between continent diversion and orthotopic bladder replacement.
Patients with significant hyperchloremic metabolic acidosis may present with fatigue, weight loss, anorexia, and polydipsia. Laboratory studies reveal a significant nonanion gap metabolic acidosis with hypochloremia and azotemia. It is estimated that up to 10% of patients with conduit diversion and a higher proportion with continent diversion require treatment for chronic acidosis. Treatment involves alkalinization and simultaneous potassium replacement. Correction of acidosis without adequate potassium repletion may precipitate or worsen hypokalemia as potassium is driven into the intracellular space. Sodium bicarbonate is used most commonly for alkalinization (1–2 g TID), but patients may suffer from flatulence. Sodium citrate (1–3 g QID) is a suitable alternative, but it has an unpleasant taste. Unless potassium supplementation is contraindicated, potassium citrate may be more appropriate in patients with CHF or renal insufficiency in whom an excessive sodium load may be poorly tolerated. Nicotinic acid (500 mg to 2 g daily) or chlorpromazine (25 to 50 mg QID) has been reported to be useful in animal models, but lacks confirmatory data in humans.
Hypocalcemia may also be a problem for patients with urinary diversions. Bone carbonate is used to continuously buffer the chronic metabolic acidosis. Calcium carbonate is mobilized from the bone to supply the buffer. Patients may require calcium supplementation daily (500 mg to 1 g). In addition, patients may experience profound drops in serum phosphorus upon rapid correction of the chronic acidosis as bone stores of calcium phosphate are replenished.
Specific management considerations in patients with a continent urinary diversion are needed due to urine’s prolonged contact with the intestinal surface compared to conduit diversions. This includes the establishment of prompt drainage (i.e., indwelling catheter) as well as empiric antibiotic therapy if urinary tract infection (UTI) is suspected. Urinary obstruction perpetuates the transport of water and solute across the bowel segment, whereas systemic infection has been known to precipitate ammoniagenic encephalopathy through bacterial endotoxin-induced hepatic dysfunction.
Altered Sensorium
Among patients with urinary diversion or reconstruction, an altered sensorium may develop secondary to magnesium deficiency, drug toxicity, or ammoniagenic encephalopathy. Hypomagnesemia, although uncommon, reflects GI malabsorption or renal magnesium wasting caused by chronic acidosis. Drug intoxication may develop whenever a particular medication or its active metabolite is excreted unchanged by the kidneys and then absorbed systemically by interposed bowel. This may occur with such drugs as phenytoin or methotrexate, among others. Although chemotherapy (including methotrexate) has been found to be equally safe among patients with continent and incontinent diversions, it seems prudent to catheterize continent patients during therapy.
Excreted by the kidneys, ammonium is absorbed by the intestinal segment and transported to the liver via the portal circulation. Ammonium (or ammonia) is metabolized by the liver into urea, which is then excreted by the kidneys. Under normal conditions, hepatic metabolism is able to adapt to the increased delivery of portal ammonia such that systemic levels remain unchanged. In the setting of liver dysfunction, however, serum ammonia may accumulate to toxic proportions.
Ammoniagenic encephalopathy is a rare complication of bowel urinary diversion. Although this syndrome develops most commonly in patients with liver dysfunction, case reports also exist among patients with normal hepatic function. Often, such cases involve systemic bacterial infection or UTI with urea-splitting organisms. Systemic infection may induce transient hepatic dysfunction secondary to the proliferation of bacterial endotoxin. Alternately, urea-splitting bacteria may generate ammonia through the enzymatic action of urease on urea. In both cases, the absorbed ammonia load may overwhelm hepatic metabolism and precipitate encephalopathy.
Depending upon the degree of hyperammonemia and the time course over which it develops, symptoms of encephalopathy vary from mild changes in mental state to deep coma. Evaluation includes an assessment of liver function as well as a thorough search for possible systemic or UTI. In patients with continent urinary diversion, obstruction should be ruled out because urinary stasis may augment the absorption of urinary ammonium. Acute management includes prompt urinary drainage by way of Foley catheter or rectal tube and empiric systemic antibiotics tailored toward urea-splitting bacteria. Dietary protein restriction, neomycin (oral), and lactulose (oral or rectal), which reduce the production or absorption of ammonia in the GI tract, also are appropriate.
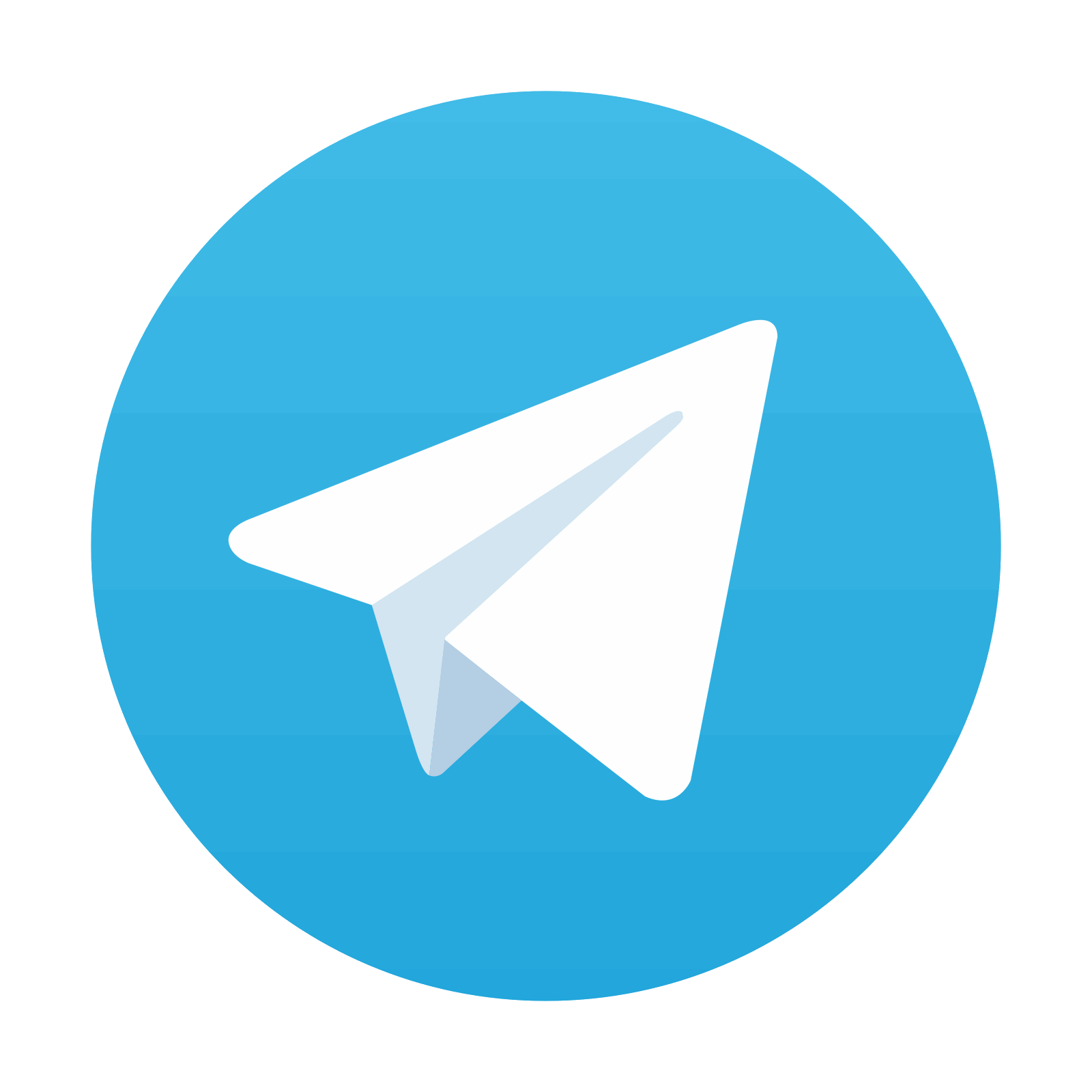
Stay updated, free articles. Join our Telegram channel

Full access? Get Clinical Tree
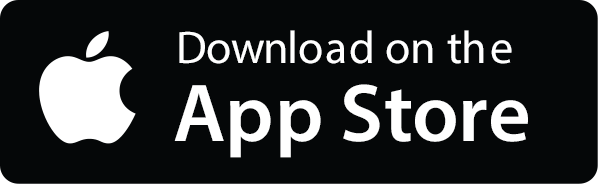
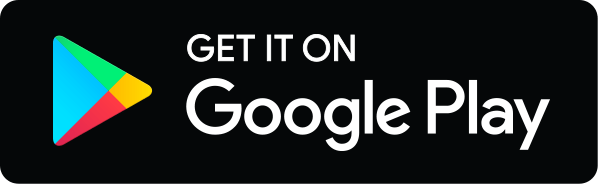