Case Study 1
A 15-year-old woman with a medical history of diabetes mellitus, obesity, compensated cirrhosis, and nonalcoholic steatohepatitis had right first distal phalanx osteomyelitis diagnosed 4 weeks before presentation and underwent right below-knee amputation. She was admitted to the hospital with abdominal pain and hematemesis.
On admission, the patient denied chest pain, shortness of breath, nausea, vomiting, diarrhea, or urinary symptoms. She also denied using over-the-counter medications. Home medications included aspirin, 81 mg, daily; furosemide, 20 mg, daily; insulin glargine, 10 units, daily; levofloxacin, 500 mg, daily; and linezolid, 600 mg, twice a day.
On physical examination, the patient’s body temperature was 36.5°C, heart rate was 86 beats/min, blood pressure was 105/53 mmHg, respiration rate was 14 breaths/min, and oxygen saturation was 98% while breathing room air. Cardiopulmonary examination findings were unremarkable. Her abdomen was distended with mild epigastric tenderness to palpation. There was no lower extremity edema. Neurologic examination was positive for asterixis.
Patient’s initial laboratory studies were hemoglobin 6.9 g/dL, white blood cell count 2500/μL, platelet count 24 × 10 3 /μL, sodium 131 mmol/L, potassium 5.5 mmol/L, chloride 94 mmol/L, bicarbonate 10 mmol/L, serum creatinine 0.7 mg/dL, BUN 29 mg/dL, calcium 7.9 mg/dL, albumin 2.3 g/dL, glucose 170 mg/dL, alanine aminotransferase 7 U/L (reference: 0 to 45), aspartase aminotransferase 16 U/L (reference: 7 to 40), alkaline phosphatase 256 U/L (reference: 40 to 150), bilirubin total 0.3 (reference: 0 to 1.5), partial thromboplastin time (PTT) 50 seconds, international normalized ratio (INR) 1.2, lactate dehydrogenase 125 U/L (reference: 0 to 249), lactate 11.5 mol/L, β-hydroxybutyrate 287 mOsm/L. β-Hydroxybutyrate 0.3 mg/dL (reference: 0 to 3), serum osmolality 287 mOsm/kg, serum acetaminophen less than 5.0 μg/mL serum salicylate level less than 0.3 mg/dL, serum ethanol level 2 mg/dL. Arterial blood gas revealed a pH 7.34, PCO 2 19 mmHg, PO 2 116 mmHg, bicarbonate 10 mmol/L. Urinalysis results were benign and blood and urine cultures were negative.
Computed tomography of the abdomen demonstrated moderate ascites and suggested liver cirrhosis. Chest x-ray revealed no acute cardiopulmonary process.
What is the acid-base disturbance in this case?
- A.
High anion-gap metabolic acidosis with respiratory alkalosis
- B.
High anion-gap metabolic acidosis
- C.
Respiratory alkalosis
- D.
Distal renal tubular acidosis
The correct answer is A
Comment: This patient has a high–anion gap (AG) metabolic acidosis; corrected for hypoalbuminemia, the AG equals 31. The increase in AG was essentially matched by a decrease in serum bicarbonate concentration [HCO 3 − ] according to the delta ratio, (AG − 12)/(24 − [HCO 3 − ]), which is 1.35 in this case. A delta ratio between 1 and 2 suggests that the patient may not have an additional acid-base disorder. However, this equation assumes that all buffering is extracellular and by bicarbonate, neither of which is correct. The calculated PaCO 2 by Winter’s formula, that is, PCO 2 = (1.5 × [HCO 3 − ] + 8) ± 2, is 23 mmHg, which is above the arterial blood gas–measured PCO 2 of 19 mmHg, indicating that the patient also has a respiratory alkalosis. In summary, our patient has a high-AG metabolic acidosis with mild respiratory alkalosis.
What is the most likely cause of this patient’s acid-base disturbance?
Comment: Patient’s acid-base disturbance can be further investigated through calculation of her osmolar gap (OG). The calculated osmolality, equivalent to {[2 × (sodium in mmol/L)] + [glucose in mg/dL]}/{18 + [(serum urea nitrogen in mg/dL)/2.8] + [ethanol/3.7]}, is 282 mOsm/kg in our patient. The OG is calculated by taking the difference between measured and calculated serum osmolality, and a normal OG is ≤ 10 mOsm/kg. Our patient had an OG of 5 mOsm/kg so it is unlikely that she had intoxication with glycols or methanol.
The elevated lactate level in this setting indicates the presence of lactic acidosis. The patient does not have signs of shock or hypovolemia. We need to account for our patient’s lactic acidosis in the setting of normotension. Salicylate toxicity can cause respiratory alkalosis with high-AG metabolic acidosis. However, it does not explain the patient’s lactic acidosis. Moreover, serum salicylate level was undetectable. Ethylene glycol can lead to a false elevation in L-lactate level due to cross-reactivity of its metabolites with the oxidase enzyme that is commonly used to measure ethylene glycol level. However, the normal OG in this patient makes ethylene glycol and methanol toxicity less likely. Also, the patient has no history of any other toxic alcohol exposure. Pyroglutamic acid can be associated with lactic acidosis; however, this patient did not have a history of acetaminophen use. The normal β-hydroxybutyrate level and lack of ketonuria exclude ketoacidosis.
Linezolid is an oxazolidinone antibiotic that inhibits bacterial protein synthesis. It can also impair mitochondrial ribosomal function, leading to severe lactic acidosis, liver toxicity, and myelosuppression.
Nucleoside analogue reverse transcriptase inhibitors, propofol, and metformin can also lead to mitochondrial dysfunction and metabolic acidosis. Major risk factors for linezolid toxicity include prolonged exposure, administration of relatively higher doses, and baseline chronic liver or kidney disease.
This patient’s high-AG lactic acidosis is most likely explained by linezolid toxicity. The patient’s respiratory alkalosis was thought to be due to her cirrhosis, which is associated with an increased level of progesterone, leading to hyperventilation.
What is the BEST treatment for this patient’s metabolic abnormalities?
Comment: Lactic acidosis often resolves rapidly following discontinuation of linezolid therapy. The medication was stopped and repeat laboratory measurements at 72 hours demonstrated significant improvement and normalization of the patient’s bicarbonate and lactate values.
Case Study 2
A 15-year-old male was admitted to the hospital with myalgias and generalized weakness. Two weeks before, he had abdominal pain, decreased oral intake, nausea, and vomiting. He denied diarrhea. One week before admission, he developed muscle weakness that worsened until he was unable to get out of bed without assistance. His medical history was unremarkable. His only reported medication was over the-counter ibuprofen for chronic back pain, 2400 to 3200 mg daily for several months. He denied alcohol or solvent abuse. On physical examination, he was alert and oriented. His vital signs were normal. He was unable to raise his upper or lower extremities against gravity and had decreased deep tendon reflexes with intact superficial sensations. The rest of his examination findings were unremarkable. Initial laboratory workup showed normal blood cell counts. Patient’s basic metabolic panel were: sodium 142 mmol/L, potassium 1.7 mmol/L, chloride 113 mmol/L, bicarbonate 20 mmol/L, anion gap 9 mmol/L, blood urea nitrogen (BUN) 18 mg/dL, creatinine 1.0 mg/dL, glucose 122 mg/dL, calcium 9.3 mg/dL, phosphorous 1.8 mg/dL, total protein 6.8 g/dL, albumin 4.3 g/dL, magnesium 2.7 mg/dL, creatine kinase 430 U/L (reference: 34 to 294). Venous blood gas revealed pH 7.32, PCO 2 31 mmHg, PO 2 158 mmHg, bicarbonate 15 mmol/L. Antinuclear antibody (ANA), anti-Sjögren syndrome antigen A and B antibodies were 5 U/mL (reference: 0 to 100). The 24-hour urine volume was 5750 mL containing sodium 466 mmol/L, potassium 112 mmol/L, chloride 552 mmol/L, and creatinine 2.25 g mg/dL with osmolality 281 mOsm/kg H 2 O. Urinalysis was normal with a pH of 7.0.
The electrocardiogram showed flattened T waves in lateral leads. He received 120 mmol of potassium overnight, and 12 hours after admission, his serum potassium level increased to 2.1 mmol/L.
What is the MOST likely diagnosis in this patient?
- A.
Hypokalemia secondary to reversible type 2 proximal renal tubular acidosis likely from ibuprofen
- B.
Hypokalemia secondary to reversible type 1 renal tubular acidosis, likely from ibuprofen
- C.
Sjögren syndrome
- D.
Pseudohyperaldosteronism
The correct answer is B
Comment: A systematic approach to hypokalemia can help narrow the differential diagnosis in this case. Hypokalemia can result from shifts of potassium from the extracellular to the intracellular space (internal balance) or from potassium depletion due to losses into the gastrointestinal tract or urine (external balance). Severe hypokalemia with paralysis due to an intracellular potassium shift can be due to hypokalemic periodic paralysis (hereditary or thyrotoxic) or exogenous insulin or catecholamines. In this patient, the relatively high urinary potassium excretion and need for large doses of potassium during replacement as well as the clinical course makes us conclude that he was potassium depleted. The causes of potassium depletion include vomiting, diarrhea, renal tubular acidosis (RTA), toluene toxicity, diuretic use, Bartter and Gitelman syndromes, and acquired or hereditary hypertensive renal potassium wasting disorders.
The expected renal response to hypokalemia would be to limit urinary potassium excretion. A patient has abnormal urinary losses during hypokalemia if potassium excretion surpasses 30 mEq in a 24-hour urine collection or a random urine potassium-creatinine ratio is greater than 13 mmol/g. This patient had 30 mmol of potassium per gram of creatinine in the urine on admission and 112 mmol of potassium in the 24-hour urine collection (while getting potassium repletion). The cause of renal potassium wasting can be determined from the associated acid-base disturbance and blood pressure. Patient had a normal anion gap metabolic acidosis. Therefore, there is no need to consider the various causes of hypertensive or normotensive renal potassium wasting with metabolic alkalosis. RTA, toluene toxicity, or diarrhea could cause normal anion gap metabolic acidosis and potassium wasting. RTA with hypokalemia can be seen in type 1 (distal) or type 2 (proximal) RTA. Proximal RTA typically has mild hypokalemia, an acid urine pH (unless treated), and other proximal tubular abnormalities (Fanconi syndrome) that were absent in this patient. Severe hypokalemia and alkaline urine pH are features of distal RTA. Inhaling solvent fumes, such as glue sniffing or paint huffing, results in systemic absorption of toluene, which is oxidized to benzoic acid and then conjugated with glycine to form hippuric acid. These hippurate anions are then secreted into the tubular lumen with sodium and potassium (along with ammonium, which raises the urine pH) causing a very similar picture to distal RTA. In addition to urine potassium and pH, other relevant urine parameters include the urinary osmoles (sodium, potassium, urea, and glucose), which enable us to calculate the urinary osmolar gap, the difference between measured urine osmolarity and calculated urine osmolarity (2 × [Na + + K + ]) + [urea ÷ 2.8] + [glucose ÷ 18]), which serves as a surrogate of ammonium excretion.
A normal anion gap metabolic acidosis caused by diarrhea or solvent abuse is associated with high rates of urine ammonium excretion; the rate of urine ammonium excretion is not high in RTA. Urine ammonium is not routinely measured by clinical laboratory tests, but it can be estimated from the gap between measured and calculated urine osmolality; urine ammonium excretion is approximately equal to half the urine osmolar gap. In this patient, the 24-hour urine osmolar gap was not high and hippuric acid was not found in urine. The elevated urine pH without an increase in urinary osmolar gap is consistent with a diagnosis of distal RTA.
The acute onset of distal RTA without nephrocalcinosis makes an acquired rather than a congenital form more likely. Autoimmune disease, most often Sjögren syndrome, is the most common cause of acquired distal RTA. Serologic testing was negative for autoimmune causes, so we should consider additional causes. Although ibuprofen has typically been associated with hyperkalemia, there have been several case reports of severe hypokalemia and transient distal RTA due to abuse of a combination drug containing ibuprofen and codeine. The pathogenesis is unclear but inhibition of carbonic anhydrase, which is present in the proximal and distal tubules, has been suggested. Consistent with the suspicion of ibuprofen being the etiologic agent, after the patient stopped treatment with the medication, his serum potassium level increased dramatically and renal losses decreased. He was discharged with a normal potassium level, normal bicarbonate level, and had no subsequent recurrences.
Case Study 3
A 13-year-old boy with a medical history of type 2 diabetes mellitus and hyperlipidemia was admitted to the hospital for elective surgery to resect an enlarging kidney cyst. His home medications included canagliflozin, 300 mg, daily; rosuvastatin, 20 mg, daily; glipizide, 5 mg, twice daily; and sitagliptin/metformin, 50/1000 mg, twice daily. The patient tolerated the procedure well and was transferred postoperatively to a surgical floor with unremarkable laboratory values. He did well in the immediate postoperative period and was tolerating clear liquids by postoperative day 3. His blood glucose level was regulated by short-acting insulin doses. His outpatient medications were not administered in the hospital. On postoperative day 4, the patient was noted to be tachypneic and tachycardic.
At that time, serum sodium was 136 mmol/L, potassium 5 mmol/L, chloride 108 mmol/L, and bicarbonate 9 mmol/L.
Serum urea nitrogen 22 mg/dL, creatinine 1.0 mg/dL, calcium 8.5 mg/dL, magnesium 2.2 mg/dL, phosphorous 3.8 mg/dL, albumin 3.8 g/dL, glucose 136 mg/dL, lactate 1.7 mmol/L, β-hydroxybutyrate 5.92 mmol/L. Urine glucose was 1000 mg/dL, ketones greater than 150 mg/dL. Arterial blood gas showed pH 7.13, PCO 2 13 mmHg, PO 2 103 mmHg, and bicarbonate 8.5 mmol/L.
Patient received multiple ampules of sodium bicarbonate and a continuous sodium bicarbonate infusion. Nephrology service was consulted for management of metabolic acidosis.
What is the cause of this patient’s metabolic acidosis and how should this condition managed?
- A.
Canagliflozin (SGLT2 inhibitor) associated euglycemic ketoacidosis
- B.
Lactic acidosis
- C.
Hypoglycemic ketoacidosis
- D.
Glycol intoxication
The correct answer is A
Comment: This patient has high anion gap metabolic acidosis due to ketoacidosis, despite the near-normal blood glucose concentration. He has normal plasma lactate levels but elevated urinary and blood ketone levels. Euglycemic ketoacidosis is an uncommon complication associated with sodium-glucose cotransporter 2 (SGLT2) inhibitor therapy. In SGLT2 inhibitor-treated patients with type 2 diabetes, a lower insulin to glucagon ratio leads to nearly 40% higher lipolysis after a meal, 20% higher fat oxidation, 60% lower carbohydrate oxidation, 15% lower glycogen synthesis, and 2-fold higher ketogenesis. The exact mechanism for the development of the more serious euglycemic ketoacidosis associated with SGLT2 inhibitor therapy is not clearly understood, though various theories have been postulated. These include a decrease in insulin levels as a result of insulin-independent hypoglycemia leading to increased lipolysis and enhanced ketogenesis, and a primary increase in glucagon secretion via direct effects on SGLT2 transporters expressed in pancreatic alpha cells leading to increased ketogenesis. Overall, it appears that similar to severe starvation ketoacidosis, SGLT2 inhibitors via various metabolic effects can lead to a low-insulin and high-glucagon state favoring ketogenesis, particularly in the presence of “stressors” such as surgery, excess alcohol consumption, pancreatitis, or severe injury.
SGLT2 inhibitors act by promoting glucose excretion in urine via inhibition of the SGLT2 cotransporter located in the proximal tubule. These drugs lower maximum renal glucose reabsorptive capacity and the renal threshold for glucosuria. There is also evidence that these agents may have beneficial effects by lowering intraglomerular pressure through increasing sodium chloride delivery to the juxtaglomerular apparatus and activating the tubulo-glomerular feedback pathway. These agents have relatively modest hypoglycemic efficacy, but are reported to have beneficial effects on blood pressure, weight, and insulin sensitivity, as well as improved cardiovascular and renal outcomes in patients with type 2 diabetes. Three SGLT2 inhibitors (dapagliflozin, canagliflozin, and empagliflozin) are currently FDA-approved for use in patients with type 2 diabetes. The pharmacokinetic profile of these agents is similar in patients with and without diabetes, and age, race, sex, body weight, food intake, or mildly decreased kidney function does not affect the pharmacokinetic characteristics of the drugs. However, dose reductions are required for moderately decreased kidney function.
Genital mycotic infections (more common in women), urinary tract infections, and orthostatic hypotension due to extracellular fluid volume depletion are the most commonly reported side effects. The true frequency of euglycemic ketoacidosis has not been established.
Early recognition of ketoacidosis is the key to successful therapy, but the relatively normal blood glucose levels and a predominance of β-hydroxybutyrate ketone bodies, which are not detected on urine dipsticks, can present a diagnostic challenge. Intravenous bicarbonate therapy alone does not address the underlying pathophysiology. Discontinuing the offending medication, early initiation of intravenous insulin therapy to suppress lipolysis and ketogenesis, and monitoring β-hydroxybutyrate level, as well as other metabolic and acid-base parameters, are all required. In addition, dextrose-containing fluids may be needed to avoid hypoglycemia. An interesting observation in this case is that although the pharmacokinetic half-life of SGLT2 inhibitors is approximately 12 hours, delayed reversibility of the drug’s action as manifested by ongoing glycosuria led to persisting metabolic acidosis for several days after cessation of the drug. Although the mechanism of a prolonged action is uncertain, it may reflect continued drug binding to renal transport proteins despite elimination from plasma. Intravenous insulin therapy in this patient was continued along with glucose infusions until postoperative day 11, at which point his serum electrolyte levels normalized and glycosuria was diminished to 300 mg/dL. On postoperative day 11, he was successfully converted to a subcutaneous insulin regimen and discharged on postoperative day 14 on insulin and metformin therapy. Thus, the persistent action of these drugs several days after discontinuation may require prolonged dextrose and insulin therapy to treat SGLT2 inhibitor therapy-associated ketoacidosis.
Case Study 4
A 19-year-old female with a history of depression presented to the emergency department after drinking three-fourth of a gallon of antifreeze over 2 days in a suicide attempt. She reported feeling as if she were “drunk.” She also noted nausea and vomiting. On physical examination, the patient appeared comfortable, alert, and oriented. Temperature was 36.5°C, heart rate was 110 beats/min, blood pressure was 100/70 mmHg, respiratory rate was 20 breaths/min, and pulse oximetry was 99% on room air. There was no papilledema. The remainder of the examination had normal findings. Laboratory analysis showed the following values: sodium, 136 mmol/L; potassium, 4.2 mmol/L; chloride, 104 mmol/L; bicarbonate, 17 mmol/L; blood urea nitrogen (BUN), 17 mg/dL; creatinine, 3.0 mg/dL; glucose, 147 mg/dL; corrected anion gap, 18.5; measured serum osmolality, 342 mOsm/kg; lactate, 9.9 mmol/L; pH 7.27; and PCO 2 , 36 mmHg. Urinalysis showed specific gravity of 1.010 and trace protein and was negative for blood and ketones. Sediment evaluation showed 0 to 2 red blood cells/high-power field, 0 to 2 white blood cells/high-power field, rare granular casts, and no crystals.
What is the MOST likely cause of metabolic acidosis in this patient?
- A.
Propylene glycol intoxication
- B.
Ethanol intoxication
- C.
Methanol intoxication
- D.
Salicylate overdose
The correct answer is A
Although the differential diagnosis of anion gap metabolic acidosis is broad, it is important to rapidly diagnose disorders that require prompt treatment to prevent morbidity and mortality. In individuals who present with altered sensorium or who report ingestions, the clinician must consider consumption of a toxic alcohol. These agents are rapidly absorbed from the gastrointestinal tract and initially will increase serum osmolality. Their presence can be ascertained quickly by measuring the osmolar gap: the difference between measured osmolality and calculated osmolality. Calculated osmolality is determined by multiplying the major extracellular cation, sodium (Na + ) ion, by 2 and converting the predominant extracellular solutes, glucose and BUN, from their traditionally reported units (mg/dL) to mOsm/kg by dividing by one-tenth of their molecular weight (2Na + + Glucose ÷ 18 + BUN ÷ 2.8). In this case, the osmolar gap is 56. A gap greater than 15 suggests the presence of a small-molecular-weight substance. Ethanol and isopropyl alcohol ingestions will present with an osmolar gap but do not cause metabolic acidosis. However, because isopropyl alcohol is metabolized to acetone, ketones may be noted if the nitroprusside reaction is used. Three alcohols—methanol, ethylene glycol, and propylene glycol—are associated with both an osmolar gap and an anion gap metabolic acidosis. Ethylene glycol and methanol are highly toxic. As these two alcohols are metabolized into glycolic/oxalic acid and formic acid, respectively, the osmolar gap will decrease while the anion gap increases. Propylene glycol, used to solubilize numerous medications, is metabolized to lactic acid and is an increasingly recognized cause of anion gap acidosis. Medications using this diluent, such as benzodiazepines, if infused rapidly enough can cause an osmolar gap and lactic acidosis.
There are several clues to the correct diagnosis in this case. Both ethylene glycol and methanol are extremely toxic, with 1 mL/kg usually resulting in death. Even if exaggerated, the quantity the patient claimed to have ingested should have been rapidly fatal. Methanol ingestions are associated with visual disturbances through inflammation of the optic nerve, which was not noted. The lack of oxalate crystals in the urine makes ethylene glycol toxicity less likely. Finally, although lactic acidosis can be seen in cases of ethylene glycol toxicity, the level present in this case would be very unusual. Although propylene glycol toxicity is most often noted in hospitalized patients receiving intravenous medications, there has been an increase in the use of propylene glycol as a substitute for ethylene glycol in commercial antifreeze because of its relative lack of toxicity. These agents are advertised as being pet friendly and less toxic. The US Food and Drug Administration considers pharmaceutical-grade propylene glycol as generally safe. However, prolonged high-dose infusions of medications containing propylene glycol can produce significant lactic acidosis through its metabolism. Although no lethal dose has been established in humans, in animals, the LD 50 is18 to 20 mL/kg.
Two days after admission, this patient’s initial ethylene glycol level returned as undetectable and family members brought in the ingested substance, Sierra antifreeze (Safe Brands Corp), containing 94% propylene glycol.
Toxic alcohol ingestions need to be treated rapidly to avoid toxicity. Often treatment must start prior to confirmation of the substance ingested. Because the toxicity of these alcohols is mediated by their metabolites, treatment is aimed at blocking their enzymatic conversion by alcohol dehydrogenase. This is accomplished by the administration of fomepizole, a potent inhibitor of alcohol dehydrogenase. Dialysis then can be considered to remove the alcohol and treat the acidosis. Propylene glycol is far less toxic than ethylene glycol. Toxicity usually manifests as confusion and kidney failure. Although deaths from propylene glycol toxicity are extremely rare, fomepizole has been used in treatment. Although in retrospect ethylene glycol toxicity was unlikely, in view of the morbidity associated with its ingestion, this patient was treated with fomepizole.
Case Study 5
An 18-year-old woman with a 4-year history of recurrent dyspnea was evaluated for 4 days of worsening shortness of breath and anorexia. One month before admission, fasting hyperglycemia was diagnosed and she was prescribed a low-carbohydrate and a high-protein diet. On examination, blood pressure was 112/71 mmHg and heart rate was 141 beats/min. She weighed 42 kg and was 162 cm tall; body mass index was 16 kg/m 2 . She was alert, but afebrile and emaciated and had dry skin. Initial laboratory findings showed metabolic acidosis and ketosis. Intravenous hydration with normal saline solution was started, and nutritional supplements with protein and amino acids were given. Despite these measures, the patient developed blurred vision and confusion on hospital day 4. Neurologic examination showed normal cranial nerves, including no ophthalmic paresis or facial diplegia, but decreased visual acuity. Computed tomography of the brain showed an area of decreased attenuation within the bilateral basal ganglia. Admission blood biochemistry and arterial blood gas results are as follows: serum sodium 138 mmol/L, potassium 3.1 mmol/L, chloride 116 mmol/L, bicarbonate 9.0 mmol/L, anion gap 15 mmol/L, BUN 15 mg/dL, creatinine 1.3 mg/dL, estimate glomerular filtration rate (eGFR) 48 mL/min/1.72 m 2 , albumin 3.2 g/dL, ketone 6.0 mmol/L, glucose 110 g/dL, osmolality 285 mOsm/kg, pH 7.36, PCO 2 16.2 mmHg. Of note is the development of profound hyperammonemia with an ammonia level of 327 g/dL (192 mmol/L).
What is the most likely cause of this patient’s metabolic acidosis and how might this patient be managed?
- A.
Starvation ketoacidosis
- B.
Lactic acidosis
- C.
Methylmalonic acidemia complicated with toxic amblyopia.
- D.
Methanol intoxication
The correct answer is C
Comment: In the setting of a low serum albumin level, the patient’s serum anion gap of 13 mEq/L (13 mmol/L) represents an expanded anion-gap metabolic acidosis. According to the clinical course and original laboratory data, a tentative diagnosis of starvation ketoacidosis was made; however, initial management with intravenous fluids failed to correct the metabolic acidosis. There was no clinical evidence of lactic acidosis, methanol intoxication (19 mg/L; toxic level, 200 mg/L), or acetaminophen overdose (0.12 g/mL; toxic level, 75 g/mL at 12 hours). Upon recognition of acute hyperammonemia, an occult metabolic disorder was suspected after acute liver failure, drug toxicity, and infectious complications were excluded. The constellation of metabolic acidosis, ketoacidosis, and hyperammonemia could be ascribed to an organic acidemia. These conditions are characterized by the excretion of non-amino organic acids in urine and disrupted serum amino acid metabolism, particularly of branched-chain amino acids. Methylmalonic and propionic acidemia are the most frequent forms of branched-chain organic acidemias. Affected patients typically present in the neonatal period or early infancy with signs of life-threatening metabolic acidosis and increased anion gap. A few are detected at an older age. Organic acidemias typically present with poor feeding, protein intolerance, vomiting, increasing lethargy that progresses to coma, and muscular hypotonia.
Serum amino acid analysis showed hyperglycinemia, and urine chromatography/mass spectrometry organic acid analysis indicated increased concentrations of methylmalonic, 2-oxooctanoic, and 1,2-benzenedicarboxylic acids. This picture is consistent with methylmalonic acidemia. MUT, MMAA , and MMAB are the 3 genes (encoding methylmalonyl coenzyme A mutase, methylmalonic aciduria type A protein, and methylmalonic aciduria type B protein, respectively) and there are at least eight different complementation groups associated with methylmalonic academia. Patients in whom residual enzymatic activity is present tend to have later and more variable presentations and a better prognosis. Affected individuals may appear well, but be prone to acute metabolic decompensation after excessive protein intake or metabolic stressors, as seen in our patient. Although genetic analysis was not performed in our patient, the constellation of urine and serum organic acid analysis, radiologic features, and the mentioned clinical findings should raise the suspicion of this under-recognized disease.
Because of the patient’s refractory hyperammonemia and worsening visual disturbance, continuous venovenous hemodialysis (CVVHD) therapy was initiated promptly before results of the urine organic-acid analysis were available. The patient’s consciousness cleared in the sixth hour of CVVHD, and she experienced gradual improvement in visual acuity in the following days. CVVHD therapy was halted after 60 hours because of resolution of hyperammonemia (ammonia, 58 μg/dL). Continuous renal replacement therapies are effective in the rapid clearance of these low-molecular-weight toxic metabolites, particularly in patients with inborn errors of metabolism, such as organic acidemia or urea cycle disorders. Prolonged latencies in the flashlight visual evoked potentials test were noticed initially, consistent with optic neuropathy (also termed toxic amblyopia). Methylmalonic acidemia complicated with optic neuropathy is rare and insidious and can progress to optic atrophy and irreversible visual deficits. After CVVHD therapy, visual disturbance and repeated visual evoked potentials testing showed a major recovery from the initial presentation.
In conclusion, this case highlights the use of dialysis for severe hyperammonemia in patients with underlying organic acidemia and acute neurologic manifestations. Prompt initiation is critical to avoid irreversible brain damage.
Case Study 6
A 14-year-old girl was seen for recurrent fractures. At the age of 2 weeks, she had been hospitalized because of failure to thrive and hyperchloremic metabolic acidosis. Laboratory findings at that time were as follows: pH, 7.15; PCO 2 , 48 mmHg; bicarbonate, 16.2 mmol/L; serum sodium, 139 mmol/L; potassium, 5.0 mmol/L; chloride, 114 mmol/L; and anion gap, 9 mmol/L. Her urinary pH was 7.8, with a urinary sodium level of 33 mmol/L, potassium level of 10 mmol/L, and chloride level of 17 mmol/L, giving a urinary anion gap of 26 mmol/L. Bicarbonate supplementation for renal tubular acidosis (RTA) was started, but over the years, it was decreased from 8 to 2 mEq/kg/day. The patient was of Mediterranean descent, and her parents were consanguineous in the second degree. Two brothers and four sisters were healthy. She had been evaluated for developmental delay and growth retardation, but no definitive diagnosis had been made. In addition, her dentition had required surgical intervention because of dental caries and misalignment. One and a half years before presentation, she fractured the first toe of her right foot after minor trauma. Two months before presentation, she fractured her first cervical vertebra from minor trauma. On physical examination, she was 1.46 m tall and 3 standard deviations (SDs) less than the mean for age, with a normal weight for height. Laboratory results were as follows: calcium, 9.0 mg/dL; phosphate, 4.83 mg/dL; alkaline phosphatase, 91 U/L; intact parathyroid hormone, 18.9 pg/mL (reference: 15 to 65 pg/mL); and 25-hydroxyvitamin D, 10 ng/mL (reference: 8 to 32 ng/mL). Capillary blood gas pH was 7.27, and bicarbonate level was 22.1 mmol/L. Urinary calcium excretion was 3.5 mg/kg/day (reference: 4 mg/kg/day). During bicarbonate loading, maximum increase in urinary PCO 2 was 77 mmHg (reference: 80 mmHg), with a urinary-blood PCO 2 difference of 32 mmHg (reference: 40 mmHg). Urinary pH increased from 5.96 to 8.3 during the test. Urinary citrate-creatinine ratio increased from 0.05 to 0.65 mmol/mmol. Ultrasound showed bilateral nephrocalcinosis.
Radiograph of the left hand showed increased cortical thickness and density of the bones of the hand and wrist, with bone-in-bone appearance in the metacarpal bones suggestive of osteopetrosis. In addition, there is an old avulsion fracture of the base of the proximal phalanx. The dual-energy x-ray absorptiometry scan of the lumbar spine revealed increased bone mineral density of the lumbar vertebrae.
What is the MOST likely diagnosis?
- A.
Renal tubular acidosis type 1 (RTA-1)
- B.
Renal tubular acidosis type 2 (RTA-2)
- C.
Carbonic anhydrase II deficiency
- D.
Renal tubular acidosis type 4
The correct answer is C
Comment: Based on hyperchloremic metabolic acidosis with a positive urinary anion gap, type 1 (distal) RTA was diagnosed in this patient. This was supported further by the bicarbonate-loading test, in which defective distal H + excretion was shown. Hypocitraturia, hypercalciuria (in our case, borderline), and nephrocalcinosis are also typical features of type 1 RTA. As expected, her bicarbonate needs decreased with time from 8 to 2 mmol/kg/day. Still, despite correction of acidosis, she showed poor height gain. In addition, she had developmental delay and dental decay, neither of which typically is encountered in patients with RTA. When she developed several fractures after minor trauma, osteopenia secondary to renal acidosis combined with hypercalciuria were first suspected. However, radiographs showed osteopetrosis, which was confirmed by means of dual-energy x-ray absorptiometry. The combination of RTA and osteopetrosis is suggestive of carbonic anhydrase II deficiency. This diagnosis was further supported by intracranial calcifications. Carbonic anhydrase II enzymatic activity in erythrocyte lysates was found to be deficient, and a novel homozygote c.2321GT mutation was identified in the patient. Her parents were heterozygous. This is the same nucleotide involved in the far more common “Arabic mutation,” but in the latter, the mutation is c.2321GA. Computed tomographic scan of the head revealed intracranial calcifications in the caudate nucleus typical for carbonic anhydrase type II deficiency.
Carbonic anhydrase II deficiency is inherited in an autosomal recessive mode. The underlying gene is mapped to chromosome 8q22, and several mutations have been described.
Carbonic anhydrase II is involved in proximal renal tubular bicarbonate reclamation and distal renal tubular acidification. Therefore, many patients with carbonic anhydrase II deficiency show characteristics of both proximal and distal RTA. Some patients develop nephrocalcinosis, as did our patient, in whose phenotype type 1 RTA predominated. Deficiency of carbonic anhydrase II disrupts the balance between osteoclast and osteoblast activity in favor of bone deposition. This causes excessive, but brittle, bone formation (osteopetrosis) that leads to an increased fracture risk. The function of carbonic anhydrase II in oligodendrocytes and astrocytes in the brain is unknown. However, most patients with carbonic anhydrase II deficiency develop intracranial calcifications involving the basal ganglia and periventricular and subcortical white matter. Calcification usually is not present at birth, but appears after the second year of life.
In contrast to other forms of osteopetrosis, cranial nerve entrapment and hematologic complications caused by bone marrow compression are relatively rare. There is no curative treatment for carbonic anhydrase II deficiency, and therapy is restricted to symptomatic correction of metabolic acidosis.
Case Study 7
A 17-year-old woman with a history of hypertension was admitted to the hospital for generalized weakness and muscle cramping with a greater than 2 packs per day smoking history for the past 2 years. She had been hospitalized 4 months prior with similar presenting symptoms, which were attributed to severe hypokalemia 2.0 mmol/L. She denied diarrhea, vomiting, laxative use, diuretic use, or alcohol abuse. Her home medications included potassium chloride, 40 mEq twice per day, and ibuprofen. Her vital signs at admission showed she was hypotensive (89/55 mmHg). The physical examination was significant for 4- or 5-muscle strength of upper and lower extremities. The admission laboratory findings were sodium 134 mmol/L, potassium 1.6 mmol/L, chloride 103 mmol/L, bicarbonate 20 mmol/L, calcium 10.0 mg/dL, magnesium 1.8 mg/dL, phosphorous 1.1 mg/dL, creatinine 1.0 mg/dL, glucose 132 mg/dL, albumin 3.9 mg/dL, and 25-hydroxyvitamin D 25 ng/mL.
The random urine electrolytes showed sodium 11 mmol/L, potassium 10 mmol/L, chloride 13 mmol/L, and anion gap 9 mmol/L. Urinary potassium-creatinine ratio (mmol/g) was 130 (> 13 suggestive of renal potassium wasting). 24-Hour urine potassium was 108 mmol (> 30 suggestive of renal potassium wasting), β 2 -microglobulin, greater than 20,000 μg/L (reference: < 300), retinol-binding protein-creatinine ratio 143,200 μg/g (reference: < 172), and glucose (dipstick) negative.
The electrocardiogram showed sinus arrhythmia, flattened T waves, and prolonged QT interval of 667 ms.
What is the Most Likely diagnosis and how should this patient be treated?
- A.
Lowe syndrome
- B.
Congenital Fanconi syndrome
- C.
Acquired Fanconi syndrome
- D.
Dent disease
The correct answer is C
Comment: The patient’s admission laboratory findings revealed a normal anion gap metabolic acidosis with positive urine anion gap-suggestive of renal tubular acidosis (RTA). The differential diagnosis includes proximal and distal RTA, given this patient’s hypokalemia with renal potassium wasting. Markedly elevated urinary excretions of β 2 -microglobulin and retinol-binding protein support a proximal tubulopathy.
Proximal RTA is caused by reduced reabsorption of filtered bicarbonate in the proximal convoluted tubule, which leads to renal bicarbonate wasting. In addition to decreased bicarbonate reabsorption, proximal RTA is commonly associated with other solute reabsorption impairments including phosphate, glucose, and amino acids. This generalized proximal tubulopathy is called Fanconi syndrome.
Most causes of proximal RTA are acquired, but inheritable causes of primary isolated proximal RTA can occur, as well as secondary proximal RTA with familial Fanconi syndrome in certain hereditary diseases (Wilson disease, cystinosis, Lowe syndrome, Fanconi-Bickel syndrome, Dent disease, etc.). Acquired proximal RTA is commonly caused by injury to the proximal tubule secondary to paraprotein disease, autoimmune disease (Sjögren syndrome), medication toxicity, or heavy metal toxicity. Severe vitamin D deficiency can also cause proximal RTA.
Given the patient’s proximal tubulopathy and absence of any known family history of potential hereditary causes, the patient was evaluated for acquired causes of proximal tubulopathy. Testing for paraprotein disease with serum protein electrophoresis with immunofixation, serum free light chains, and urine protein electrophoresis with immunofixation was unremarkable. Likewise, testing for autoimmune disease with antibodies against antinuclear and extractable nuclear antigens was unremarkable. Her 25-hydroxyvitamin D level was within normal limits. The review of this patient’s current and past prescriptions and over-the-counter medications did not identify any offending agent. A heavy metals screen was ordered to evaluate for potential heavy metal toxicity, including arsenic, cadmium, mercury, lead, copper, and zinc. The patient was found to have severe cadmium toxicity, with 4.4 μg in a 24-hour urine collection , corresponding to a cadmium-creatinine ratio of 6.24 μg/g.
Cadmium is a heavy metal that can cause both severe acute and chronic toxicity. The kidneys are the primary target organ for chronic cadmium toxicity. Sources of cadmium exposure include smoking cigarettes, eating contaminated foods (rice and grains farmed with cadmium-containing fertilizer), and occupational exposure.
The main source of cadmium exposure in smokers is inhalation of tobacco smoke, as opposed to ingestion of contaminated foods in never-smokers. Smoking doubles the lifetime body burden of cadmium.
Daily intake of cadmium through contaminated food is around 30 μg, of which only 1% to 10% is absorbed. A single cigarette has around 2 μg of cadmium, of which up to 10% is inhaled through smoking. Inhalation from smoking 1 pack (20 cigarettes) daily is between 1 and 3 μg.
The critical urinary cadmium-creatinine ratio associated with onset of renal tubular injury is 2 to 10 μg/g. This patient had a ratio of 6.2 μg/g.
After systemic absorption by inhalation or ingestion, cadmium is freely filtered across the glomerulus and reabsorbed by proximal tubular cells while bound to metallothionein. Metallothionein is degraded by lysosomes within tubular cells, releasing free cadmium that generates reactive oxygen species, which result in proximal tubulopathy and RTA.
Chronic cadmium toxicity can progress to Fanconi syndrome. Progressive loss of kidney function from cadmium toxicity does not commonly occur. Unfortunately, tubular injury from chronic cadmium toxicity is typically irreversible, even after cessation of exposure.
Management of this patient’s proximal tubulopathy and RTA starts with avoiding the offending agent: in this case, smoking cessation is required. This patient reported smoking 2 packs per day—exposure to approximately 2 to 6 μg of inhaled cadmium daily. The patient received education on the cause of her proximal tubulopathy and the link between cigarette smoking and cadmium toxicity.
After correction of this patient’s severe hypokalemia using intravenous potassium, she was prescribed potassium citrate with split dosing throughout the day for treatment of her proximal RTA and to maintain normokalemia. Bicarbonate supplementation in patients with proximal RTA can result in increased urinary bicarbonate excretion with resultant worsening of hypokalemia. Potassium levels must be monitored closely with alkali therapy; concurrent potassium supplementation is often required.
Case Study 8
A 13-year-old male with a history of epilepsy has a grand mal seizure. Laboratory tests taken immediately after the seizure has stopped reveal arterial pH 7.14, PCO 2 45 mmHg, serum sodium 140 mmol/L, K 5.5 mmol/L, chloride 98 mmol/L, and bicarbonate 17 mmol/L.
What is the acid-base disturbance and what will happen to his plasma potassium after correction of acidosis?
- A.
Mixed metabolic and respiratory acidosis
- B.
Simple metabolic acidosis
The correct answer is A
Comment: This patient has a combined respiratory and high anion gap metabolic acidosis, most likely due to seizure-induced lactic acidosis. There is likely to be no change, since neither lactic acidosis nor its correction seem to affect the internal distribution of potassium.
Case Study 9
An 18-year-old man (80 kg) with a history of chronic bronchitis develops severe diarrhea caused by pseudomembranous colitis. It is noted that the volume of diarrheal fluid is approximately 1 L/h. Results of the initial laboratory tests are: serum sodium 138 mmol/L, potassium 3.8 mmol/L, chloride 115 mmol/L, bicarbonate 9 mmol/L, arterial pH 6.07, and PCO 2 40 mmHg.
What is the most likely acid-base disorder and how much bicarbonate should be given to raise the plasma pH to 7.20?
- A.
Simple metabolic acidosis
- B.
Mixed metabolic acidosis and respiratory acidosis
- C.
Mixed metabolic acidosis and respiratory alkalosis
- D.
Mixed metabolic alkalosis and respiratory acidosis
The correct answer is B
Comment: This patient has a mixed metabolic and respiratory acidosis, since a PCO 2 of 40 mmHg is inappropriately high in a patient with a plasma bicarbonate concentration of 9 mmol/L. The expected value is about 22 mmHg, since the PCO 2 normally falls by about 1.2 mmHg for every 1 mmol/L reduction in the plasma bicarbonate concentration.
The H + concentration at a pH of 7.20 is 63 mmol/L. Thus,
[H + ] = 24 × [PCO 2 ] ÷ [HCO 3 − ] or 63 = 24 × 46/[HCO 3 − ] or [HCO 3 − ] = 15 mmol/L. At this degree of acidemia, the initial distribution of the excess acid is 50% to 70% of lean body weight. Thus,
HCO 3 − deficit = 0.6 × 80 × (15 − 9) or 288 mmol is needed to raise plasma pH to 7.20.
Case Study 10
A 15-year-old female has severe chronic renal failure. The following laboratory data are obtained: serum sodium 137 mmol/L, potassium 5.4 mmol/L, chloride 102 mmol/L, bicarbonate 10 mmol/L, arterial pH 7.22, PCO 2 25 mmHg.
Why does metabolic acidosis develop in renal failure?
- A.
Uremia
- B.
Reduced glomerular filtration rate
- C.
Decreased NH 4 + excretion
- D.
Reduced bicarbonate reabsorption
The correct answer is C
Comment: The metabolic acidosis in renal failure is primarily due to reduced NH 4 + excretion, which prevents the urinary excretion of all of dietary acid load.
Case Study 11
A 19-year-old woman with type 1, insulin-dependent diabetes is admitted to the hospital with a soft-tissue infection of the palate. The initial laboratory data include the following: serum sodium 140 mmol/L, potassium 3.8 mmol/L, chloride 110 mmol/L, bicarbonate 23 mmol/L, and glucose 147 mg/dL.
The patient eats sparingly because of pain on swallowing. To minimize the risk of hypoglycemia, her insulin is withheld. Repeat blood tests are obtained 36 hours later: serum sodium 135 mmol/L, potassium 5.0 mmol/L, chloride 105 mmol/L, bicarbonate 15 mmol/L, glucose 270 mg/dL, anion gap 15 mmol/L, ketone 4 + arterial pH 7.32, and PCO 2 30 mmHg.
Why is the anion gap only slightly elevated despite the presence of ketoacidosis?
- A.
β-Hydroxybutyrate and acetoacetate excretion in urine
- B.
Laboratory error
- C.
Increased serum sulfates and phosphates concentration
- D.
Ethylene glycol ingestion
The correct answer is A
Comment: The acidemia is due to retention of H + ions from the ketoacids; the associated anions (β-hydroxybutyrate and acetoacetate) were presumably excreted in the urine, resulting in only a minor elevation in the anion gap. The patient should be given insulin with glucose. This will correct the ketoacidosis without the risk of hypoglycemia.
Case Study 12
A 12-year-old girl was rushed into the Emergency Department following a motor vehicle accident and was found to have stroke as a result of left parietal hemorrhage. Her medical history was uneventful except for a history of chronic asthma diagnosed 2 years earlier. Physical examination revealed a well-nourished and developed child for her age. She was stuporous, disoriented, and did not follow commands. She was breathing comfortably in room air with deep respirations. The blood pressure was 120/78 mmHg, pulse 71 beats/min, and respiratory rate 29 breaths/min. Her chest was clear to auscultation and percussion. Examination of the eyes, ears, nose, and throat was unremarkable. The remaining physical examination was unremarkable. She was transferred to the neurosurgery intensive care unit for monitoring. She remained stable and repeated imaging demonstrated a resolving brain hemorrhage. Arterial blood gas (ABG) showed a blood pH of 7.44, PCO 2 24 mmHg, PO 2 90 mmHg, and bicarbonate 15 mmol/L.
What is the MOST likely acid-base disturbance in this patient?
- A.
Proximal renal tubular acidosis (RTA-2)
- B.
Distal renal tubular acidosis (RTA-1)
- C.
Chronic respiratory acidosis
- D.
Mixed metabolic acidosis and respiratory acidosis
The correct answer is C
Comment: A low plasma bicarbonate concentration due to CRA is often misdiagnosed as a metabolic acidosis and mistreated with the administration of alkali therapy especially when blood pH and PCO 2 are unavailable.
Our patient presented with a low serum bicarbonate concentration, hypocapnia, and positive UAG, which suggest the diagnosis of chronic respiratory alkalosis (CRA), distal renal tubular acidosis (dRTA), or mixed CRA and dRTA. The constellation of hyperventilation as a result of stroke and history of chronic asthma, blood pH in the upper range of normal, and positive urine anion gap (UAG) in the absence of chronic renal disease in our patient are consistent with the diagnosis of primary CRA rather than the presumed dRTA. The positive UAG is the result of suppressed ammonium (NH 4 ) excretion, which is a compensatory response to chronic alkalemia. The urine pH of 6.1 in our patient does not necessarily suggest a defect in hydrogen ion (H + ) secretion, as in the setting of chronic alkalemia; distal ammonia (NH 3 ) excretion increases to the extent that the secreted H + is almost completely buffered as ammonium (NH 4 ). This may result in a very low free H + in the lumen to lower the urine pH below 5.5, as was the case in our patient. However, the expected serum bicarbonate is 15 mEq/L for a PCO 2 of 24 mmHg in our patient, which closely approximates the expected renal compensation, satisfying the diagnosis of CRA and distinguishing CRA from mixed metabolic acidosis and CRA.
In CRA, UAG is increased (positive) on account of suppressed ammonium (NH 4 ) excretion, which is a compensatory response to chronic alkalemia. Because dRTA is relatively rare and CRA is frequently observed in hospitalized patients, UAG helps distinguish metabolic acidosis from CRA in the initial evaluation of hypocarbonatemia.
UAG can help in evaluating the cause of a low plasma bicarbonate concentration, as our teaching case illustrates. The UAG, calculated as urine (Na + + K + ) − (Cl − ) is a useful diagnostic index to estimate kidney ability to excrete ammonium in patients with metabolic acidosis, when urine ammonium is not measured.
There is a strong relationship between UAG and urine ammonium excretion; that is to say, the more ammonium in the urine, the lower the UAG is with a value ranging from 0 to 200 mEq/L during metabolic acidosis. In patients with dRTA, the UAG is positive, because of the impaired H + secretion in the distal nephron leading to reduced ammonium excretion.
In CRA, the UAG is also positive secondary to low ammonium excretion, which is a compensatory response to chronic alkalemia. In the present case, the diagnosis of CRA as the cause of the low plasma bicarbonate concentration was suspected before ABG evaluation because the patient had hyperventilation on physical examination and the UAG was positive. Moreover, there were no clinical features to suspect metabolic acidosis caused by dRTA and the patient did not have CKD to explain the low bicarbonate concentration and positive UAG.
In CRA, the renal adaptation involves suppression of ammonium excretion and decreasing bicarbonate reabsorption. This results in a net reduction in acid excretion that helps attenuate the increase in blood pH. The compensatory renal response to CRA is so efficient in lowering plasma bicarbonate concentrations that blood pH is close to normal.
It is worth noting that the ability of the UAG as a surrogate for ammonium excretion in patients with chronic kidney disease (CKD) is limited because urine ammonium excretion falls as glomerular filtration rate (GFR) declines. In addition, the quantity of unmeasured anions in the urine such as sulfate and phosphate, which are not included in the typical UAG calculation, critically influences the ability of UAG to estimate NH4 concentration in CKD patients.
Case Study 13
A 5-year-old white male with a history of bronchospasm was admitted to the hospital for treatment of status asthmaticus. Physical examination revealed a well-developed male in respiratory distress. Blood pressure was 115/59 mmHg, heart rate was 92 beats/min, respiratory rate was 18 breaths/min, and temperature was 38°C. Expiratory wheezing was heard bilaterally. Laboratory data on admission were blood pH 7.41, PCO 2 58 mmHg, sodium 141 mmol/L, potassium 4.6 mmol/L, chloride 100 mmol/L, and bicarbonate 29 mmol/L. The patient was treated with repeated doses of albuterol sulfate and intravenous infusion of theophylline and methylprednisolone. Oxygen was administered by nasal prongs at a flow rate of 4 L/min. After 36 hours, repeated laboratory studies revealed: serum sodium 142 mmol/L, potassium 3.1 mmol/L, chloride 93 mmol/L, bicarbonate 29 mmol/L, creatinine 0.5 mg/dL, and urea nitrogen 15 mg/dL. The arterial blood pH was 7.37, PCO 2 36 mmHg, and PO 2 88 mmHg.
What acid-base disturbance is present at this time?
- A.
Respiratory acidosis
- B.
Mixed metabolic alkalosis and metabolic acidosis
- C.
Mixed reparatory acidosis and metabolic acidosis
- D.
Mixed respiratory acidosis and metabolic alkalosis
The correct diagnosis is B
Comment: The initial blood gas values, with the pH of 7.41 and a PCO 2 of 58 mmHg, are consistent with the pattern of respiratory failure. The elevated serum bicarbonate (29 mmol/L) suggests that the respiratory acidosis had been proceeding for a sufficient time to permit some degree of renal compensation. As a rule, serum bicarbonate will increase by 3.5 mmol/L for each 10 mmHg rise in PCO 2 in chronic respiratory acidosis (Δ bicarbonate = 0.35 Δ PCO 2 ).
Therapy with bronchodilators normalized the blood pH but reduced blood PCO 2 . Moreover, the anion increased by 14 mmol/L, while blood bicarbonate concentration reduced by no more than 6 mmol/L. The increased anion gap and the low blood bicarbonate concentration signify the presence of metabolic acidosis. A diagnosis of pure metabolic acidosis could not be made in this patient since the fall in blood bicarbonate concentration is not matched by a commensurate rise in the unmeasured anion concentration. As a rule, with metabolic acidosis of the high anion gap variety, there should be a 1:1 correspondence between decreasing serum bicarbonate and increasing AG (Δ bicarbonate/ AG = 1). Whenever the anion gap is elevated but serum bicarbonate is not reduced reciprocally, a mixed metabolic acidosis and alkalosis must be strongly considered. In this patient, the reciprocity between the decrement in blood bicarbonate and increment in anion gap is lost by 8 mEq/L, providing evidence for a coexisting metabolic alkalosis. The presence of hypochloremia favors this interpretation.
The increased anion gap metabolic acidosis in this patient is the result of lactic acidosis owing to the therapy of bronchospasm. The finding of a blood lactate level of 6.1 mmol/L supports this diagnosis during albuterol therapy. With cessation of albuterol, the patient’s blood lactate fell within 24 hours to 0.7 mmol/L. During this period, his blood bicarbonate concentration increased, and his anion gap fell to 13 mmol/L. Increased work of breathing could not account for the rise in the blood lactate level in this patient, since the initial respiratory rate of 18 breaths/min argues against such a possibility. Furthermore, the patient never had hypotension, sepsis, signs of tissue hypoxia, or any of the other known causes of lactic acidosis. The cellular mechanism by which β2-adrenergic stimulation causes lactic acidosis is related to 13 z-receptor activation and subsequent stimulation of adenylate cyclase. Adenylate cyclase, in turn, enhances cyclic AMP-mediated activation of glycogenolysis, lipolysis, and Na + -K + -ATPase. As muscle lacks glucose-6-phosphatase, lactate is the end product of muscle glycogenolysis. Increased free fatty acids from lipolysis inhibit pyruvate oxidation, which also results in lactic production. Activation of Na + -K + in the cell membrane results in sodium efflux and potassium influx.
Theophylline is known to potentiate the β2-adrenergic effects by increasing the cellular cyclic AMP levels through inhibition of 5′-phosphodiesterase. Glucocorticoids also might enhance the sensitivity of β-receptors to β-adrenergic agents. The metabolic alkalosis in the patient is most likely the result of both hypokalemia and the state of posthypercapnia.
Case Study 14
A 16-year-old white male was found unconscious in the street and was brought into the emergency room. The patient was stuporous, without focal neurological signs. Blood pressure was 120/80 mmHg and his pulse 80 beats/min. Examination of the head, eyes, ears, nose, and throat was unremarkable. His chest was clear to auscultation and percussion. The remainder of the physical examination was unremarkable. Laboratory investigations showed: serum sodium 137 mmol/L, potassium 4.6 mmol/L, chloride 102 mmol/L, bicarbonate 15 mmol/L, urea nitrogen 43 mg/dL, creatinine 1.0 mg/dL, and glucose 200 mg/dL. Serum ketones were trace positive and serum osmolality was 330 mOsm/kg. Blood pH was 7.32 and PCO 2 was 28 mmHg. Urinalysis was negative with a pH of 5.1.
What acid-base disorder is present?
- A.
Simple anion gap metabolic acidosis
- B.
Mixed anion gap metabolic acidosis and respiratory acidosis
- C.
Mixed anion gap metabolic acidosis and respiratory alkalosis
- D.
Lactic acidosis
The correct answer is A
Comment: A low plasma bicarbonate concentration and a low pH signify the presence of metabolic acidosis. The PCO 2 is depressed by 10 mmHg and the plasma bicarbonate concentration by 9 mmol/L. This level of respiratory compensation is appropriate for the steady-state level of bicarbonate. The fall in plasma bicarbonate concentration is matched by a commensurate rise in the unmeasured anion concentration; thus, this is a pure high anion gap type of metabolic acidosis.
Under normal conditions, the osmolar gap, difference between the measured and the estimated serum osmolality [2 (sodium concentration (mEq/L)] + [BUN (mg/dL) + 2.8] + [glucose concentration (mg/dL)+18], is less than 10 mOsm/kg H 2 O. As a general rule, a value greater than this indicates the presence of an additional osmotically active substance in the circulation. Methanol intoxication, ethylene glycol intoxication, and alcohol ketoacidosis are disorders associated with high anion gap metabolic acidosis and increased serum osmolality. The measured serum osmolality in this patient was 330 mOsm/kg H 2 O, giving an osmolar gap of 30 mOsm/kg H 2 O. The patient had no oxalate crystals in the urine, as found in ethylene glycol intoxication, and there was no evidence of optic papillitis on funduscopic examination, as might be anticipated in a patient with methanol intoxication. Blood alcohol levels were very high, indicating the diagnosis of alcoholic ketoacidosis.
Case Study 15
A 2-year-old male child was admitted with shortness of breath and drowsiness for 1 day. He had suffered from acute intermittent diarrhea treated with loperamide and dioctahedral smectite and was fed with glucose water recently for 1 week. He had a pertinent history of midgut volvulus status post bowel resection at the age of 1 month. His pulse rate was 140 beats/min, blood pressure 70/46 mmHg, respiratory rate 40 breaths/min, and body temperature 37.0°C. Physical examination showed drowsy consciousness, suprasternal and intercostal retraction, and delayed capillary refill time. Laboratory studies were notable for profound, high anion gap (AG) metabolic acidosis (HCO 3 − 7.0 mmol/L, AG 25 mmol/L), hyperammonemia (ammonia 220 μg/dL), and hypokalemia (3.0 mmol/L). Urine analysis revealed Na + 69 mmol/L, K + 15 mmol/L, Cl − 58 mmol/L, and osmolality 420 mOsm/kg. Routine stool analysis did not show pus cells. His severe metabolic acidosis and hyperammonemia were unresponsive to intravenous high-dose sodium bicarbonate (NaHCO 3 20 mmol/kg/day) administration for 2 days.
What is the MOST likely cause of this patient’s metabolic acidosis and hyperammonemia?
- A.
D-lactic acidosis with hyperammonemia
- B.
Ketoacidosis
- C.
Uremic acidosis
- D.
Lactic acidosis
The correct answer is A
Comment: Patient presented with high AG metabolic acidosis and normal serum osmolal gap, kidney function, glucose, ketone bodies, and L-lactate, thus eliminating ethanol intoxication, uremic acidosis, ketoacidosis, and lactic acidosis as possible causes. In addition, he had a triad of the history of short bowel disease, diarrhea, and neurological features (altered mentality) suggesting D-lactic acidosis generated from gastrointestinal bacterial overgrowth in the colon. Higher serum D-lactate level (9.6 mmol/L) measured by spectrophotometric assay confirmed this diagnosis. Oral neomycin to eradicate the bacterial overgrowth led to normalization of serum D-lactate with correction of metabolic acidosis and rapid recovery of neurological features. Of note, hyperammonemia was also evident and could result from the failure of ammonia disposal in the liver and overproduction of ammonia. His liver function was normal. Defects in the urea cycle usually have normal acid-base state or an accompanied ketoacidosis (organic acidemia).
The overproduction of ammonia was highly responsible for his hyperammonemia and could originate from systemic ammonia generated by kidneys and skeletal muscle, and portal ammonia produced by gut microflora. Hypokalemia and metabolic acidosis as observed in our patient are two well-known factors to stimulate the systemic ammoniagenesis of kidneys and muscle. Ammoniagenesis by overgrown bacteria might also have been contributory. Gut ammonia production was completely resolved with the correction of D-lactic acidosis and hypokalemia.
Removal of the offending agents and elimination of the bacterial overgrowth in colon and repletion of volume with alkalization by sodium bicarbonate are the mainstay treatment of D-lactic acidosis. The offending agents, including carbohydrates and D-lactate-containing crystalloids, should be withdrawn. Probiotics containing D-lactate-producing Lactobacillus spp. commonly prescribed for gastroenteritis should be avoided. Oral non-absorbable antibiotics such as neomycin are absolutely “Sine Qua Non” to completely eradicate the overgrown bacterial flora in the colon, as shown in this case. Rarely, hemodialysis is indicated for refractory severe acidosis with compromised hemodynamics. For recurrent and severe D-lactic acidosis, cyclic antibiotic treatment and/or non-D-lactate-producing probiotics for alteration of intestinal microbiota could be considered.
Case Study 16
A 15-year-old Chinese girl presented to the emergency department with muscle paralysis of bilateral lower extremities over the course of 1 day. She had a 2-year history of polyuria, nocturia, and rampant dental caries and calculi. She denied vomiting, diarrhea, or use of alcohol, laxatives, or diuretics, and her family history was unremarkable.
Her pulse rate was 90 breaths/min, blood pressure 112/72 mmHg, and body temperature 36.4°C. Physical examination revealed severe dental caries and calculi with dry oral mucosa. Her thyroid gland was not enlarged. Neurologic examination disclosed symmetric flaccid paralysis with areflexia of both lower extremities. The remainder of the physical examination was unremarkable. The most striking biochemical abnormalities were profound hypokalemia (1.8 mmol/L) and hyperchloremic metabolic acidosis (pH 7.28, bicarbonate 16.6 mmol/L, Na + 141 mmol/L, and Cl − 114 mmol/L). Her renal, liver, and thyroid function were all normal (creatinine 0.9 mg/dL). Urinalysis revealed proteinuria (1 +), low urine specific gravity (1.010), high K + excretion (transtubular K + gradient 5, 24-hour urine K + 38 mmol/day), positive urine anion gap (Na + 43 mmol/L, K + 16 mmol/L and Cl − 39 mEq/L), and persistent alkaline urine (pH 7 to 7.5). Electrocardiogram revealed prolonged PR interval with flattened T wave. Abdominal ultrasonography showed bilaterally medullary nephrocalcinosis.
What is the MOST likely diagnosis in this patient?
- A.
Hypokalemic familial periodic paralysis
- B.
Systemic lupus erythematous
- C.
Sjögren syndrome
- D.
Fanconi syndrome
The correct answer is C
Comment: This 15-year-old girl presented with hypokalemic paralysis, which can result from hypokalemic periodic paralysis (HPP) due to acute K + shift into cells or non-HypoPP due to a large total body K + deficit. Measurements of urinary K + excretion and blood acid-base status can help in the differential diagnosis. Her high urinary K + excretion, reflected by an elevated trans-tubular potassium gradient (TTKG), suggested renal K + wasting and non-HypoPP. Her concurrent hyperchloremic metabolic acidosis suggested a condition with both K + depletion and direct or indirect bicarbonate loss (renal tubular acidosis). The assessment of urine NH4 + excretion by urine anion gap and/or urine osmolal gap separates renal tubular acidosis (RTA) from non-RTA. A positive urine anion gap indicated defective NH4 + excretion associated with RTA. Her persistently alkaline urine (pH > 7.0) pointed to a diagnosis of distal RTA. In fact, hypokalemia is a common finding in distal RTA and results from the combination of renal Na + wasting, secondary hyperaldosteronism, and bicarbonaturia.
Nevertheless, the underlying cause of distal RTA must be identified. Upon review of her history, she had been experiencing dry mouth for the past 3 months despite normal Schirmer’s test for dry eye. An exhaustive work-up demonstrated elevated anti-Ro antibody, rheumatoid factor, antinuclear antibody (> 1:1280), polyclonal IgG, typical delayed salivary secretion on salivary scintigraphy and sialo duct ectasia and typical periductal lymphocytic infiltration (focus score 3) in the salivary gland biopsy. Renal histology showed chronic tubulointerstitial nephritis with predominant lymphocytic infiltration. After ruling out other autoimmune diseases, like systemic lupus erythematous or juvenile arthritis, primary SS was diagnosed.
Sjögren syndrome is a chronic autoimmune disease characterized by progressive lymphocytic infiltration of exocrine glands with typical features of keratoconjunctivitis, sicca, and xerostomia. Various degrees of extraglandular involvement, like arthritis, RTA, and lymphoma may develop before or after glandular damage. SS is most prevalent in women in their fourth and fifth decades and uncommon in children or adolescents. The clinical presentations of juvenile Sjögren syndrome are diverse. Dry eye and dry mouth sensation are the most common presenting complaints in adults, but usually develop later in juveniles, reflecting the atypical presentations of juvenile Sjögren syndrome at the outset. The nonspecific clinical picture and lack of universal diagnostic criteria mean that most patients are under-diagnosed until they experience complications. Profound hypokalemia with paralysis is a rare primary manifestation of SS in children.
Impaired distal tubular H + secretion is by far the most common renal manifestation of Sjögren syndrome. It takes time to progress from impaired distal tubular H + secretion to full-blown RTA. Therefore, defective renal acidification (pH > 5.5) in response to the acid-load test and low urine citrate excretion are the earliest indices to suggest renal involvement in Sjögren syndrome. Impaired ability to concentrate (hyposthenuria) is also another marker of early primary Sjögren syndrome.
Hypokalemia and nephrocalcinosis (or nephrolithiasis) are common but late manifestations in distal RTA, because they are nearly asymptomatic (> 90%) in the early stages. The combination of renal Na + wasting, secondary hyperaldosteronism, and bicarbonaturia in distal RTA contribute to hypokalemia. Metabolic acidosis, per se, can induce bone resorption and reduce renal tubular calcium and phosphate reabsorption, leading to increased urine calcium and phosphate excretion. This, in combination with hypocitraturia, as a result of metabolic acidosis and hypokalemia, precipitates the formation of nephrocalcinosis or nephrolithiasis.
With respect to therapy, K + citrate must be used to correct hypokalemia and metabolic acidosis and prevent further nephrocalcinosis. Care for oral involvement includes mechanical stimulation of the salivary glands, diet modification, regular oral hygiene, and topical fluoride. Prompt recognition and management of Sjögren syndrome achieves a good prognosis by preventing glandular and extraglandular complications. Corticosteroids are the drugs of choice for Sjögren syndrome with visceral involvement. In life-threatening cases, mycophenolate mofetil and novel biological therapies like rituximab and infliximab can be attempted.
Case Study 17
A 10-week-old female infant developed hypertension. The elevated blood pressure was associated with metabolic alkalosis and urinary chloride wastage. The family history was unremarkable. Her urinalysis, blood urea nitrogen (BUN), and serum creatinine concentrations were all normal. A renal ultrasound was normal. A technetium-99m diethylenetriaminepentaacetic acid (DTPA) renal scans with captopril showed normal blood flow bilaterally. The head ultrasound and echocardiogram were normal. Blood epinephrine, norepinephrine, catecholamines, thyroxin, and steroid levels were also normal. Treatment with various combinations of labetalol, hydralazine, captopril, methyldopa, nifedipine, and spironolactone, all at high doses, failed to control the elevated blood pressure. Serum aldosterone level and peripheral plasma renin activity were low.
What is the MOST likely diagnosis?
- A.
Apparent mineralocorticoid excess
- B.
Congenital adrenal hyperplasia
- C.
Primary hyperaldosteronism
- D.
Liddle syndrome
The correct answer is D
Comment: Our patient had normal renal function and structure. In addition, her normal physical examination, baseline laboratory data, including the low plasma renin activity and aldosterone secretion, and the findings on echocardiogram, renal ultrasound, and renal scan excluded the possibility of coarctation of aorta, congenital heart disease, adrenogenital syndrome, and infectious, neurologic, endocrine, and renovascular causes of hypertension. The constellation of hypertension, metabolic alkalosis, and low plasma renin activity and aldosterone level in our patient prompted us to consider other diagnostic possibilities. The lack of therapeutic response to spironolactone, with good response to amiloride, strongly suggested the diagnosis of Liddle syndrome. The recurrence of hypertension and metabolic alkalosis after cessation of amiloride that was subsequently treated with amiloride confirmed the diagnosis of Liddle syndrome.
Liddle syndrome classically presents with the concurrent triad of hypertension, hypokalemia, and metabolic alkalosis similar to disorders caused by mineralocorticoid excess. The consistent finding among patients with Liddle syndrome is decreased aldosterone and renin secretion. However, not all patients with Liddle syndrome are hypokalemic at presentation, similar to our case.
The differential diagnosis of Liddle syndrome includes some forms of congenital adrenal hyperplasia including 11β-HSD, the syndrome of apparent mineralocorticoid excess, familial cortisol resistance, and a deoxycorticosterone-producing tumor. If a diagnosis is not firmly established, a therapeutic response to dexamethasone should lead to the suspicion of possible 11β-HSD. CT or magnetic resonance imaging can usually detect deoxycorticosterone-producing tumor.
The primary abnormality in Liddle syndrome is a distal renal tubular transport defect characterized by increased rate of sodium reabsorption and, in most cases, increased rate of potassium and hydrogen ion secretion resulting in fluid retention, hypertension, suppression of renin and aldosterone secretion and hypokalemic metabolic alkalosis. Data derived from cloned sodium channel genes indicate that the site of the enhanced activity of the sodium channel, in Liddle syndrome, is the late distal tubule and cortical outer medullary portions of the collecting tubule. The genetic abnormality involves the beta or gamma subunit of the collecting tubule sodium channel.
Therapy in Liddle syndrome consists of prescribing amiloride or triamterene, which directly close the sodium channels. The mineralocorticoid antagonist spironolactone activity is not mediated in this disorder.
Case Study 18
A 25-day-old male infant was admitted to the hospital because of diarrhea and dehydration. His mother was a 22-year-old black female, who had an uncomplicated pregnancy and delivery. His birth weight was 2.4 kg. He was discharged from the premature nursery at 2 weeks of age, weighing 2.32 kg. A routine urinalysis at 1 week of age was normal. At home he did well initially but then began to feed poorly. When seen in the emergency room he was severely dehydrated, cachectic, and tremulous. His weight was 2.20 kg, temperature 38.7°C, pulse 140 beats/min, and respiratory rate 30 breaths/min. Physical examination was otherwise unremarkable. Laboratory data on admission included the following: hematocrit 50%, blood urea 19 mg/dL, Na + 159 mmol/L, Cl − 138 mmol/L, and bicarbonate 14.8 mmol/L. Urinalysis revealed the following: pH 5, trace of protein, and no cells. Blood, stool, and urine cultures were negative. On admission the diagnosis of sepsis was suspected; antibiotics were given for 10 days in addition to conventional intravenous fluids. After 3 days of therapy the infant was markedly improved, having gained 320 g in weight. On the 6th hospital day, hyperchloremic acidosis was still present despite complete correction of the dehydration. The laboratory findings were blood pH 7.17, Na + 132 mmol/L, C1 − 120 mmol/L, K + 5.2 mmol/L, bicarbonate 10.0 mmol/L, urea 21 mg/dL, calcium 19.1 mg/dL. The urine pH was 5.5 and the urinary molar ratio of calcium to creatinine was 0.96. The acidosis persisted and he was started on an oral citrate mixture (10 mEq/day) and calcium gluconate. On the 10th hospital day, the blood pH was 7.18, bicarbonate 12.5 mmol/L, and Cl − 115 mmol/L. The dose of citrate was increased to 40 mEq/day (16 mEq/kg) with gradual and complete correction of the acidemia. The effect of this therapy was remarkable in that the baby gained 2 kg in the following 2 months. While receiving citrate, the urine pH was consistently above 7, and the Ca/Cr ratio decreased to 0.45. At 3 months of age inulin clearance was 86 mL/min/1.73 m 2 . Citrate therapy was stopped, with immediate reappearance of the hyperchloremic acidosis, which, however, disappeared promptly with reinstitution of therapy. The infant was discharged at 3.5 months of age, in good health, weighing 4.96 kg. He received a regular diet plus 40 mEq/day sodium citrate.
What is the MOST likely diagnosis?
- A.
Proximal renal tubular acidosis
- B.
Distal renal tubular acidosis
- C.
Tubulointerstitial disease
- D.
Fanconi syndrome
The correct answer is D
Comment: The persistence of hyperchloremic acidosis, with no apparent cause, combined with normal renal function, suggests the diagnosis of renal tubular acidosis (RTA). The ability to acidify the urine maximally and, during acidosis, to excrete titratable acid and ammonium at normal rates excludes the diagnosis of distal RTA.
Most commonly proximal RTA is one abnormality in a complex of renal tubular dysfunction, as encountered in the Fanconi syndrome. These disorders are excluded by examinations for abnormalities other than RTA, including polyuria, aminoaciduria, glucosuria, phosphaturia, nephrocalcinosis, bone disease, and serum abnormalities other than hyperchloremic acidosis.
The diagnosis of proximal RTA, in which there is a large ongoing loss of bicarbonate in the urine, often exceeding 10% to 15% of the filtered load, is supported by the need to provide citrate at a rate of 10 or more mEq/kg/24 h in order to maintain the serum bicarbonate within the normal range.
The diagnosis of proximal RTA is confirmed by the demonstration of a renal bicarbonate threshold below normal for age. Some infants with distal RTA are “bicarbonate wasters,” but they usually do not excrete more than 5% of their filtered bicarbonate.
Patients with isolated proximal RTA do not waste potassium and, therefore, can be treated exclusively with sodium salts (usually sodium bicarbonate or sodium citrate). Their dosage requirement, however, must be adjusted to maintain the blood bicarbonate within normal limits. The most important evidence of adequacy of treatment is acceleration in the rate of growth to achieve catch-up and then maintenance of a normal growth velocity.
Case Study 19
A 16-year-old white male was found unconscious in the street and was brought into the emergency room. The patient was stuporous, without focal neurological signs. Blood pressure was 120/80 mmHg and his pulse 80 beats/min. Examination of the head, eyes, ears, nose, and throat was unremarkable. His chest was clear to auscultation and percussion. The remainder of the physical examination was unremarkable. Laboratory investigations showed: serum sodium 137 mmol/L, potassium 4.6 mmol/L, chloride 102 mmol/L, bicarbonate 15 mmol/L, urea nitrogen 43 mg/dL, creatinine 1.0 mg/dL, and glucose 200 mg/dL. Serum ketones were trace positive and serum osmolality was 330 mOsm/kg H 2 O. Blood pH was 7.32 and PCO 2 was 28 mmHg. Urinalysis was negative with a pH of 5.1.
What acid-base disorder is present?
- A.
High anion gap metabolic acidosis
- B.
Mixed reparatory acidosis and high anion gap metabolic acidosis
- C.
Mixed reparatory alkalosis and high anion gap metabolic acidosis
- D.
Chronic respiratory alkalosis
The correct answer is A
Comment: A low plasma bicarbonate concentration and a low pH signify the presence of metabolic acidosis. The PCO 2 is depressed by 10 mmHg and the plasma bicarbonate concentration by 9 mEq/L. This level of respiratory compensation is appropriate for the steady state level of bicarbonate. The fall in plasma bicarbonate concentration is matched by a commensurate rise in the unmeasured anion concentration; thus, this is a pure high anion gap type of metabolic acidosis.
Under normal conditions, the osmolar gap, difference between the measured and the estimated serum osmolality (2 × sodium concentration, mEq/L) + (BUN, 2.8 mg/dL) + (glucose concentration, 18 mg/dL), is less than 10 mOsm/kg H 2 O. As a general rule, a value greater than this indicates the presence of an additional osmotically active substance in the circulation. Methanol intoxication, ethylene glycol intoxication, and alcohol ketoacidosis are disorders associated with high anion gap metabolic acidosis and increased serum osmolality. The measured serum osmolality in this patient was 330 mOsm/kg/H 2 O, giving an osmolal gap of 30 mOsm/kg H 2 O. The patient had no oxalate crystals in the urine, as found in ethylene glycol intoxication, and there was no evidence of optic papillitis on funduscopic examination, as might be anticipated in a patient with methanol intoxication. Blood alcohol levels were very high, indicating the diagnosis of alcoholic ketoacidosis.
Disorders producing metabolic acidosis and elevated anion gap include methanol intoxication, ethylene glycol intoxication, and alcohol ketoacidosis, and one must utilize the history, physical examination, and supporting laboratory data to identify the specific cause when it is not apparent. Patients should be questioned specifically about a history of diabetes, renal disease, and alcohol or other drug ingestions. The physical examination provides important information regarding tissue perfusion and mental status. The presence of acidosis and an increased anion gap in a patient in shock most often is due to lactic acidosis. In a patient with unexplained metabolic acidosis, the degree to which the anion gap is elevated can be helpful in identifying the cause. Patients who have sniffed solvents or who are intoxicated with salicylates commonly have anion gaps of 17 to 19 mEq/L. Patients with the other disorders listed usually have anion gaps between 20 and 30 mEq/L. Severe acidemia, a pH less than 7.00, occurs most commonly in diabetic ketoacidosis, lactic acidosis, and methanol or ethylene glycol intoxication. Hyperglycemia signifies diabetic ketoacidosis or hyperglycemic hyperosmolar coma. Hyperglycemia and glycosuria also can occur in salicylate intoxication. Conversely, hypoglycemia should suggest alcoholic ketoacidosis, because serum glucose levels are lower than 50 mg/dL in about 13% of patients with this disorder. Ketonuria occurs almost invariably in diabetic ketoacidosis, commonly in alcoholic ketoacidosis and in approximately 25% of patients with salicylic intoxication. In patients with ethylene glycol intoxication, the urine sediment can contain many calcium oxalate crystals. In addition to the value of finding an elevated anion gap, the relationship between the increase in the anion gap and the fall in plasma bicarbonate concentration may also be helpful diagnostically. As a rule, with metabolic acidosis of the high anion gap variety, there should be 1:1 correspondence between decreasing serum bicarbonate and increasing anion gap. Whenever the anion gap is elevated but serum bicarbonate is not reduced reciprocally, a mixed metabolic acidosis and alkalosis must be strongly considered.
Calculation of the osmolar gap may be helpful, particularly if methanol and ethylene glycol levels cannot be determined rapidly. The osmolar gap is defined as the difference between the measured and the calculated osmolality. A discrepancy of 10 mOsmol or more between measured and calculated osmolality suggests the presence of an osmotically active substance in the plasma that is not measured and, thus, not included in the calculation. Such an elevation of the osmolar gap occurs in methanol, ethanol, and ethylene glycol intoxication.
Case Study 20
A 12-year-old girl was rushed to the emergency department following a motor vehicle accident and was found to have stroke as a result of left parietal hemorrhage. Her weight was 45 kg (50th percentile), height 139 cm (10th percentile). The vital signs were temperature 38.6°C, blood pressure 120/78 mmHg (90th percentile), pulse 71 beats/min, and respiratory rates 29 breaths/min. Her breathing was rapid and deep. Her chest was clear to auscultation and percussion. Abdomen was soft and non-tender. The Glasgow coma scale estimated between 9 and 12. The remainder of the physical examination was normal.
Serum Na + was 140 mmol/L (reference: 135 to 145 mmol/L), K + 3.0 mmol/L (reference: 4 to 5 mmol/L), Cl − 113 mmol/L (reference: 105 to 110 mmol/L), bicarbonate 18 mEq/L normal range 22 to 26 mEq/L), and anion gap 9.0 mmol/L [(Na + ) − (Cl − + HCO 3 − )] (normal range 8 to 16 mmol/L). Urinalysis revealed a pH of 5.5, specific gravity 1.018 without protein or blood. Urine pH was 5.7, Na + 52 mmol/L, K + 41 mmol/L, Cl − 77 mmol/L, and UAG 16 mmol/L (Na + + K + ) − (Cl − ).
Serum creatinine was 0.8 mg/dL (normal range 0.5 to 1.0 mg/dL) and eGFR was 101 mL/min/1.73 m 2 (normal 95 to 101 mL/min/1.72 m 2 ). The positive UAG in the clinical setting of hyperventilation suggested CRA as the likely diagnosis. The diagnosis was later confirmed by obtaining capillary blood gas, which showed a decrease in PCO 2 and high normal pH values.
What acid-base disturbance is present and what is the MOST likely explanation for the development of positive UAG?
- A.
Chronic respiratory alkalosis (CRA)
- B.
Type-1 distal renal tubular acidosis (dRTA-1)
- C.
Mixed respiratory alkalosis and dRTA
- D.
None of the above
The correct answer is A
Comment: In evaluation of patients with low serum bicarbonate concentration, CRA and dRTA-1 are often within the spectrum of differential diagnoses. CRA can present with a picture that is similar to dRTA-1. Failure to diagnose CRA can lead to inappropriate alkali therapy, which, in turn, may cause cerebral vasoconstriction and hypocalcemia, both of which could cause seizure and increase the morbidity and mortality of the underlying disease.
In our patient, the constellation of hyperventilation, low serum bicarbonate concentration, and positive UAG helped to distinguish CRA from MA even in the absence of arterial blood gas analysis.
The use of UAG as a surrogate of ammonium (NH 4 + ) excretion may differentiate between CRA and MA especially when arterial blood gas is not obtained. When the clinical history (hyperventilation) supports CRA, urine pH above 5.5 in non-gap acidosis does not necessarily suggest a primary defect in H + secretion such as dRTA-1 as CRA also suppresses renal free H + excretion, maintaining a positive UAG and causing urine pH above 5.5 as our patient illustrates.
The UAG represents the differences between the principal cations (Na + + K + ) and anion (Cl − ) and is used as a surrogate of renal NH 4 excretion. In the setting of CRA distal ammonia (NH 3 ) excretion increases to the extent that the secreted H + is almost completely buffered as NH 4 + . This may result in a very low free H + in the lumen to lower the urine pH below 5.5.
In simple respiratory alkalosis, the decline in bicarbonate concentration is a proportional response to the decrement in PCO 2 . The expected serum bicarbonate for a PCO 2 between 17 and 25 mmHg among our patients closely approximates the expected renal compensation, signifying the presence of pure CRA, excluding the diagnosis of mixed acid-base disorders.
Although a positive UAG in a setting of low serum bicarbonate concentration may support the diagnosis of CRA, in the absence of blood gas results, history of hyperventilation and clinical findings of hyperpnea are necessary underpinnings to diagnosis and with positive UAG providing support and guidance. Treatment of the underlying illness usually improves CRA and metabolic treatment is generally not indicated. Rebreathing into a closed system, anxiolytic medications, or opioids have been used rarely to slow respiration.
References
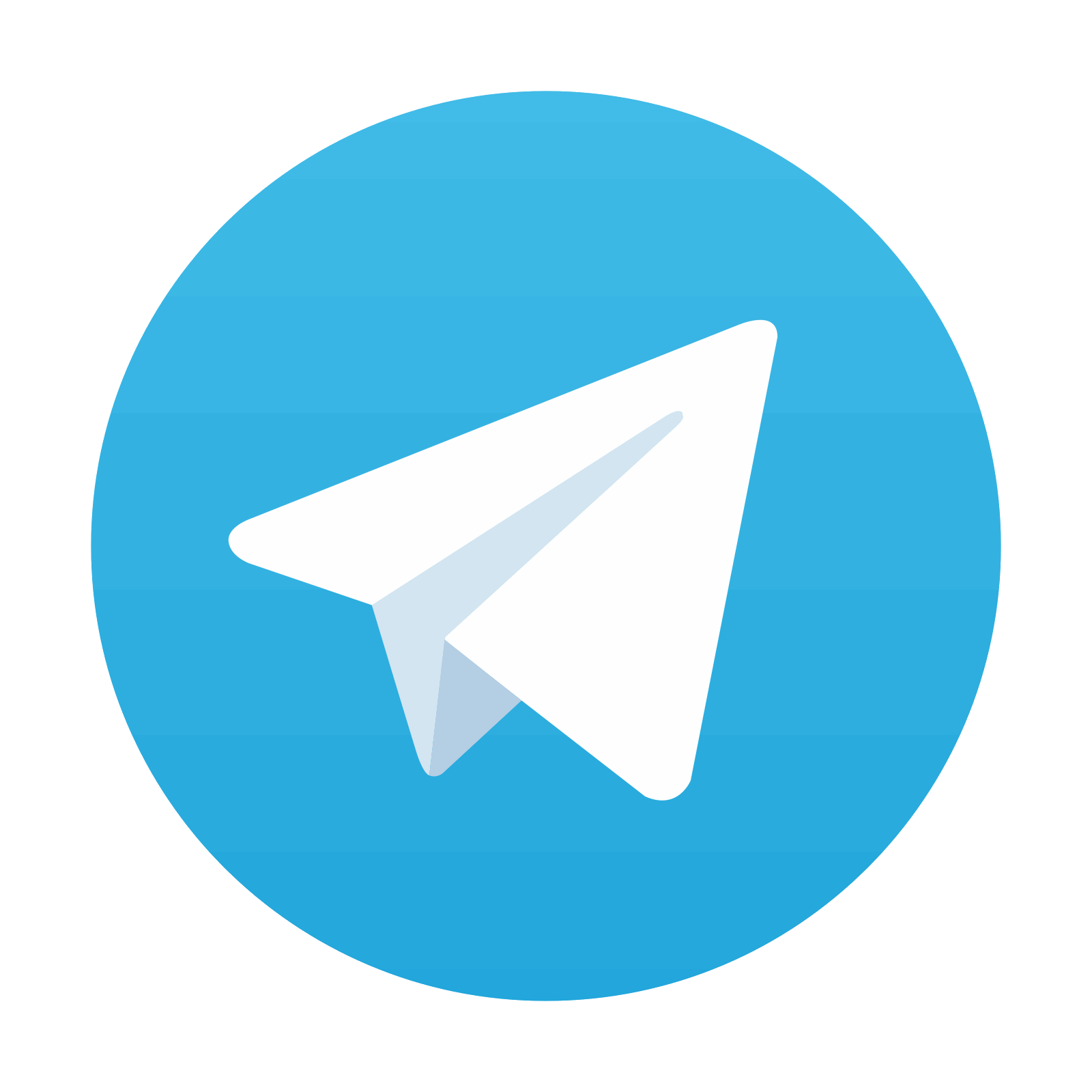
Stay updated, free articles. Join our Telegram channel

Full access? Get Clinical Tree
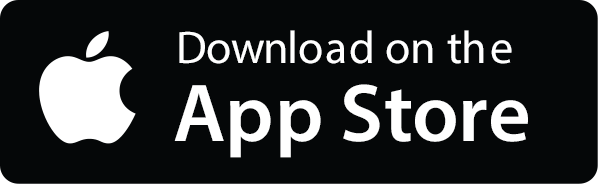
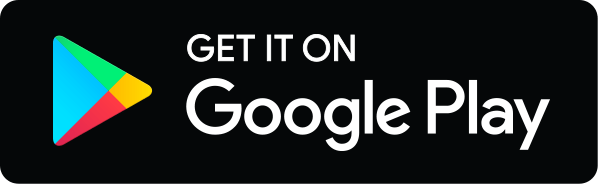
