Fig. 13.1
Red blood cells of a patient with sickle-cell disease. Left: Oxygenated. Right: Deoxygenated. The shape of the cells when deoxygenated causes them to break easily. [Courtesy of Dr. Anthony C. Allison.]
Twenty-five percent of sickle cell patients have compound heterozygosity of HbS with another β-globin chain variant, such as hemoglobin C, D-Punjab, O-Arab, and E. The third form of SCD occurs when HbS is inherited with a β-thalassemia allele, causing HbS/β-thalassemia.
Epidemiology
Historically, SCA have been shown to prevail in malarious areas, since HbS carries a selective advantage against malaria. Subsequent migration from malaria-endemic regions lead to the widespread SCA [2].
SCD is the most common inherited blood disorder in the United States. It is responsible for approximately 113,000 hospitalizations and $488 million dollars in hospitalization costs annually. It is estimated that 90,000–100,000 Americans of all races suffer from SCD. SCD predominantly affect African-Americans where 1 in 13 have sickle trait (heterozygous) and 1 in 365 have SCA [3].
Pathophysiology
SCD manifestations are driven by two major pathophysiological processes : vaso-occlusion with ischemia–reperfusion injury and hemolytic anemia.
Acute vaso-occlusive pain is thought to be caused by entrapment of erythrocytes and leucocytes in the microcirculation, causing vascular obstruction and tissue ischemia. Although this process requires HbS polymerization, the event that triggers the vascular obstruction by sickle erythrocytes is often inflammatory where hypoxia induces endothelial–leucocyte–erythrocyte adhesive interactions in the post-capillary venules. Vascular occlusion is the result of a dynamic interaction between erythrocytes and the vascular endothelium, resulting in episodic microvascular occlusion and ischemia, followed by restoration of blood flow, which further promotes tissue injury mediated by reperfusion. Necrotic marrow fat and cellular elements (Fat emboli) contribute to vascular occlusion, particularly in the lungs, where it causes acute chest syndrome.
The second pathophysiological process in sickle-cell disease is hemolytic anemia, which is also driven by HbS polymerization. Hemolysis cause anemia, fatigue, cholelithiasis, and more importantly contributes to the development of progressive vasculopathy. Therefore, patients develop pulmonary hypertension, cutaneous leg ulceration, priapism, endothelial dysfunction, cerebrovascular events, and proliferative changes in the intima and smooth muscle of blood vessels (Fig. 13.2) [4].


Fig. 13.2
Pathophysiology of sickle cell disease
Diagnosis and Screening
Diagnosis of SCD is based on analysis of hemoglobin. Typically, this analysis involves protein electrophoresis or chromatography, which are inexpensive techniques and widely available worldwide. Antenatal screening is available to women in some countries to help identify couples who are at risk of having a baby with SCD [5].
Management by Complications
Anemia
SCD produces a chronic, compensated hemolytic anemia with episodic acute drops in hemoglobin leading to symptomatic anemia. Other contributing factors to chronic anemia include inappropriately low serum erythropoietin (EPO) concentration (due to renal disease or increased plasma viscosity) and/or folate or iron deficiency.
Chronic Hemolytic Anemia
Sickled cells undergo hemolysis , with a typical red blood cell (RBC) lifespan of approximately 17 days (one-seventh that of normal RBCs), leading to chronic hemolytic anemia. Blood counts will show a hemoglobin baseline level between 8 and 10 g/dL (hematocrit 20–30%). High MCV can be seen if the patient has significant reticulocytosis or is taking hydroxyurea. White blood cells (WBC) are mildly increased and peripheral smears may show abundant reticulocytosis (3–15%), sickled cells (drepanocytes), and Howell Jolly bodies (nuclear remnants that have not been phagocytosed due to reduced splenic function). Other findings related to hemolysis include elevated serum lactate dehydrogenase (LDH) and low serum haptoglobin [6].
Aplastic Crisis
Aplastic crisis is characterized by an acute drop in hemoglobin level caused by a transient arrest of erythropoiesis, leading to abrupt reductions in red cell precursors in the bone marrow and a markedly reduced number of reticulocytes in the peripheral blood (<1.0%). Although TAC usually manifests as a pure red cell aplasia, white cell and platelet counts may also decline resulting in pancytopenia. Management is with transfusion for hemoglobin close to the known patient baseline. Eighty percent of SCD patients who develop TAC were found to be secondary to acute parvovirus [discussed in more detail under section “Infections”] [7].
Splenic Sequestration Crisis
Splenic sequestration crisis is characterized by an acute drop in hemoglobin level caused by vasoocclusion within the spleen and splenic pooling of RBCs. A large percentage of blood can become sequestered in the spleen, leading to hypovolemic shock and death. Patients with splenic sequestration crisis present with a rapidly enlarging spleen and a marked decrease in hemoglobin level despite persistent reticulocytosis. The mortality rate is as high as 10–15% with up to 50% of the patients having recurrent episodes. Treatment requires, immediate diagnosis with swift volume resuscitation using intravenous normal saline and blood transfusions. Splenectomy is often used after the first acute event to prevent recurrence [8].
Hyper-hemolytic Crisis
Hyper-hemolytic crisis refers to the sudden exacerbation of hemolysis with worsening anemia despite ongoing reticulocyte production. This complication is rare and the causes are unknown. Some believe it is a form of a delayed hemolytic transfusion reaction. Infections and/or drug exposure may be responsible for increased hemolysis in some cases. In a study, 7/8 children with hyper-hemolytic crisis had associated glucose-6-phosphate dehydrogenase (G6PD) deficiency.
Some reports have noted improvement with immunosuppressive therapies including intravenous immune globulin (IVIG) plus glucocorticoids. Rituximab also has been used with some success. The successful use of immunosuppressive agents hint at an underlying antibody-mediated etiology for the hemolysis [9].
Acute VASO-Occlusive Pain Crisis
Vaso-occlusive crises are recurrent episodes of severe pain in sickle cell disease. The first episode may occur as early as 6 months of age, often presenting as dactylitis (vasoocclusive pain in the small bones of the hands and feet), and thereafter may occur with variable frequency, usually in the extremities, chest, and back.
Epidemiological studies of the frequency and severity of vaso-occlusive crises indicate an association with high concentrations of HbS, low concentrations of HbF, and high steady-state leukocyte counts and hemoglobin levels.
Many patients have specific triggers for pain such as cold, wind, low humidity, dehydration, stress, alcohol, and menses, which they then develop strategies to minimize or avoid. However, most painful episodes have no identifiable cause.
Although acute vaso-occlusive pain is typically self-limiting and does not result in permanent organ damage, it is the most important complication from the patient’s perspective, and increased frequency of pain is associated with early death and the risk for other complications such as acute chest syndrome (ACS), requiring rapid triage, evaluation, and administration of analgesics. Patients with SCD experiencing acute pain crises may be incorrectly identified as those with drug-seeking behavior or addiction.
Appropriate opioid agents include morphine hydromorphone, or fentanyl. Medication can be provided by intermittent dosing or continuous infusion. Of these, continuous infusion by patient-controlled analgesia (PCA) is preferred because it provides the most even therapy with the patient in the therapeutic window of pain control without side effects, along with the option for self-administration of additional doses for breakthrough pain. Importantly, as-needed (PRN) pain medication as the sole source of pain relief should be avoided based on the pharmacological principle that the patient will have sub-therapeutic pain medication levels resulting in unnecessary pain [10].
For individuals receiving chronic hydroxyurea therapy, hydroxyurea should be continued at the patient’s regular dose during acute events including hospitalizations, unless there is a hydroxyurea-induced cytopenia or other laboratory abnormalities. For individuals not receiving hydroxyurea at the time of hospitalization, the possibility of starting hydroxyurea to reduce future pain episodes generally is discussed with the primary hematologist on an outpatient basis when the patient is feeling well; the benefits of hydroxyurea for preventing pain typically do not occur until at least 3 months after starting therapy.
During painful episodes, individuals with SCD are frequently hypovolemic. An initial intravenous (IV) bolus of 0.5–1 L of normal saline should be administered thereafter maintenance IV fluids along with PO fluid intake should be established. A non-RCT study in 1971 suggested that treatment of sickle cell crisis with rapid infusion of hypotonic saline can lower serum osmolality, decreases pain and is well tolerated. However, high-quality evidence to support a specific route, type, or quantity of hydration is lacking [11].
Infections
Bacterial infections are a major cause of morbidity and mortality in patients with SCD. Mechanisms include functional hyposplenism or asplenism, reduced tissue perfusion, presence of an indwelling catheter (e.g., for chronic transfusion), splinting, and hypoventilation.
Functional hyposplenism develops in early childhood (often starting as early as 4 months of age), and infants and young children are at greatest risk of certain infections. Splenic auto-infarction typically renders patients functionally asplenic by 2–4 years of age, which greatly increases the risk of serious infection with encapsulated organisms.
Most reported sites of infection include bacteremia, meningitis, osteomyelitis, bone marrow (especially by Parvovirus B19), and pulmonary infections (pneumonia and acute chest syndrome [ACS]).
Meningitis has been associated with SCD given the prevalence of encapsulated organism infections [12].
Parvovirus B19 infection which invades proliferating erythroid progenitors leading to transient arrest of erythropoiesis (Transient aplastic crisis) is a relatively common event for patients with SCD. Parvovirus B19 infection has an incidence of 11.3 per 100 patient-years with transient aplastic crisis occurring in 65–80% of them [12].
The incidence of osteomyelitis is also increased in individuals with SCD. Long bones are usually affected, often at multiple sites, resulting from infection of infarcted bone. The most common organisms are Salmonella species. Diagnosis is stablished by biopsy or aspiration of a suspected locus of infection by imaging. Plain films, bone scintigram, and MRI can all be used to find a locus of infection.
Prevention of infections is crucial in decreasing morbidity and mortality in SCD patients. Two major measures for preventing infection in individuals with SCD are immunization for all patients, and prophylactic penicillin (prophylaxis is done up to age 5). All patients with SCD should be immunized with both pneumococcal conjugate vaccine (PCV13) and pneumococcal polysaccharide vaccine (PPSV23). The United States Advisory Committee on Immunization Practices (ACIP) recommends revaccination with PPSV23 every 5 years. All patients should also receive H. Flu type b (Hib) conjugate vaccine, meningococcal vaccine and, yearly influenza vaccine [12].
Fever or rigors is usually the first sign of bacteremia which might lead to fulminant septicemia. Asplenic individuals, especially those who no longer take daily prophylactic antibiotics, should self-medicate with high-dose oral antibiotics. These patients should have prescribed antibiotics on hand and take them immediately. Amoxicillin-clavulanate is the drug of choice or extended-spectrum fluoroquinolones for penicillin-allergic patients. This an acceptable approach up until the age of 18 then antibiotics should be used cautiously and only after proving the patient has an active infection as the risk of pneumococcal infection reduces significantly with age: 9.8 per 100 patients before age 5 to 0.67 per 100 patients after age 5. Furthermore, long-term penicillin prophylaxis is not without its problems. Prolonged or intermittent antibiotic use can promote the development of resistance, so the recommendation to continue must represent a balance between the risk to the individual of pneumococcal infection versus the danger resistant organisms pose to the whole population [12].
Neurological Complications
Stroke is a common and potentially devastating manifestation of sickle cell disease that can affect both children and adults. It is usually seen with SCA (HbSS). Many cases are associated with vasculopathy affecting the distal internal carotid and middle cerebral arteries. Although the mechanisms for stroke remain uncertain, contributory factors to this vasculopathy include anemia, leukocytosis, hypoxemia, functional nitric oxide deficiency associated with hemolysis, and impaired regulation of blood flow causing hyperemia. The vasculopathy seems to start in infancy, with 11% of patients with SCD have had a stroke by the age of 20 years and 25% by age 45. Once a stroke has occurred, the risk of recurrence is more than 60% [13].
Vasculopathy can be detected at an early stage by use of transcranial doppler (TCD) ultrasonography. TCD is done on the distal internal carotid artery or proximal middle cerebral artery. High-risk patients were identified as patient that exhibit high TCD flow velocities (≥200 cm/s) since their stroke risk was up to 40%. The Stroke Prevention in Sickle Cell Anemia (STOP) study, done in pediatric population 2–16 years of age, showed that regular blood transfusion (both simple and exchange transfusions were used) to keep HbS below 30% reduced the risk of stroke by 90% in high-risk patients. The study also showed that patients on transfusion therapy were more likely to have normal TCD results. Transfusions did however result in iron overload and alloimmunization, but not more frequent infections. A follow-up study (STOP II) was done based on the hypothesis that the former patients were potential candidates for stopping transfusion; unfortunately, this did not prove to be the case. Discontinuation of transfusion after at least 30 months in patients who have converted to normal TCD velocities was associated with high risk of reversion to abnormal and chance of stroke [13].
The role of hydroxyurea and phlebotomy was studied in the Stroke with Transfusions Changing to Hydroxyurea (SWiTCH) study . SWiTCH compared the efficacy of regular blood transfusions and iron chelation with hydroxyurea and phlebotomy in children with SCD and stroke. However, the study was stopped early due to the high number of strokes in the hydroxyurea group; no strokes occurred in patients receiving blood transfusions [14].
Primary prevention to reduce the risk of a first stroke is based on the use of regular transcranial Doppler measurements for risk stratification. Screening beyond the age of 16 years is not recommended given the changing patterns of cerebral blood flow velocities above this age.
High-risk patients are treated with chronic prophylactic transfusion with a goal to lower and maintain HbS level at ≤30% of total hemoglobin, while maintaining a pre-transfusion hemoglobin concentration of approximately 9 g/dL or 27% hematocrit and up to 10 g/dL in chronically transfused patients.
New studies have showed that allogenic stem cell transplant allowed the safe stop of all transfusions in all patients, even in those with abnormal TCD velocities prior to transplant [15].
Intracranial bleeds occur in patients of all ages, but are most common between the ages of 20 years and 30 years; they are typically associated with either a moyamoya-like syndrome or cerebral aneurysms. Treatment is neurosurgical and outcomes are generally poor.
In patients with radiologically confirmed acute ischemic stroke, transfusion therapy should be initiated promptly. The goal is to lower the percentage of HbS to ≤30% of total hemoglobin and to aim for a total hemoglobin level of ≤10 g/dL. Both simple and exchange transfusion can be used. Thrombolytic therapy is not generally used, although there are no good quality data to guide practice. Antiplatelet therapy with aspirin is a mainstay of treatment in patients with SCD who have an acute ischemic stroke [16].
Acute Chest Syndrome
Acute chest syndrome (ACS) is defined as new infiltrate involving at least one complete lung segment (usually predominate the lower lobes), that is accompanied by fever, chest pain, tachypnea, wheezing, and/or cough in a patient with SCD. Pain crisis is a prodrome of the ACS; ACS usually occurs 24–72 h after the onset of severe pain in the arms, legs, or chest.
ACS is a leading cause of morbidity and mortality in patients with SCD. The Cooperative Study of Sickle Cell Disease (CSSCD), the largest natural history study of SCD (939 patients) found that approximately 50% of patients with SCD will have an ACS. While the etiology of respiratory dysfunction in SCD is multifactorial, pulmonary embolism (PE) and infection contribute to ACS in a significant proportion of cases. The National Acute Chest Syndrome Study Group (the largest study on ACS with 538 patients) noted that pulmonary infections by a community-acquired pathogen was identified in 54% of patients with ACS. Chlamydia was the most commonly isolated infective organism associated with ACS, although the rate of infection with this organism was similar to that in the general population. PE (both venous thromboembolism (VTE) and fat embolism) were the second most common cause of ACS in 17% of episodes.
To minimize the risk of ACS in SCD patients, influenza and pneumococcal vaccines should be administered (as discussed earlier). Furthermore, those admitted for painful crises should be considered to be in the prodromal phase of ASC; they require incentive spirometry and daily monitoring for pulmonary disease.
Once ACS has been diagnosed, broad-spectrum antibiotics (including a macrolide) are required. Given that bronchodilator therapy has shown to be effective in improving pulmonary function in ACS patients, they should be initiated even if airway hyper-reactivity is not evident. Routine, early exchange or simple transfusions have shown to improve oxygenation in ACS and are indicated for patients at high risk for complications, including adults and those who have a history of cardiac disease and severe pain in the arms and legs at presentation. Those who present with severe anemia and thrombocytopenia should receive exchange transfusion before respiratory distress (ARDS) develops. New data suggest that corticosteroids and nitric oxide are beneficial in severe cases that have not responded to other treatments [17, 18].
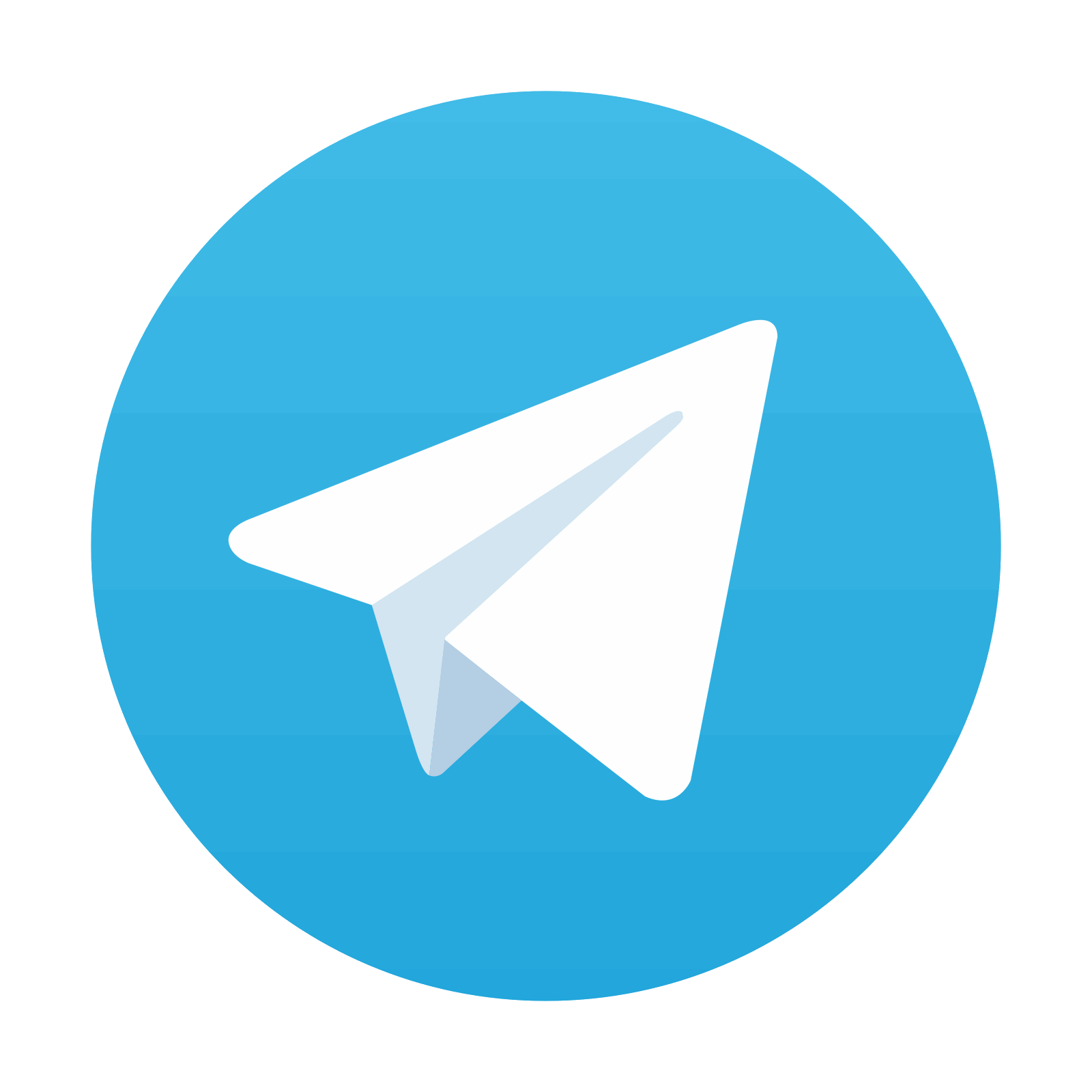
Stay updated, free articles. Join our Telegram channel

Full access? Get Clinical Tree
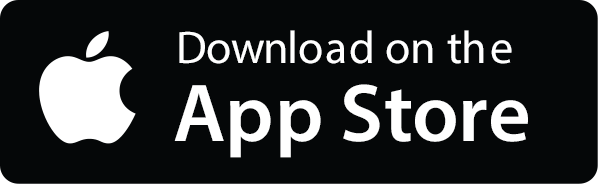
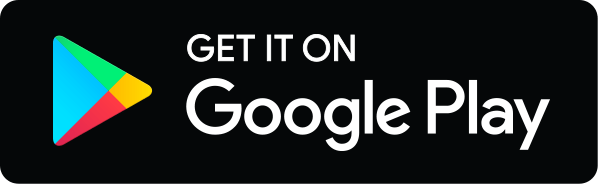