Lower extremity injuries represent the primary cause of more than half of all hospitalizations for trauma. Their frequency, severity, and costs emphasize the impact of those injuries on society.1,2 Lower extremity fractures may be caused by either low- or high-energy forces and occur both in isolation and as multiple injuries. The mechanism of injury defines the specific individual fracture pattern. Typical trauma mechanisms include blunt versus penetrating trauma, low-energy versus high-energy forces, twisting, bending, or crushing forces. Significant lower extremity injuries compromise functional outcome and can lead to long-term pain, abnormal gait, degenerative joint disease, chronic infection, and limb loss.
Dislocations of the hip, knee, or more distal joints, as well as displaced fractures, may cause pressure on nerves, vessels, or skin that can result in permanent deficits if not dealt promptly. Many of these injuries are either surgical emergencies or, at the very least, require urgent treatment in the operating room. Failure to recognize the significance of these injuries can lead to sequela as significant as amputation or death. While not true emergencies, open fractures of the lower extremities require timely surgical treatment to minimize the risk of infection and limb loss.
The wide prevalence of safety belt usage and changes in vehicular design such as crumple zones and mandatory airbags has led to an increased number of survivors of high-energy crashes who consequently suffer from a higher severity of lower extremity injuries. For example, Shock Trauma in Baltimore noted a drop in the mortality associated with bilateral femur fractures from 26 to 7% over a 15-year period. There was an associated drop in Injury Severity Score (ISS) that suggested a contribution to this decrease in mortality from changes in motor vehicles.3 Any trauma victim involved in a high-energy trauma mechanism may have associated potentially life-threatening injuries to the head and torso. Thus, the initial evaluation of lower extremity fractures must focus on the patient as a whole, and not focus exclusively on the injured limb.4,5,6
The concept of “damage control orthopedics” (DCO) was established based on the principle that prolonged early definitive treatment of long bone fractures can be detrimental for severely injured patients who are in unstable physiological conditions.7,8 In these patients, the early mitigation of the “lethal triad” of persistent metabolic acidosis, hypothermia, and coagulopathy represents the prime goal for survival.4 More recently, however, our ability to resuscitate patients has improved, and multiple high-powered studies have shown improved outcomes while utilizing an “early total care” (ETC) model. In resuscitated patients, definitive intramedullary nailing of femur fractures and open fixation of pelvic and acetabular fractures has proceeded within 24–36 hours after injury with subsequently shorter ICU stays and fewer pulmonary complications, all at an overall lower cost.9,10,11,12 The controversial concept of “limb for life” entails the early amputation of a mangled lower extremity in critically injured patients with the aim of increasing the likelihood of survival.
A relatively recent concept in lower extremity fracture care is that the majority of fractures can be treated entirely or in part with minimally invasive fixation. The evolution of techniques for percutaneous reduction and fixation of fractures, coupled with technological adaptation of fracture implants, has completely revolutionized fracture fixation.13 While intra-articular fractures usually require some form of open reduction to restore articular congruity, most diaphyseal and metaphyseal lower extremity fractures can be treated with minimally invasive surgery. The decreased blood loss, lowered risk of infection, and increased rate of healing likely have positive implications for the injured patient with lower extremity fractures.
Orthopedic surgery has developed through the need to alleviate pain, correct deformity, and restore function following fractures. Evidence of splinted fractures and the first successful amputations dates as far back as the fifth Egyptian Dynasty, about 4500 years ago. The Corpus Hippocrates described the principles of traction, countertraction, and external fixation. Surgeons have built on these past foundations with the advancement of technology. In England, Thomas described the traction splint that still bears his name. In France, Malgaigne described the external fixator, and Delbet reported use of a weight-bearing cast for tibial fractures. In the United States, Buck described skin traction, while Steinmann in Switzerland and Kirschner in Germany introduced skeletal traction. Another German, Küntscher, made many contributions to modern intramedullary nailing. In Austria, Böhler established hospitals devoted to the care of injuries and published a comprehensive text on fracture surgery. Lambotte, a Belgian, is the father of modern internal fixation, which was advanced further by his countryman, Danis, who demonstrated that rigid fixation could result in direct bone healing without callus formation. The Swiss-based “Arbeitsgemeinschaft für Osteosynthesefragen” or “Association for the Study of Internal Fixation” (AO/ASIF) was founded in 1958 by a group of Swiss surgeons to produce and disseminate a system of fracture care based on stable fixation with preservation of soft tissue, active motion, and functional rehabilitation.14 This association has earned itself a worldwide reputation as an international authority in the treatment of trauma through its continuing research and development of instrumentation. Further advances continue, with emphasis on indirect reduction techniques, closed or minimally invasive fracture fixation, and stable but less rigid fixation that promotes rather than suppresses indirect, callus-dependent healing of bone.13
At about the same time in the Soviet Union, Professor Ilizarov, working in the Siberian town of Kurgan, developed and refined the concept of distraction osteogenesis, permitting healthy de novo bone to be created in vivo through distraction with a ring external fixator system with Kirschner-type wires. His work led to significant advances in the use of external fixators as definitive treatment for a variety of traumatic injuries and post-traumatic complications.
The increasing ability of orthopedic surgeons to obtain early fracture stability with relatively low complication rates has led to improvements in post-injury rehabilitation. Rehabilitation concepts have changed from the prolonged rest suggested by Thomas to the present emphasis on rapid restoration of skeletal stability that allows for prompt mobilization of injured extremities and patients. Early weight bearing is encouraged, whenever possible to promote bone healing and overall physiological restoration. Detailed knowledge of a patient’s musculoskeletal injuries, his or her treatment, and his or her response is crucial for appropriate decision making in both the acute and late phases of care. Therefore, the orthopedic surgeon should ideally be directly involved from the trauma bay to the entire recovery process.
Fractures occur when the applied load to the bone exceeds its load-bearing capacity. Fracture patterns relate to bone strength and the forces that cause the injury. Young, active individuals have strong bone. Children’s bones can undergo plastic deformation and may bend without breaking. Elderly, osteoporotic individuals have diffusely weak bone. Focal bone defects may weaken a bone so significantly that it fails under a load that would normally pose no problem, resulting in a pathologic fracture. Such pathologic fractures may be due to tumor, infection, or dysplasia, as well as more generalized conditions that severely weaken bone, such as osteoporosis. The amount of energy that produced a given fracture is suggested by the patient’s history and the fracture pattern. Basic physics is implied: kinetic energy equals ½(mass)(velocity)2. Thus, the greater the velocity is, an exponentially higher amount of energy is stored within a system. Upon impact, that energy is absorbed by the body and the musculoskeletal system. This energy is realized as comminution (multi-fragmentary fractures) and local damage to soft tissue.
The impact to a bone may be a bending or twisting moment, or it may result from a pointed impact such as a projectile. Bones are weakest in torsion, and spiral fractures result from torsional forces. Less local soft tissue damage is generally present. Transverse fractures result from directly applied forces where the bone is “bent” over an object or fails under off-axis loading. Bending moments can also cause wedge or “butterfly” fragments formation. When a bending moment is applied to a bone, there is a resultant compressive force on the bone closest to the concavity of the bend and a reciprocal tension on the convex side. Bone initially fails in tension, and as the fracture propagates toward the concavity, the fracture will move around the compressed bone both proximal and distal to it, creating a wedge-shaped fragment.
Obtaining a thorough patient history provides the physician with useful information to begin forming a list of differential diagnoses in his or her mind prior to radiographic examination of the patient. The history should specify the mechanism of injury, provide information regarding the severity of the applied forces, and alert the physician to associated injuries, illness, or medically relevant problems. While an accurate history may be difficult or impossible to obtain initially in a seriously injured patient, more details should always be sought and reconfirmed as the patient improves or more information becomes available. The history may be particularly helpful in managing open fractures by providing information on the following: the identification of the source and extent of contamination, the time elapsed from the moment of injury, and whether bone was initially protruding from an extremity wound.
A history inconsistent with the extent of injury suggests either a pathologic fracture or the possibility of abuse. A normal child, particularly less than 2 years, should not fracture his or her femur while playing, even roughly, with a friend or parent. An elderly patient will not normally sustain a hip fracture from turning over in bed. Although pathologic fractures should be suspected in a patient with known malignant or metabolic disease and can be preceded by local pain, fractures may occur in completely asymptomatic patients as the initial presentation of an underlying disorder. In a young child, multiple fractures at various stages of healing are pathognomonic of child abuse, the diagnosis and appropriate management of which may be lifesaving. The report of pain or impaired function of an extremity requires careful evaluation to exclude a fracture or injury to a joint, nerves, muscles, or vascular structures.
Examination according to the Advanced Trauma Life Support (ATLS) protocol provides a systematic method of thoroughly examining the trauma patient and minimizing missed injuries.4,15 In addition, the importance of continuous detailed documentation of the physical findings cannot be overemphasized. Patients should be thoroughly examined at multiple time points by multiple members of the clinical team. All assessments should be well-documented for easy comparison. A thorough exam should be revisited when an initially obtunded patient is first extubated. While tenderness, deformity, and swelling almost inevitably occur with fractures or dislocations of the lower extremity, these can easily be masked: an intubated patient cannot complain of pain; and diagnosing deformity or swelling in even a moderately obese patient can be difficult.
That said, many lower extremity injuries are quite obvious. Displaced long bone fractures result in shortening, malrotation, or angulation. Dislocations typically assume characteristic positions but may be masked by associated fractures. Intra-articular injuries usually cause a hemarthrosis unless the joint capsule is disrupted, in which case more diffuse soft tissue swelling occurs about the joint. Instability or abnormal motion when stressing a bone or joint, while it may be difficult to elicit if the region is tender, is particularly important and useful in the anesthetized patient. When in doubt, obtain imaging—a simple x-ray gives a great deal of information. Immediate reduction of dislocated joints and splint placement where applicable reduce pain and blood loss, often restoring circulation to a pulseless extremity in the process.
As per ATLS protocol, an anteroposterior (AP) chest and pelvis, and adequate lateral cervical spine radiographs are indicated early in the evaluation and resuscitation of the injured patient during the primary survey.4,15 The improved imaging capabilities of CT have led to a tendency to skip over the pelvis and cervical spine x-rays. While the cervical spine is better imaged using more advance imaging, a tremendous amount of information can be gleaned form a simple AP pelvis x-ray, and it should not be neglected.
X-rays of injured extremities are of much lower priority and fall into the secondary survey. Resuscitation of the patient should never be delayed or interrupted for x-rays of the extremities. X-rays can be taken after urgent surgical treatment for other life-threatening problems. In the unstable patient, care should be concurrent and not contiguous, implying that x-rays and fracture stabilization can occur concomitantly in the operating theater or resuscitation bay with lifesaving maneuvers such as laparotomy or thoracotomy. If adequate extremity radiographs can be obtained without delaying other essential aspects of the evaluation and treatment of the trauma patient, they can be valuable in making the initial care plan.
Extremity x-rays should include orthogonal views (AP, lateral) of the entire bone in question. Generally speaking, injury films should be obtained prior to reduction and splint placement. Not only is the quality of the image improved without overlying splint material but also knowledge of the injury pattern guides surgical decision making. In the event of vascular compromise upon initial physical examination, reduction should proceed prior to imaging. This is more common with fractures and dislocations about the knee and ankle. After reduction and splinting, the clinical exam should be repeated and x-rays obtained. In the event of a change in exam (new onset vascular or neuropathic compromise) or a failure of reduction, the reduction should be repeated. Certain complex articular fractures are best visualized with computerized tomography (CT) scans. If a patient is hemodynamically stable and requires other CT studies, extremity CTs may be obtained at the same time, although if external fixation is indicated the CT is often more useful after external fixation. Early involvement of the orthopedic surgeon ensures proper imaging and avoidance of unnecessary diagnostic studies.
A high level of suspicion for a significant associated vascular injury, in conjunction with a thorough clinical exam (Table 40-1), should help in the guidance of the acute management of joint dislocations. Any pulse deficit or measurable reduction in arterial pressure index (API), before or after manipulation, must be considered evidence of a vascular injury. The accuracy of pulse examination alone for the detection of an arterial injury is very low. The five clinical hard signs of an arterial injury (see Table 40-1)16 are present in more than two-thirds of all dislocations with an associated significant vascular injury and are of paramount importance in the clinical guidance for decision making related to the acute management concept.16,17,18 In presence of a hard sign of arterial injury, intervention is indicated. In penetrating injuries, exploration can proceed immediately. In a portion of blunt trauma, an angiogram prior to exploration—either preoperatively or on the operating table—should be considered. The majority (>95%) of arterial injuries occur in proximity to the site of the fracture or joint dislocation. Delay in diagnosis secondary to missing a hard sign can result in limb loss. The use of soft signs to detect occult vascular injury is less clear (Table 40-1). The yield of arteriography in the setting of clinical soft signs is very low and the lesions that are typically identified are nonocclusive lesions: intimal flap, contusion, spasm, and pseudoaneurysm. The natural history of these lesions is benign and self-limiting, and they rarely require expensive surgical repair.19,20 In the setting of late presentation, angiography is indicated for surgical planning.21
“Hard signs” | “Soft signs” |
---|---|
Active or pulsatile hemorrhage | Asymmetric extremity blood pressures |
Pulsatile or expanding hematoma | Stable and nonpulsatile hematoma |
Clinical signs of limb ischemia | Proximity of wound to a major vessel |
Diminished or absent pulses | Peripheral neurological deficit |
Bruit or thrill, suggesting AV fistula | Presence of shock/hypotension |
The neurovascular status may be difficult to assess clinically in a severely injured patient. Therefore, a high level of suspicion is required for identifying and treating potentially catastrophic vascular injuries. Capillary filling is not, by itself, adequate clinical evidence of an intact proximal vascular flow. Distal pulses may be present after a significant arterial injury. Perhaps the most familiar arterial injury in the lower extremity involves the popliteal artery in association with knee dislocations or periarticular fracture. Vigilance must be maintained: knee dislocations will often spontaneously reduce, thus appearing relatively benign; and fracture severity may be underappreciated. The popliteal artery is tethered at the adductor hiatus to the distal femur and at the soleus muscle on the proximal tibia, and thus any fracture with significant displacement of the adjacent bone should be checked for vascular injury. In particular, fractures of the medial tibia plateau are often underestimated and should be considered akin to a knee dislocation.21
Late thrombosis of an initially nonocclusive injury may result in limb loss. Frequent assessment of pedal pulses is required for such patients. Any alteration of pedal pulses requires assessment, at least with Doppler pressure measurement. Assessment of ankle systolic blood pressure is an important adjunct to the physical examination. Pressure below 90% of that in the arms or the opposite leg requires prompt evaluation by a vascular surgeon. Doppler sonography or contrast arteriography may be considered if pulses decrease, but should not delay consultation with a trauma surgeon. Risk factors for limb loss include delayed surgery, arterial contusion with consecutive thrombosis, and, most importantly, failed revascularization.
An arterial injury in combination with an orthopedic injury, such as a traumatic joint dislocation or fracture–dislocation, requires a coordinated approach by the acute care surgery and orthopedic teams. Initially, a temporary arterial shunt is placed to restore limb perfusion and minimize tissue ischemia (Fig. 40-1). Frequently, after shunt placement, the limb is pulled out to length and stabilized using external fixation by the orthopedic surgeon. Subsequent to this, arterial repair is completed by autologous repair of the vessel. While these two events can be interchanged, care must be taken to avoid repairing the artery in a shortened position, thus risking damage to the repair when the limb is lengthened and stabilized. Time to revascularization is of utmost concern since delays in excess of 8 hours after injury carry a risk of amputation in excess of 80%. In contrast, successful operative vascular repair within 8 hours of injury yields excellent rates of limb salvage.”
FIGURE 40-1
Limb salvage procedure in a 35-year-old male patient who was involved in a high-speed motorcycle collision. The patient sustained right-side traumatic lower leg amputation (A and B) and a contralateral type IIIC open traumatic “floating knee” injury, with a combination of a displaced intra-articular distal femur fracture and a comminuted proximal tibia fracture. On presentation to the emergency department, the left lower extremity was dysvascular, pulseless, with a pathologic ankle–brachial index (ABI) of 0.5. The patient was immediately taken to the operating room for spanning external fixation of the left knee and vascular repair. Intraoperative shunting was performed to reconstitute temporary blood flow distal to the SFA (C). Successful vascular repair was performed with a saphenous vein graft from the contralateral side. The amputated residual limb on the contralateral side was debrided and successfully closed by a split-thickness skin graft within 2 weeks (D). The patient recovered well from his injuries and was able to ambulate without support within 3 months using a custom-made above-knee prosthesis for the right residual limb, and functional rehabilitation on the left lower extremity.

The neurological status of the extremity should be documented before any definitive treatment, whenever possible. The neurological examination, like the vascular examination, may be unreliable in the severely injured patient or extremity, and it may also be subject to change. While deficits that are specifically dermatomal or isolated to a single motor nerve are more likely real, there can still discrepancies in the exam. The vast majority of acute nerve injuries recover spontaneously, at least partially.
Specific to the lower extremity, the peroneal nerve is prone to injury. While the entire sciatic nerve may be injured in hip dislocations and thigh injuries, frequently the peroneal nerve is injured and the tibial nerve is spared. The peroneal nerve is a smaller nerve with less neuron redundancy, and it is tethered as it passes around the fibular neck, which makes it more prone to traction injuries.22 Dislocations and fractures about the knee can directly affect the common peroneal. These injuries typically manifest as a foot-drop with or without sensory involvement. Because nerves about the foot and ankle are relatively superficial, have less soft tissue padding, and are typically tethered to local structures, lacerations or deformity to the distal leg can easily affect any of the nerves—superficial peroneal, deep peroneal, saphenous, sural, or posterior tibial. These injuries typically manifest with primarily sensory deficits.
Most peripheral nerve injuries of the leg are neurapraxias the result from stretching mechanisms or blast effect secondary to projectiles. Most commonly resolve without intervention. A peripheral nerve injury is assessed and followed using the clinical exam. If there is no recovery, nerve conduction studies within 6–12 weeks after trauma can aid in defining the extent and prognosis of injury. If complete nerve injury is confirmed, depending on the level of the nerve, a delayed nerve repair can be considered. Given that, even with a successful surgery, prognosis is often poor, patient factors such as age, comorbidities, and social situation all play a role in the surgical decision making at this point.
Awareness of typical combinations of lower extremity injuries aids diagnosis and may decrease the risk of missing important injuries. One mechanism can produce several injuries. An unrestrained passenger in a head-on motor vehicle collision may strike his or her knee against the dashboard, sustaining a patellar fracture or injury to knee ligaments, depending on the point of impact. The force indirectly applied along the femur then dislocates the flexed hip, concurrently producing a posterior wall acetabular fracture and/or fracture of the femoral head. Some other associations are as follows:
Femur shaft and pelvis/acetabulum fractures
Femur shaft and femoral neck fractures
Calcaneus and thoracolumbar spine fractures
Thoracic aortic injuries and pelvis fractures
Combined femur/tibia shaft fractures (“floating knees”) and soft tissue or vascular injuries about the knee
Knee dislocation/tibial plateau fracture and neurovascular injury
Fibular neck fracture and peroneal nerve injury
Vigilance for these injury combinations must be maintained. Some can have a very nuanced presentation. For instance, for diagnosing subtle femoral neck fractures that occur in conjunction with femoral shaft fractures, plain radiographs and CT have similarly unimpressive sensitivities (56–64%). Thus, intraoperative and postoperative imaging must be assessed carefully.23 While fractures are often distractingly obvious on x-rays, injuries to joints such as subluxations and even dislocations may easily be overlooked unless one is suspicious.
While most orthopedic injuries frequently take a backseat to other, more critical, injuries, compartment syndrome remains a true surgical emergency. Vigilance must be maintained—while there are certain injuries that commonly cause compartment syndrome, there have been reports of compartment syndrome from all over the body: gluteal muscles, paraspinal muscles, deltoid, thigh, foot, carpal tunnel, abdomen, leg, and forearm. Compartment syndrome can occur in open fractures. The pathophysiology behind compartment syndrome is the same in all cases: there is raised pressure within a confined space that causes permanent and irreversible damage to the contents of the space. It is a circular mechanism in which increased compartment pressures cause decreased local blood flow, resulting in decreased muscle perfusion, which causes an inflammatory release and an increase in capillary permeability, subsequently causing increased muscle swelling, thus further increasing the compartment pressures. Within the first 4 hours the damage is reversible, and after 8 hours the damage becomes irreversible.24
Compartment syndrome can result from either increase in compartment volume—hemorrhage, swelling from traumatized tissue (crush, burn), etc—or it can result from decreased volume, as in the setting of a tight dressing or cast. The most common cause is muscle injury that leads to edema, and there are certain clinical settings in which compartment syndrome is more frequently seen: high energy or crush injuries, segmental or widely displaced fractures—particularly in the forearm or leg, severely comminuted fractures, and patients with impaired sensorium.
The key with the successful treatment of compartment syndrome is making the diagnosis in a timely manner. Certain physical exam findings should trigger an evaluation for compartment syndrome. As seen in Fig. 40-2, fracture blisters, diffuse eccymosis and swelling, and tissue crepitis are all indicative of significant tissue injury. Compartment syndrome should be suspected when pain is disproportionate to the injury and worsens over time and across multiple exams, when there is pain with passive extension of the involved muscle compartment, and when there are paresthesias. Compartment pressure measurement, when utilized, should be performed in all compartments and within 5 cm of the fracture.25 Either an arterial line setup, a slit catheter, or a side-port needle can be used, but one must be cognizant of the fact that the arterial line will read 5–15 mm Hg higher than a side-port needle. Typically, a threshold for diagnosis of within 30 mm Hg of the diastolic blood pressure is used rather than absolute pressures.26,27,28,29 The above aside, there are multiple issues involved that make diagnosing compartment syndrome difficult:
The clinical evaluation between treating physicians can be unreliable.28
One-time compartment pressure measurement has a high false-positive rate.30
Clinical exam and pressure measurements can be unreliable in an obtunded or anesthetized patient.31
FIGURE 40-2
Critical soft tissue injury in a 43-year-old patient who sustained a high-energy proximal tibia fracture after a motorcycle crash. Fracture blisters developed within 24 hours of injury. The critical soft tissue conditions mandated a staged treatment concept by initial spanning external fixation and delayed conversion to internal fixation once the tissue swelling had subsided and the fracture blisters had healed. Despite the high-energy injury pattern and critical soft tissues, this patient did not develop a compartment syndrome and had an uneventful long-term recovery.

One solution is continuous pressure measurement, but this is frequently not available and difficult to perform on all patients.32 What seems to be the most attainable solution is the establishment of an institution-wide protocol that empowers all team members (nurses, residents, faculty, etc) and has rapid escalation when compartment syndrome is suspected.33,34 Regular, documented clinical exams are used for diagnosis with compartment measurements to confirm the clinical decision. Documentation is important both to compare physical examination findings as well as for medicolegal reasons: increasing time from onset of symptoms to the actual fasciotomy is linearly associated with an increase in indemnity payments.35
Fasciotomy techniques differ among the part of the extremity affected, but the goal is always the same. The thigh is typically treated with a single lateral incision; the tibia can be treated with either single or dual incisions, and the foot requires multiple incisions.36 While it is emergent and limb-saving, it should be kept in mind that a fasciotomy is extremely morbid and expensive: tibia fractures are much more likely to become infected or go on to nonunion; there are significant scarring, weakness, and chronic pain issues; and the cost and length of the hospital stay are more than doubled.37,38
Open fractures should be quickly assessed on arrival of the patient in the emergency room. The most important determinant of the patient’s outcome is the time from injury to the administration of appropriate antibiotic coverage.39 The choice of antibiotic depends on the grade of the fracture. The grading systems most often used for open fractures was that described by Gustilo et al40 (Table 40-2). Despite a significant interobserver variability and limitations related to the wide spectrum of open fracture types covered in three categories, the Gustilo classification is very practicable and therefore well accepted among orthopedic surgeons. Newer classification systems, such as the Ganga Hospital Score from India,41 attempt to overcome the shortcomings from the Gustilo classification by increasing the number of categories and correlating the total score with a guideline for treatment. As such, the Ganga Hospital Score for open fractures will mandate treatment protocols including the “Fix and close” protocol, “Fix, Bone Graft and Close” protocol, “Fix and Flap” protocol, or the “Stabilise, Watch, Assess and Reconstruct” protocol.41
Type | Description |
---|---|
I | Clean wound <1 cm, inside-out perforation, little or no contamination, simple fracture pattern |
II | Skin laceration >1 cm, surrounding soft tissue without signs of contusion, vital musculature, moderate to severe fracture instability |
III | Extensive soft tissue damage, wound contamination, exposed bone, marked fracture instability due to comminution or segmental defects |
IIIA | Adequate soft tissue coverage of the fractured bone |
IIIB | Exposed bone with periosteal stripping |
IIIC | Any open fracture with associated arterial injury requiring vascular repair |
Data from Gustilo RB et al. J Trauma. 1984;24(8):742. |
Once the grade of the fracture has been determined, the antibiotic to be administered should be chosen. The higher-grade fractures are considered to have a greater risk of contamination and are thus covered for more bacteria using broad-spectrum antibiotics. For simplicity, open fractures can be divided into two subsets: “Low energy” fractures that are grades I and II, and “high energy” fractures (all grade III fractures). Different antibiotics are given whether a fracture is “low energy” or “high energy.” There can be some blurring of the fracture grade definitions: a segmental tibia fracture that has just a poke-hole in the skin is by strict definition a grade I fracture, but clearly this is a “high energy” fracture. Given the number of physicians who might be part of the emergency center care of an open fracture patient, the authors have found the most effective way to improve adherence to antibiotic guidelines was to simplify it as above.
So, what are the best antibiotics to give for “low energy” and “high energy” fractures? If only it was so simple. There is only moderate consensus on what antibiotic to give: a recent survey of Orthopedic Trauma Association (OTA) members from around the world found that, while 86% of respondents agreed that antibiotics should be administered within one hour of determination of the presence of an open fracture, there was little consensus on what antibiotics to give and for how long to give them.42 Despite concerns regarding efficacy and nephrotoxicity, aminoglycosides were the most commonly prescribed antibiotic. Typically, intravenous antibiotics are given for 48–72 hours, but there is considerable variation among practice habits.42 It is the authors’ opinion that thought should be given to the prevalence of certain bacteria such as Methicillin Resistant Staph aureus (MRSA) within one’s geographic area of practice. For example, the authors practice in South-Central United States in a warm and humid climate with a community prevalence of MRSA of 38%.43 The antibiotic regimen that we have chosen to use is illustrated in Table 40-3. Despite the general consensus to give aminoglycosides, we chose not to do so, opting for greater gram positive bacterial coverage. Given that the most common bacteria is different in different communities, surgeons are encouraged to examine their own region and adjust treatment appropriately. Of note, there are considerations within the table for patients with soil and marine contamination as well as gunshot injuries. While ballistic injuries have historically been considered sterile, examination of our patient base, which includes a large number of ballistic injuries, found a higher than expected rate of infection with MRSA and enterococcus being the most commonly cultures bacteria. We adjusted our antibiotic protocol in response.
Type | Antibiotic | Duration |
---|---|---|
Low energy (I/II)
| Ancef | 48 hours from presentation 24 hours after subsequent intervention |
High energy (IIIA/B/C)
| Vancomycin Cefepime | 48 hours from presentation 24 hours after subsequent intervention |
Soil contamination | Penicillin | Single dose |
Marine contamination | Levaquin | Single dose |
Gunshot injury with fracture | Vancomycin Cefepime | 48 hours from presentation 24 hours after subsequent intervention |
Having given antibiotics, the open fracture wound should be covered with a sterile saline dressing and only examined in the operating room in order to avoid further contamination and soft tissue damage. Extensive exploration or manipulation of exposed bone should not be attempted in the emergency department. Bleeding, even from amputation wounds, can almost always be managed with a pressure dressing. A tourniquet should be reserved for uncontrollable hemorrhage from penetrating lower extremity injuries.44 A “mangled extremity” defines a severely injured limb secondary to trauma in which there is a significant risk of amputation as a potential outcome. More specifically, a functional limb is composed of the following critical elements: skin and subcutaneous tissue, blood vessels, muscles and tendons, bones, joints including cartilage and ligaments, and peripheral nerves. Irreparable injury to one or more elements may significantly impair the function of a limb and lead to disability. A mangled extremity, the most severe form of injury, encompasses significant injury to multiple elements critical to limb function.
In the event of multiple injuries, hemorrhagic shock, prolonged delay to definitive care, and/or risk of death, amputation may be the preferred option: the “limb for life concept.” Once the patient responds to resuscitative efforts, the extremity is carefully examined during the secondary survey. Evaluation focuses on signs of arterial injury, extent of soft tissue and bone injury, and degree of contamination. A search for “hard signs” and “soft signs” (see Table 40-1) of arterial injury is essential since both civilian and combat experiences demonstrate that the risk of limb loss correlates with a delay in revascularization beyond 6 hours. The risk of limb loss is further increased in the setting of ischemia with associated major venous, soft tissue, and muscle injury.45 Since a significant percentage of injuries, particularly those involving the distal extremity and the major joints, are missed during the initial trauma evaluation, repeated exams are essential, especially as the patient’s recovery permits more cooperation. At least one “tertiary” survey is an important part of each significantly injured patient’s diagnostic evaluation.
All open fractures require prompt surgical treatment to reduce the risks of infection, soft tissue damage, and ongoing bleeding. As soon as the patient’s condition permits, radiographs of the injured limb are obtained. Operative care of the open fracture must fit appropriately into the care of the patient’s other problems. This should be done in an operating room with general or regional anesthesia. While previously it was thought that debridement should be performed within six hours of injury, more recent data has shown that time to debridement is not a predictor of infection as long as it is performed within 24 hours of injury.46 A thorough debridement includes the sharp excision of all devascularized muscle, fascia, subcutaneous tissue, skin, and bone, the removal of all foreign material, and the copious irrigation of the wound bed using low pressure fluid lavage.47 Pulse lavage and antibiotics in the irrigant are generally avoided as they have been found to damage tissue unnecessarily.47
In the setting of a “high energy” fracture or extensive contamination, definitive fixation is deferred until a second or third debridement can be performed to ensure an appropriately clean wound bed. Often antibiotic-eluting methylmethacrylate beads are placed that contain vancomycin and tobramycin. These act to increase the local antibiotic delivery.48 Often temporizing external fixation is placed until the definitive fixation can proceed. Primary closure of all wounds is attempted. Leaving an otherwise closable wound bed open effectively buys that patient flap coverage. Vacuum-assisted closure (VAC) has a significant utility in closed incisions/lacerations, and it can greatly help the healing of a wound in the immediate postoperative period.49 VAC therapy is also frequently used, in conjunction with or without antibiotic beads, for grade IIIB fractures, or those in which primarily closure is not feasible. For these fractures, the time to flap-coverage is of the utmost importance and should be performed definitively within seven days of the injury.39 Despite recent advancements, open fractures of the lower extremity remain one of the most difficult challenges faced in orthopedic trauma (Fig. 40-3).
FIGURE 40-3
Healed Gritti-stokes amputation in a 14-year-old female after failed tumor reconstruction. The bone cuts on the patella and distal femur are similar to those used in a total knee arthroplasty. The patella is secured to the distal femoral metaphysis, where it then heals. This is an end-bearing amputation. (Photo used with permission of Dr David Chafey.)

The large volume of musculoskeletal tissue in the lower extremities, including the pelvis, increases the potential systemic effects of lower extremity injuries. Bleeding and accumulation of extracellular fluid may cause hypovolemia and contribute to systemic hypotension.50 Several units of blood can be lost into severely injured thighs, and preoperative blood loss associated with a single femur fracture is up to 1500–2000 mL. A crushing wound of the lower extremity releases intravasated debris (eg, bone marrow), myoglobin, related muscle breakdown products, and various inflammatory mediators. The release of these substances may cause fat embolism and adult respiratory distress syndrome (ARDS), acute renal failure, and multiple organ failure (MOF).51,52,53
As demonstrated by Tscherne, Bone et al, Trentz and coworkers, and others, prompt surgical treatment for severe extremity injuries benefits the whole patient.6,54,55 Early fracture stabilization reduces the systemic effects of fractures, including SIRS, sepsis, MOF, and ARDS. Early stabilization reduces pain and the need for analgesic medication, and promotes mobilization of the patient with attendant benefits to the respiratory and gastrointestinal systems. While fracture fixation is particularly beneficial for the patient with injuries to the lower extremity and pelvis, “damage control” procedures should be undertaken if the patient is in shock, coagulopathic, hypothermic, or has an actively developing traumatic brain injury.4,5,56,57,58 The concept of damage control orthopedics (DCO) emphasizes rapid provisional skeletal stabilization with simple external fixators, followed by delayed definitive fixation when the patient is stable and the inflammatory system is less primed, usually at 5–10 days post-injury, thus minimizing the risk of the second hit phenomenon.4,7,8,56,59 There is a large body of evidence illustrating that intramedullary nailing has significant consequences on the immunologic status of a patient.60,61,62 While most surgeons are in agreement regarding the use of DCO for patients in extremis and early total care (ETC) for stable patients, controversy exists concerning the subset of patients who are “borderline,” neither in extremis or stable.
There is a substantial body of evidence on both sides of the argument concerning the treatment of “borderline” patients. DCO has been championed by Pape and others in increasingly improving literature.8,63,64,65 More recently, there has been a considerable body of evidence that expands the indications for ETC, absorbing the majority of the “borderline” patients.9,11,66,67,68 Pape’s original study has been repeated with results that clearly support the use of ETC for the same subset of patients for which he found DCO to be better.69 There is likely a large part of this shift that has resulted from improvements in resuscitative efforts: patients are improving from “borderline” to stable and resuscitated before there has even been an opportunity to initiate DCO. Thus, ETC becomes the more viable option.
One of the most challenging decisions involved in the care of an injured patient is whether or not to attempt salvage of a severely injured limb.70 Although every appropriate effort should be made to preserve functional and anatomic integrity, for some severe lower extremity injuries, an amputation and prosthesis may be more effective for the patient than a limb that is still attached but is of limited use. In the acute phase, the decision to amputate will depend primarily on the immediate condition of the patient and the feasibility of stabilizing/revascularizing the injured limb (see Fig. 40-1). In those cases in which the patient is hemodynamically unstable and revascularization cannot be accomplished without increasing the chance of death, amputation is the only choice. In these cases a guillotine-type amputation is appropriate, but every effort should be made to preserve length and coverage options. In particular, efforts to preserve the potential for a below-knee amputation (BKA) by preserving any viable distal muscle and/or skin will improve the patient’s outcome.71 Free tissue transfers, rotational flaps, and skin grafts can all be used effectively to improve length and provide a durable, useful stump. Many surgeons are unaware that well-padded stumps can be skin-grafted and that free flaps are even feasible. A correctly made BKA prosthesis should load through the thigh and not be end-bearing. Unfortunately, in many cases, the decision to amputate is made in the middle of the night, without opportunity for consultation with experienced salvage surgeons. Multidisciplinary decision making in these severe cases may provide increased reconstructive options whether the limb is salvaged in entirety or amputated.
If the limb is initially salvaged, then further decisions must be made regarding the desirability of maintaining the salvage effort, which usually involves multiple further operations. The doctor-patient relationship becomes very important at this point. The psychosocial factors that play into losing a limb are similar to the patterns of grief associated with losing a loved one.72 Generally speaking, a patient who has sustained a catastrophic lower extremity injury will never have the same level of function as prior to the injury; and how well that patient recovers—whether salvage or amputation is performed—is more determined by economic, personal, and social factors than by injury or surgical factors.73 If a patient is low-functioning at baseline, it is unlikely he will have a good outcome regardless of which operation is performed. The more cost-effective option at that point is therefore amputation. It can take a considerable amount of time spent with the patient to elucidate the characteristics that will define their recovery.
At times, the decision between salvage and amputation is made simpler by certain clinical findings. For example, while a limb can still be salvaged despite necrosis of the plantar skin, free flaps and skin grafts to the sole of the foot are frequently not tolerated and are unlikely to be very functional. Similarly, systemic consequences of the initial injury can supersede decisions regarding the limb. Historically, there were clinical indicators that were used to help guide this decision such as injury scoring systems and the presence or absence of plantar sensation. The Lower Extremity Assessment Project (LEAP) is the best recent effort to characterize these injuries, and it found that injury scores were not predictive of a patient’s likelihood to have success with reconstruction.74,75 Similarly, the presence or absence of plantar sensation upon initial presentation was not predictive of plantar sensation after reconstruction.76 Despite improvements in the body of evidence surrounding this difficult subject, much of the decision making remains out of the hands of the surgeon: it requires a discerning eye to provide the best care for each individual patient faced with this difficult decision.
If the decision is made to amputate, the level of amputation greatly impacts future function. Proximal amputations have greater functional impairment and are often less satisfactory then prosthetic alternatives. Prosthetic replacement of the foot and ankle is highly functional whereas the prosthesis for an above-knee amputation requires more energy for ambulation and is less functional. Thus, every reasonable effort is appropriate to preserve the patient’s own knee joint and enough proximal tibia (at least 10 cm below the joint) to provide for good prosthetic fitting. If this cannot be done, a Gritti-Stokes amputation can be considered. This is a through knee amputation where the patella is attached to the cut end of the femur (see Fig. 40-3). Different from a BKA, this is an end-bearing amputation that can be used with a prosthesis that is not too different from that for a below knee. Prostheses for very proximal femoral amputation levels, hip disarticulation, or hemipelvectomies are rarely functional for ambulation; therefore, efforts are also appropriate to preserve an adequate above-knee amputation level.
Technically, replantation is possible for complete and subtotal lower extremity amputations. However, given the current near impossibility of lower extremity nerve regeneration in adults, the functional outcome is questionable. In general, only cleanly separated traumatic amputations in young individuals without significant systemic risk factors, including smoking, deserve consideration for replantation. Revascularization in the face of severe neuromuscular injury may result in a viable but painful, dysfunctional limb. Consultation with an experienced replantation team is essential. Preservation of the amputated part is according to the same principles for upper extremity replantation. Care must be taken to not jeopardize the patients’ life in lower extremity replantation and revascularization. Reestablishment of blood flow after a period of prolonged hypoxia can have a toxic effect, causing systemic inflammation and MOF. Consideration should be given to rapid external fixator and arterial shunt placement to “buy time” and permit an overall reassessment of the patient and of the desirability of reattachment of the limb.
Successful open reduction and internal fixation (ORIF) of displaced acetabular fractures significantly improves the prognosis of these potentially devastating injuries and permits early mobilization of a patient who might previously have been managed with many weeks of skeletal traction and bed rest.
Fractures of the acetabulum are articular injuries of the pelvic portion of the hip joint with profound implications for the long-term function of the hip joint. One of the first historical citations of these injuries was in Homer’s Iliad: “Just as Diomedes hefted a boulder in his hands, a tremendous feat…flung it and struck Aeneas’s thigh where hipbone turns inside the pelvis, the joint they call the cup—it smashed the socket.”77 Judet and Letournel’s seminal work has led to our current classification, understanding, and management of acetabular fractures.78 Today, there is a bimodal distribution in the patients presenting with these fractures: high energy in young patients, and low energy fall in the elderly. The fracture pattern is a result of the position of the femoral head when it impacts the acetabulum. There is a high association of other injuries whether they are orthopedic or systemic.79 The AP pelvis view obtained in the original ATLS survey has six radiographic landmarks used to quickly identify an acetabulum fracture. Prompt orthopedic consultation is warranted with all acetabular fractures. Fractures associated with subluxation of the femoral head or incarcerated fragments may require skeletal traction until the patient is medically cleared for surgery. Oblique x-rays and CT scans are used to classify the acetabular fracture, to assess displacement and need for surgical treatment, and to determine the best surgical approach. There are multiple surgical approaches to the acetabulum including the Kocher-Langenbeck, the ilioinguinal, the extended iliofemoral, the modified iliofemoral, the Stoppa, the triradiate, combined anterior/posterior approaches, and percutaneous.80 The surgical approach is dictated by the fracture pattern and the overall condition of the patient. Certain approaches that may be otherwise ideal may have to be abandoned secondary to prior interventions, including a very distal laparotomy incision or embolization to major pelvic vasculature, such as the superior gluteal artery. A complete three-dimensional understanding of the fracture is essential for formulating a preoperative surgical plan. Preservation of soft tissue attachments is needed to promote healing and avoid osteonecrosis of the fracture fragments. Vital neurovascular structures must be protected. A precise anatomic reduction must be achieved and fixed stably, generally with screws and plates, which must not encroach upon the articular surface. Intraoperative fluoroscopy has become a valuable tool for ensuring appropriate placement of orthopedic hardware around the acetabulum. Minimally invasive percutaneous screw fixation represents a challenging but valid alternative to open reduction with internal fixation in minimally displaced fractures or in patients with significant risk for wound complications or a “second hit” insult related to extensive surgical procedures. Complications and poor results become less frequent with increasing experience of the acetabular surgeon. Acetabular fracture surgery remains among the most challenging procedures in orthopedics. These difficult and dangerous reconstructive surgeries should generally be done in specialized centers to ensure that each patient receives optimal treatment.81
Acetabular fractures in osteoporotic individuals pose special problems. There is frequently significant comminution, often with a protrusio deformity (Fig. 40-4), and bone quality so poor that conventional fixation techniques are doomed to failure. In these instances, total hip arthroplasty with specialized acetabular reconstruction devices allows improved fixation and early weight bearing of the elderly patient. Because the femoral head is removed in total hip arthroplasty, extensile or combined exposures may not be required, and operative morbidity may be reduced.82
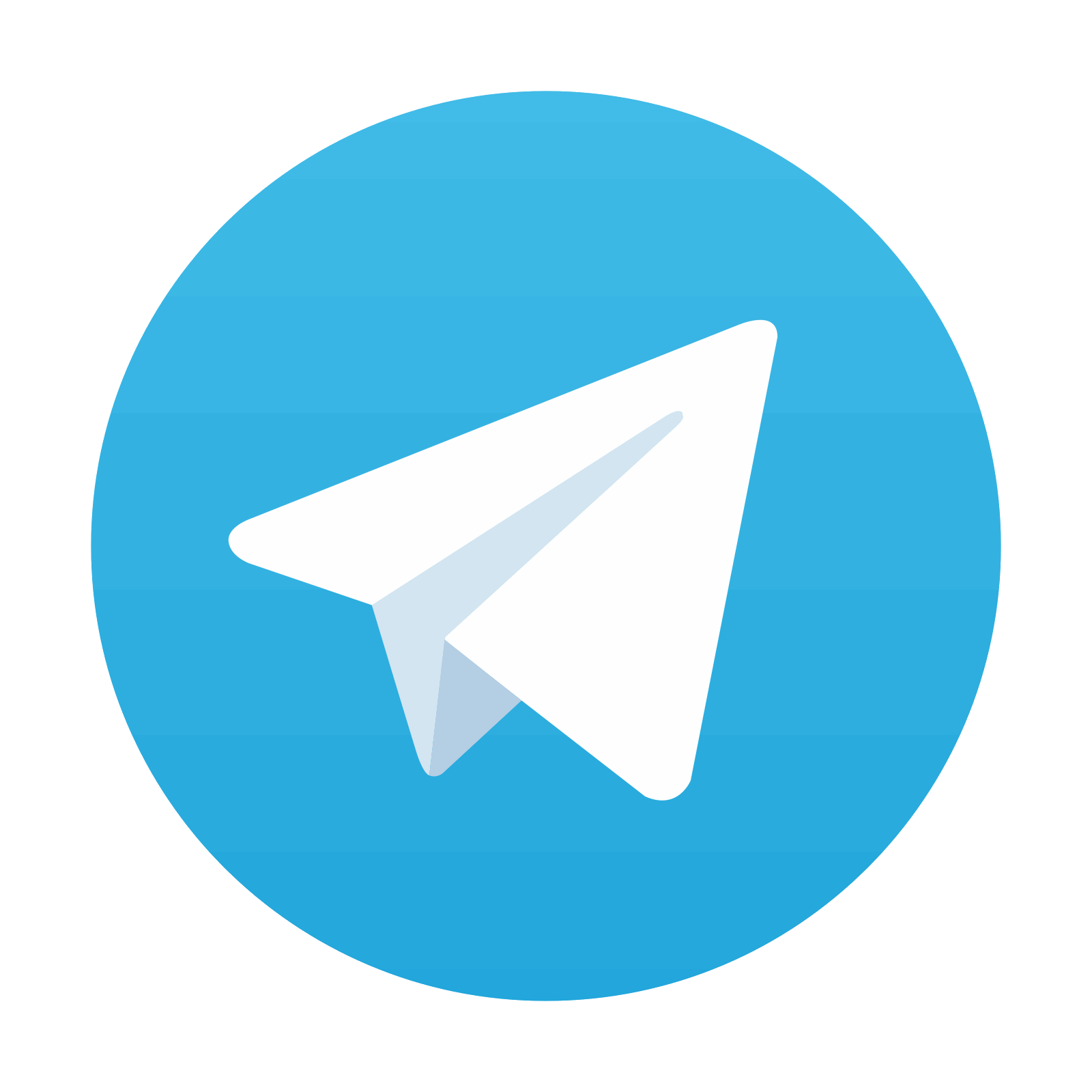
Stay updated, free articles. Join our Telegram channel

Full access? Get Clinical Tree
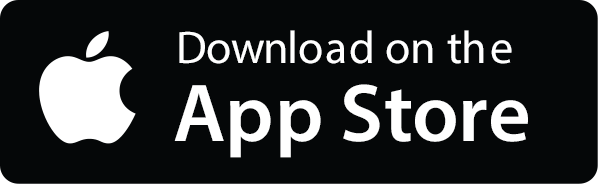
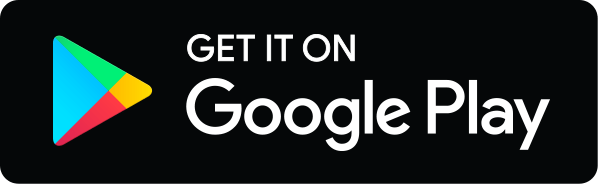