Autologous
Allogeneic
Donor disease issues may require alternatives
Prospective donor selection can reduce genetic risk
Expensive to manufacture
Only iPSC-based source
Much cheaper but cost of immune suppression
Much longer to make and so unavailable for acute damage
Alternative tissue sources possible
Regulations may make it impossible
Off the shelf product that fits current business models
Regulatory changes may make it much easier
Available for acute therapy as intermediate product can be stored
Immune matched so immune suppression not required
Regulatory pathway clear
iPSC haplobank strategy may reduce cost
The blood brain barrier may reduce the immune issue even if not matched
Future cost reductions possible with technical breakthroughs
Haplobank strategy can further mitigate the immune issue
Acceptance and perceived value higher
Novel engineering strategies may solve the immune issue
To develop therapies related to treatment with hepatocytes, three models of iPSC-based therapy have evolved (summarized in Fig. 11.1). The first is the autologous model (one to one model) where cells from the affected individual are isolated and if necessary the gene defect is corrected and the corrected IPSC cell clone is then differentiated into an appropriate phenotype and this differentiated cell product is used for therapy. This autologous model is akin to autologous therapy utilized with sibling-matched bone marrow transplant or autologous cartilage repair or adipose stem cell-based therapy. The model here is that hundreds or millions of iPSC lines would be made but would be made on a case-by-case basis. Once made, the cells could be stored indefinitely so that if there was a need by the same individual again, then the line could be differentiated into a second type of required cell or even a third if required. While the initial therapy would take time and would be time consuming, subsequent need could be satisfied relatively quickly. Wealthy individuals could have their own iPSC lines made as an insurance akin to what private cord blood and tissue banks do now with the added advantage that iPSC unlike cord blood represents an infinite supply and can make many more derivatives than cord blood. The market has shown that such a model can work though there are some important differences between storing cord blood and making and storing iPSC. Perhaps the first critical difference is the regulations regarding such therapy in the United States. Unlike autologous cord blood transplants, autologous iPSC (even if they are not gene corrected) will be treated as a new biologic and will require an IND process under current rules.
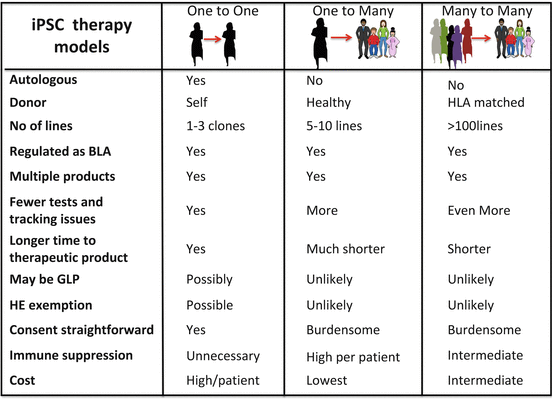
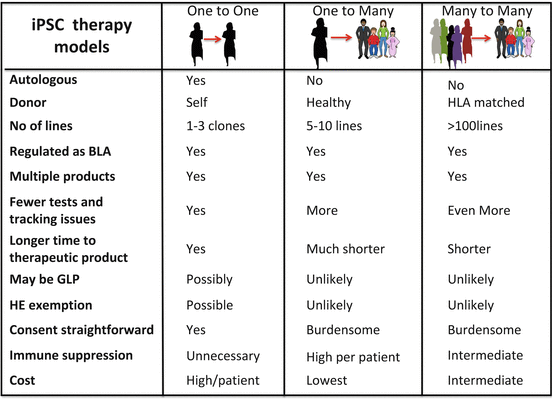
Fig. 11.1
Three models of IPSC-based banking are described and their relative advantages and disadvantages summarized
A second model (many to many model) for IPSC-based therapy is to consider HLA matching and make an immune compatible cell. The model here is to identify donors prospectively whose HLA profile is such that they can donate to many individuals. Thus, a much more limited but selected set of iPSC lines will be enough to provide a 6/6 match of the HLA locus and then would require limited immune suppression. Evidence that only limited immune suppression will be required and the drugs that are suitable has come from studies done in bone marrow and organ transplants. The regimes, while expensive and with side effects, are certainly well tolerated by a large number of individuals. Calculations to determine the number of lines required have been provided by various groups [19], and these suggest that as few as a hundred lines may be sufficient for some homogeneous populations. In any case even in the worst case scenario, the number of lines required is manageable and indeed a haplobank approach has been proposed by Dr. Wilmut and colleagues [19]. The model here is akin to the National Bone Marrow registries where a database of HLA types available worldwide is maintained. A consensus on the collection and derivation of the product by the various national groups is agreed to so that a sample from any bone marrow registry can be sent to any recipient worldwide. A potential recipient or his/her physician searches the repository and identifies a potential match. A criteria is developed for prioritization of recipients and for shipping the sample worldwide.
While the model for iPSC is conceptually similar, there are two important distinctions. One crucial difference is that IPSC themselves are not the final product, but rather they are the starting material to make multiple final products and in the case of a haplobank for multiple individuals. In addition to developing a shipping and storage capacity like the bone marrow banks, one will have to develop a manufacturing capacity to make multiple products. Manufacturing a clinical product adds a separate and additional layer of regulations to those under which registries and banks are governed. It is important to note that there is some but not adequate harmonization of manufacturing regulations that one can reasonably assume that a product manufactured in one country can be utilized worldwide. The second distinction is that there are currently no consensus agreements on what constitutes a good iPSC line and what adequate QC and potency assays are. This is crucial for a haplobank strategy. If the regulators in one country are not comfortable with the approval process in another, then applying the bone marrow model may be impossible. While the regulators may be willing to come to consensus, it is not clear investigators themselves have agreed to either a list of tests or the method of testing or what is an agreed to acceptance and rejection criteria.
A third approach is simply the allogeneic (one to many model) approach with the assumption that cell transplants can work without immune suppression or at most limited suppression in certain regions of the body. Postmortem analysis performed in a limited number of patients showed that the graft survived and integrated. Morizane and colleagues [11] did match and mismatched transplants in primates and likewise showed that while completely matched transplants did better, the mismatched transplants also survived for periods as long as a year (maximum time period examined). There are several reports of organ transplant recipients stopping their immune suppression regimes with organ failure or rejection. Indeed many of the fetal transplants for Parkinson’s disease were not immune matched and either limited or no immune suppression was used. There is also hope that as technology advances, other methods of immune regulation will obviate the need for HLA-based matching efforts. In this case, the model would be that a group would identify two or three lines that would be carefully selected from individuals who were healthy, who had no hereditary disease, and who did not carry alleles for increased susceptibility to any common disease and the iPSC clone would be selected for its ability to grow and differentiate into multiple phenotypes [2]. There are several reasons companies find this model very attractive. One, it fits their current model for manufacturing biologics and for getting approvals through the IND process. Two, it is much cheaper to qualify one line for therapy and then test multiple lines in preclinical studies. Three, since a single iPSC can be used to generate multiple products, one can design manufacturing processes to achieve even greater economies than are possible with adult stem cell populations making iPSC-based cell therapy economically viable. iPSC would still be preferred over ESCs as one could identify healthy individuals and select the best donor which one could not do with ESC lines which are genetically distinct from either parent. Getting approvals/consent is also much easier as case law is quite clear on tissue donation from adults as is ownership of material. Unlike obtaining consent for tissue donation for iPSC generation, neither the approvals nor the consent process is as clear-cut with blastocyst donation for the generation and use of ESCs. Likewise, the patent rules for ESCs ownership are complex and in flux, while those for iPSCs somewhat more clear-cut.
In summary, each of these models appear to be viable, and ones choice depends to some extent on the scientific assumptions one makes and the corresponding manufacturing constraints. If costs can be contained, then true autologous therapy would be a first choice. This could be performed relatively easily, the size of production is small, and the parental line can be stored for future use. There is no ongoing cost or risk of ongoing immune suppression, and the history of autologous transplants being better is generally well accepted. Even in chronic degenerative diseases where the cell product will carry the same genetic susceptibility, one can reasonably argue that the disease process will take long, and as such autologous therapy will work long enough to confer benefit. The advances in zero-footprint gene engineering which allow for integration-free reprogramming using episomal plasmids, Sendai virus, or mRNA enable the generation of high-quality iPSCs free from viral integration. The standardization of these methods and reduction in cost allow one to consider autologous cell-based therapy as well. This coupled with advances in gene editing techniques suggests that iPSCs from patients with genetic disorders could be isolated, gene edited, or engineered to provide the missing gene product which permits the treatment of individual patients. These advances, coupled with advances in differentiation, allow us to consider generating “cured cells” for personalized therapy.
The other models previously discussed offer significant cost savings and, in many cases, a time to therapy advantage that is simply not possible for autologous therapy. Thus, there are several acute indications where cells may be required only transiently in which case allogeneic therapy may be the preferred option. Allogeneic therapy may also be the preferred option when cells are required only transiently. Once the cells provide their trophic or immune modulatory or other short-term functions, one can rely on the body’s natural immune response to eliminate the mismatched cells in due course. This lessens the risks of long-term integration, potential tumorigenicity, and monitoring. One may also imagine indications where one wants to stimulate the bodies’ natural immune response, and in this case, allogeneic cells may be the preferred cell type. It is important not to discount the cost savings as well.
The haplobank combines cost savings with a faster time to delivery with a reduction in the cost of immune suppression, thus allowing cells to be used for both long-term integration and transient use. While not optimized for either mode of therapy, it provides a significant advantage for approval through the regulatory process though unlike an allogeneic model, it is more complex to develop and requires harmonization and standardization between regulations in different countries to be viable.
To us it appears likely that all three models will coexist and users will have a choice of the optimum cell type they wish to use for a particular indication and regulatory authorities will have to evaluate their regulatory regimes to ensure that choices are available.
11.2 Regulatory Issues with PSC-Based Therapy
Irrespective of which model ultimately prevails or all three models exist side by side, these models share several issues in common. In all cases, donors have to be identified and iPSs generated, iPSCs need to be stored until used, and differentiated products must be derived from them. The end product needs a defined release criteria to ensure quality and consistency. This product then needs to be tested in preclinical studies to demonstrate safety of the manufactured product followed by early clinical studies to demonstrate safety and efficacy of the product. A proposed common framework of how this may be done is outlined in Fig. 11.2, and the US FDA has proactively begun developing regulations for this new class of therapy. What is clear is that allogeneic, autologous, and cells from a haplobank will be regulated under the same core set of regulations that govern any other manufactured biologic and these issues that are common with other biologics are discussed elsewhere [15]. In the section below, we discuss the efforts made by different regulatory authorities to accelerate the translational efforts of various groups using existing regulations or modifying them to ensure that safety is not compromised while accelerating the translational efforts and reducing the risk burden on providers of this type of therapy.