CHAPTER 16 Intestinal Gas
COMPOSITION AND VOLUME OF GASTROINTESTINAL GAS
Five gases—N2, O2, CO2, H2, and methane (CH4)—account for more than 99% of intestinal gas; many additional gases are present in trace concentrations. In a study of 10 healthy fasting subjects, the composition of gas within the gastrointestinal tract was assessed using a washout technique in which a rapid infusion of argon into the jejunum was used to flush out the intestinal gases.1 N2 was usually predominant, O2 was present in low concentrations, and the concentrations of CO2, H2, and CH4 were highly variable. The volume of each gas within the intestinal lumen reflects the balance between the input and output of that gas. Input may result from swallowing, chemical reactions, bacterial fermentation, and diffusion from the blood, whereas output involves belching, bacterial consumption, absorption into the blood, and anal evacuation. Measurements of intestinal gas volume, originally obtained using a body plethysmograph and later using a washout technique, have indicated that the volume of intestinal gas is approximately 200 mL in healthy subjects. Similar data have been reported using a specifically designed and validated computed tomography (CT) technique.2 In the fasting state, the healthy gastrointestinal tract contains only about 100 mL of gas distributed almost equally among six compartments—stomach, small intestine, ascending colon, transverse colon, descending colon, and distal (pelvic) colon. Postprandially, the volume of gas increases by 65%, primarily in the pelvic colon (Figs. 16-1 and 16-2).
GAS METABOLISM AND EXCRETION
The diffusivity of a gas across the mucosa of the gastrointestinal tract depends on its solubility in water; for a given partial pressure difference, CO2 diffuses much more rapidly than H2, CH4, N2, and O2. The rate and direction of diffusion of each gas is a function of the diffusivity, partial pressure difference between lumen and blood, and exposure of the gas to the mucosal surface.3 H2 and CH4 absorbed from the bowel are excreted in expired air, and breath analysis provides a simple means of assessing the volume of these gases in the gastrointestinal tract. Figure 16-3 schematically depicts the processes regulating the volume and composition of gas in various segments of the gastrointestinal tract.
MOUTH TO STOMACH
The absence of the gastric bubble in subjects with advanced achalasia indicates that air swallowing (rather than intraluminal production) is the major source of stomach gas. Ultrafast CT studies have shown that an average of 17.7 mL of air (14 mL of N2) is swallowed with a 10-mL bolus of liquid.4 Most of this swallowed air presumably is regurgitated, because neither absorption of intestinal gas nor excretion in flatus can account for the relatively enormous quantities of N2 that would be swallowed with the daily ingestion of 1500 mL of liquid.5 Swallowed air contains minimal CO2, and CO2 diffuses from blood into the stomach bubble. The Po2 of swallowed air is higher than that of blood, and O2 is absorbed from the stomach.3
SMALL INTESTINE
In the upper small intestine, CO2 is liberated from the interaction of bicarbonate and acid. Bicarbonate is delivered to the intestinal lumen via biliary, pancreatic, and small intestinal secretions, and the acid is delivered via gastric secretion (about 30 mEq/hr after meals6) or fatty acids released during digestion of triglycerides (about 100 mEq of acid/30 g of fat). The Pco2 of duodenal contents after a meal rises to 300 mm Hg in control subjects and 500 mm Hg in patients with duodenal ulcers,7 which indicates that CO2 comprises about 40% and 66% of duodenal gas in these two states, respectively. This high Pco2 causes the luminal Pn2 to fall below that of blood, and N2 diffuses from blood into the lumen.
Although CO2 is absorbed rapidly during its passage through the small intestine, a study using CT-based volumetric analysis has shown that in the postprandial period, small bowel gas volume remains constant, whereas gas increases in the pelvic colon (see Fig. 16-2). This colonic increment occurs 99 ± 22 minutes after the meal, earlier than would be expected for gas derived from colonic fermentation of food substrates. Thus, gas (N2 or CO2) of proximal origin presumably is propelled caudally to increase the volume of gas in the pelvic colon.8
COLON
In the colon, luminal bacteria produce and consume intestinal gases, and this activity frequently is the primary determinant of anal gas output. The composition of the colonic microflora, which varies considerably among individuals, is determined by early environmental conditions as well as factors encountered later in life, such as antibiotic and dietary exposures.9 For example, chronic ingestion of high doses of an intestinally malabsorbed disaccharide (lactulose by patients with constipation or lactose by persons with intestinal lactase deficiency) results in diminished breath H2 excretion following a challenge dose of the same disaccharide.10 This phenomenon appears to result from the colonic proliferation of organisms such as Bifidobacterium spp. that ferment lactose or lactulose via non-H2 releasing pathways.11 The production of the bacterial gases CO2, H2, and CH4 may indirectly increase luminal gas volumes by reducing luminal Pn2 to a value less than that of blood, thereby causing N2 to diffuse from the blood to the lumen. Similarly, the low Po2 of colonic gas results in diffusion of O2 from the blood to the lumen; however, O2 is rapidly consumed by intestinal organisms (see Fig. 16-3).
Fermentation of Unabsorbed Substrates
The colon harbors large number of hydrogen-producing bacteria that ferment undigested substrates (carbohydrates and protein), with the release of hydrogen and carbon dioxide.12–14 Newborn infants, as well as germ-free rats, excrete no H2, whereas H2 is detected within hours of bacterial contamination of the gastrointestinal tract. Therefore, bacterial metabolism is the sole source of intestinal H2.15
Various carbohydrates are incompletely absorbed by the intestines of healthy subjects (Table 16-1). Most of the world’s adult population malabsorbs lactose as a result of a genetically programmed reduction in lactase synthesis. Fruits and vegetables (particularly legumes) contain indigestible oligosaccharides such as stachyose and raffinose that are readily fermented by colonic bacteria.16 A fraction of the complex carbohydrate in wheat (pasta, white bread), oats, potatoes, and corn is not absorbed in the small bowel.17 In part, this malabsorption reflects the presence of starch in a physical form that resists amylase digestion. Resistance to amylase is further enhanced when starches are refrigerated and then reheated, a process that results in crystallization (retrogradation) of the starch.18 White rice flour is the only complex carbohydrate that is almost totally absorbed. A sizable fraction of the healthy population cannot completely absorb the large quantity of fructose present in soft drinks. The poor absorption of sorbitol has led to its use as a low-calorie sugar substitute; however, this compound is readily fermented by colonic bacteria. Although fermentable fiber is commonly assumed to provide substrate for gas production, the standard dose of a psyllium results in a minimal increase in H2 excretion.19 Some components of normal meals interfere with absorption of nutrients and thereby increase colonic gas production; for example, fiber increases starch malabsorption,20 and a pancreatic amylase inhibitor in beans slows starch digestion and absorption.21 The high fasting H2 excretion observed in small intestinal bacterial overgrowth or untreated celiac disease has been attributed to fermentation of large quantities of mucus secreted by the intestine in these conditions.22
Table 16-1 Carbohydrate-Containing Foods That May Be Malabsorbed in the Healthy Human Small Intestine
FOOD | MALABSORBED CARBOHYDRATE |
---|---|
Complex carbohydrates (wheat, corn, potatoes) | Resistant and retrograded starch (see text) |
Dairy products (milk, ice cream, cottage cheese, yogurt) | Lactose |
Dietetic candies and chewing gum | Mannitol, sorbitol, xylitol |
Grains, fruits, vegetables | Fiber (hemicellulose, pectin, gums, mucilage) |
Legumes (baked beans, soy beans) | Stachyose, raffinose |
Soft drinks, honey | Fructose |
Gas-Consuming Flora
Some colonic bacteria consume intraluminal gases (H2, CO2, and O2), and this catabolism may account for a considerable proportion of intraluminal gas disposal.14,23,24 The vast majority of H2 released in the intestine normally is consumed by other bacteria, and the marked interindividual differences in breath H2 excretion observed after ingestion of a given dose of lactulose probably reflects differences in bacterial H2 consumption rather than differences in production. Sulfate-reducing bacteria use H2 to reduce sulfate to sulfide. Methanogenic bacteria use H2 to reduce CO2 to CH4.25–27 This reaction uses 5 moles of gas to produce 1 mole of CH4, and thus results in a reduction in bowel gas. Although methanogens are present in the feces of almost all adults, only about 40% of adults have sufficient concentrations of methanogens (106/g) to yield detectable breath CH4 concentrations.28 Sulfate-reducing bacteria and methanogens compete for H2, and feces of subjects usually contain one or the other type of organism. Analysis of flatus, however, has shown that subjects who excrete methane also can excrete appreciable H2S, suggesting that sulfate-reducing bacteria remain active in the proximal colons of these subjects.
The fraction of H2 that escapes consumption is determined by the efficiency of fecal stirring and the quantity and type of H2-consuming bacteria. Because a higher hydrogen tension is maintained in poorly stirred feces, a greater fraction of total H2 production is consumed in the semisolid feces of the left colon than in the liquid feces of the right colon. Consumption is markedly enhanced by the presence of methanogens, which oxidize H2 more rapidly than other H2-consuming bacteria. Studies of strongly methanogenic feces have suggested that if similar high concentrations of methanogens were present throughout the colon, almost no H2 would escape consumption. CH4-producing bacteria, however, are normally present in a high concentration only in the left colon.29 As a result, H2 liberated in the right colon is not acted on by methanogens until it reaches the left colon, thus explaining the reduced but still appreciable breath H2 excretion observed in most CH4-producing subjects.30 The inability of some subjects to increase breath H2 excretion after intestinal carbohydrate malabsorption probably reflects extremely efficient consumption of H2 by methanogens rather than a failure to produce H2.
Odoriferous Gases
None of the quantitatively important gases has an odor, and the unpleasant odor of feces results from gases present in trace quantities. The intensity of the noxious odor of flatus samples positively correlates with the concentrations of hydrogen sulfide and methanethiol.31 Hydrogen sulfide is released during bacterial metabolism of sulfate, cysteine, and mucin; therefore, both exogenous and endogenous compounds supply the substrate for this reaction. Methionine appears to be the favored substrate for methanethiol production. In addition to their noxious odor, hydrogen sulfide and methanethiol have toxicities similar to that of cyanide. The colonic mucosa protects itself from the damaging effect of these compounds via a highly developed system that metabolizes these gases to thiosulfate.32 This detoxification mechanism is so efficient that negligible quantities of these gases enter the blood perfusing the colon, and hydrogen sulfide and methanethiol of intestinal origin are not excreted in the breath.33 By contrast, an odoriferous sulfur-containing gas (allyl methyl sulfide) derived from garlic is not metabolized by the intestinal mucosa and is absorbed from the gut and excreted in expired air.33
ELIMINATION
The intestinal gas that is not absorbed or metabolized is eliminated via anal evacuation. Despite the many beliefs concerning which foods cause gas, scientific data concerning diet-related increases in rectal gas evacuation are sparse. Collection of gas using a rectal tube has shown a postprandial excretion rate of 15 mL/hr with a low-fiber diet, 93 mL/hr with a normal diet, 140 mL/hr after ingestion of Brussels sprouts, and 176 mL/hr with a pork and beans diet.16 Another study in which 24-hour gas evacuation was measured has shown that with a normal diet containing 200 g of beans, 705 mL (range, 476 to 1491 mL) was excreted per anus, and 50% of this gas was hydrogen, whereas with a fiber-free diet, the evacuation rate fell to 214 mL, with a low hydrogen content.34
The average frequency of passages of gas per rectum by healthy subjects is roughly 10 times daily, with an upper limit of normal of about 20 times daily.35 Neither age nor gender significantly correlates with flatus frequency.16 The composition of flatus is highly variable, depending on fermentative activity in the colon. In the presence of fermentable residues in the colon, the volume of flatus increases as a result of more rapid production of H2, CO2, and methane (in subjects with methanogenic flora), whereas N2 and O2 concentrations decline. Because absorbed H2 and CH4 are not metabolized, breath excretion of these gases equals their rates of absorption. Breath H2 excretion is the product of the alveolar ventilation rate and alveolar H2 concentration. Because alveolar ventilation is relatively constant in sedentary persons, the end-alveolar breath H2 concentration can be used as a simple indicator of total breath H2 excretion. Long-term simultaneous measurements of rectal and breath H2 excretion have been made in adult subjects maintained in an air-tight environment.36 Normally, breath H2 excretion averages about 50% of total (breath plus rectal) excretion; however, this percentage increases to 65% when production is low and decreases to 20% when production is high. Because the intestinal absorption process for H2 is not saturatable, the decreasing proportion of H2 excreted in the breath with rapid production presumably is a result of more rapid propulsion of this gas to the anus.
INTESTINAL PROPULSION, ACCOMMODATION, AND TOLERANCE OF GAS
The rate of propulsion of gas in the intestine toward the anus is a crucial determinant of the volume of gas present in each segment of the gastrointestinal tract and the total volume in all segments at any moment. Gas transit determines the residence time of gas in the intestinal lumen; hence, the absorption and bacterial consumption of gas are influenced by transit time, as is the composition of gas evacuated from the rectum.37 Intestinal gas transit and tolerance have been measured using a gas challenge test, in which a mixture of gases is constantly infused into the jejunum and anal gas output is quantified (Fig. 16-4). An initial dose-response study using infusion rates of up to 30 mL/min (1.8 L/hr) has shown that most healthy subjects evacuate gas as rapidly as it is infused, with little or no discomfort.38 The intestine actively propels gas, with transit of gas more effectively in the erect than in the supine posture.39 Transit of gas, like that of solids and liquids, is modulated by a series of reflex mechanisms; intraluminal nutrients, particularly lipids, delay gas transit,40 whereas mechanical stimulation of the intestine (e.g., mild rectal distention) has a strong prokinetic effect.41
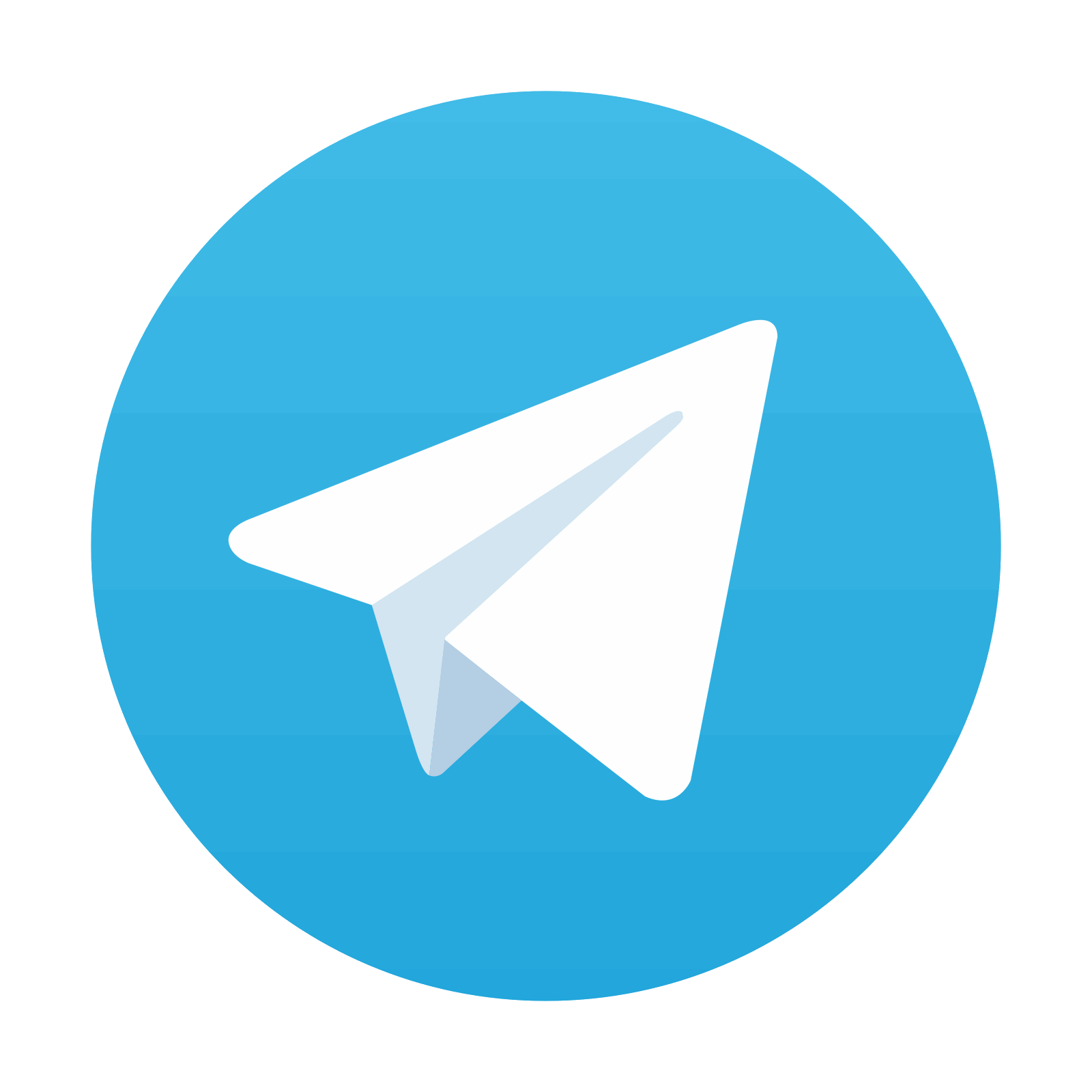
Stay updated, free articles. Join our Telegram channel

Full access? Get Clinical Tree
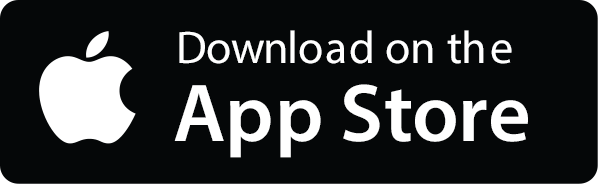
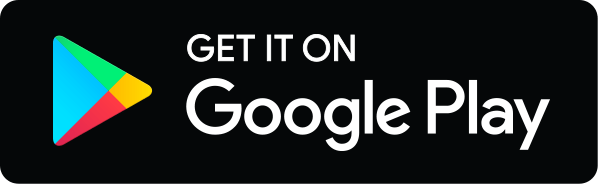