3.1
Generalities
The instruments used for the ureteroscopic approach of upper urinary tract conditions are in a continuous evolution. The first ureteroscopes were too large in diameter, their use being restricted to a small number of patients. Moreover, the methods for stone fragmentation or extraction, as well as for tissue resection/fulguration, were limited. Subsequently, the instruments evolved and the technique improved, the ureteroscopes allowing the use of an increasing number of accessory instruments, in the conditions of a decreasing diameter.
Recently, both rigid and flexible ureteroscopes have undergone many improvements in order to increase their efficiency, durability, and to gain the ability of using specific working instruments. Most of the ureteroscopes that are currently in use have dimensions that allow them to be ascended without requiring dilation of the ureteral orifice. A large number of accessory instruments may be used for rigid ureteroscopes, as well as for flexible ones.
The development of rigid and flexible ureteroscopes with increasingly smaller dimensions determined the diversification of transurethral upper urinary tract endoscopy.
The quality of the optical system is extremely important for the endoscopes’ efficiency ( ). There are two types of optical systems:
- •
rigid optical systems
- •
flexible optical systems
The rigid optical systems include a lens that is placed at the distal end of the telescope, a lens system that flips the image, and a viewfinder fitted with a lens. The fixation system of the cylindrical lenses (Hopkins), in the detriment of the serial position, has made it possible to lower the number of optical interfaces, which has led to a decrease of diffraction, to an increase of light transmission, and to an increase of resolution and color fidelity.
The fiberglass light conductor is built parallel with the lenses fitted in the telescope. The viewing angle of the telescope is determined by the ratio between its central axis and the angle obtained at production (0–120°).
In the case of flexible instruments, the transmission of light is achieved through total internal reflection within the optic fascicles. The materials used to make the flexible optical conductors must have different refraction indexes between the central and the peripheral part. The optical fibers are fabricated in parallel bands and lead the image from the distal lens’ focal point to the proximal end of the telescope, the viewfinder. The viewing angle is calculated by the ratio between the instrument’s 0° axis and the viewfinder’s degree of flexion related to this axis.
The flexible instruments have a lower reliability due to the high risk of breaking the optical fibers, the reduction in the quality of the images being directly proportional to the amount of broken optical fibers. This fact leads to the requirement of frequent instrument inspection for the early detection of the decrease in illumination and visualization capability.
3.2
Rigid ureteroscopes
Rigid ureteroscopy can be performed retrogradely, by inserting the endoscope through the ureteral orifice after a prior dilatation of the intramural segment of the ureter or antegradely, by inserting the endoscope through the percutaneous nephrostomy tract and subsequently, through the pyeloureteral junction, into the ureter.
The ureteroscopes, optical instruments intended for the diagnosis and treatment of upper urinary tract disorders, have a caliber that fits in the internal circumference of the dilated ureter. According to Matoushek’s description, the ureteroscope is similar in design “to an elongated pediatric endoscope.”
Rigid ureteroscopes ( Fig. 3.1 ) are ideal for interventions at the distal ureter, being easy to maneuver and providing very good control of the working instruments. The technical progress and improvement of design have also allowed for their use in the proximal ureter and renal pelvis. Classically, the rigid ureteroscopes used cylindrical lens systems. The modern endoscopes involve the use of optic fibers that allow a significant reduction of dimensions, with an increase of durability and with the prevention of image distortion. However, even now, the images obtained by using optic fibers do not equal the quality of those obtained through cylindrical lens systems.

3.2.1
External Configuration
Regarding their dimensions, the classic rigid ureteroscopes had calibers between 13 F and 16 F. The larger caliber endoscopes have the advantage of a wider working channel and, implicitly, superior irrigation and visibility.
However, due to the diameter of over 10 F, the use of these instruments requires the dilatation of the ureteral orifice ( ), increasing the aggressiveness to the tissues.
The incidence of secondary ureteral stenoses after ureteroscopic maneuvers is in direct correlation with the dimensions of the endoscope.
described a rigid ureteroscope with a cylindrical lens system, with an external diameter of 8.5 F and a working channel of 3.5 F.
Regarding the distal end’s design, the first ureteroscopes copied the design of cystoscopes, determining difficulties in approaching the intramural ureter. Resolving this issue subsequently led to the development of ureteroscopes with a conical end ( Fig. 3.2 ).

The uretero-resectoscopes ( Fig. 3.3 ) have in addition a mobile working element, similar to that of resectoscopes used for low endourological interventions.

3.2.2
Optical Systems
The use of rigid optical systems determines distortions in the transmission of light when bent. This phenomenon determines the reduction of the visual field by up to 50% ( ). Applying a torsion force during the approach of the distal ureter may lead, in time, to damaging the optical system.
Rigid ureteroscopes with interchangeable telescope sets have been produced. This allows for an increase of the visual field, and 0–70° optical systems can be used in the same sheath. Due to the large caliber of the sheaths (13–16 F), the subsequent models included integrated telescopes with a visual angle varying between 0° and 6.5°, allowing the ureteroscope’s diameter to be reduced while maintaining a sufficient working channel (3.5–5 F). Obtaining a visual angle of 6.5° allows for an easier orientation of the instruments when emerging from the working channel.
3.2.1
External Configuration
Regarding their dimensions, the classic rigid ureteroscopes had calibers between 13 F and 16 F. The larger caliber endoscopes have the advantage of a wider working channel and, implicitly, superior irrigation and visibility.
However, due to the diameter of over 10 F, the use of these instruments requires the dilatation of the ureteral orifice ( ), increasing the aggressiveness to the tissues.
The incidence of secondary ureteral stenoses after ureteroscopic maneuvers is in direct correlation with the dimensions of the endoscope.
described a rigid ureteroscope with a cylindrical lens system, with an external diameter of 8.5 F and a working channel of 3.5 F.
Regarding the distal end’s design, the first ureteroscopes copied the design of cystoscopes, determining difficulties in approaching the intramural ureter. Resolving this issue subsequently led to the development of ureteroscopes with a conical end ( Fig. 3.2 ).

The uretero-resectoscopes ( Fig. 3.3 ) have in addition a mobile working element, similar to that of resectoscopes used for low endourological interventions.

3.2.2
Optical Systems
The use of rigid optical systems determines distortions in the transmission of light when bent. This phenomenon determines the reduction of the visual field by up to 50% ( ). Applying a torsion force during the approach of the distal ureter may lead, in time, to damaging the optical system.
Rigid ureteroscopes with interchangeable telescope sets have been produced. This allows for an increase of the visual field, and 0–70° optical systems can be used in the same sheath. Due to the large caliber of the sheaths (13–16 F), the subsequent models included integrated telescopes with a visual angle varying between 0° and 6.5°, allowing the ureteroscope’s diameter to be reduced while maintaining a sufficient working channel (3.5–5 F). Obtaining a visual angle of 6.5° allows for an easier orientation of the instruments when emerging from the working channel.
3.3
Semirigid ureteroscopes
With the technological evolution, the rigid ureteroscopes have been largely replaced by semirigid ureteroscopes ( Fig. 3.4 ). These include a high-density optic fiber fascicle, enclosed in a semirigid metallic sheath. The use of these fibers has determined the reduction of the space necessary for the optical component of the ureteroscope, allowing the incorporation of a large working channel, despite a low external diameter.

The optic fiber fascicle allows a vertical flexibility of the endoscope, without creating image distortions.
3.3.1
External Configuration
Usually, the distal end of semirigid ureteroscopes has a diameter between 6 F and 10 F. These dimensions allow performing ureteroscopic maneuvers without dilation of the intramural ureter. The sheath of most semirigid ureteroscopes has a progressively increasing diameter from the distal to the proximal end, where it reaches a caliber varying between 7.8 F and 14.5 F. This design facilitates the progressive dilatation of the ureter as the ureteroscope is advanced. The working length of the models used in practice is of over 31 cm. This length allows the approach of the distal and middle ureter, and in women it is sufficient to also approach the renal pelvis. For ureteroscopic interventions on the pyelocaliceal system in men, an endoscope’s length of approximately 40 cm is required.
The distal end of semirigid ureteroscopes has a circular or oval configuration ( Fig. 3.5 ). Recently, ureteroscopes with a triangular end have been described ( Fig. 3.6 ), facilitating the approach of the ureteral orifice.


3.3.2
Optical Systems
The optical systems of semirigid ureteroscopes include optic fibers similar to those used in flexible instruments. Due to the relatively large diameter, these instruments contain a large number of fibers, ensuring a higher image quality.
Initially, the image offered by these instruments had a low quality due to the low resolution and the “honeycomb” aspect produced by the optic fibers. The modern fiber layout and the progress of the video systems have solved these problems ( ).
Due to the flexibility of the optic fiber systems, these ureteroscopes can be angled, while at the same time ensuring a straight working channel that is necessary for rigid working instruments. Furthermore, the physical properties of this type of optical system allow the positioning of the viewfinder in the axis of the instrument, as well as eccentric to it ( Fig. 3.7 ).

In recent years, in the attempt to optimize the endoscopic image, digital semirigid ureteroscopes have been developed (video-ureteroscopes). These have at the distal end a CMOS- or CCD-type sensor that receives the information and transmits it in a digital format.
3.3.3
Working Channel
The working channel of modern semirigid ureteroscopes has a caliber between 2.1 F and 6.6 F. In general, the ureteroscopes have at least one channel with a diameter of 3.4 F that allows the passage of most accessory instruments, as well as a proper irrigation ( Fig. 3.8 ). Today, semirigid instruments with two working channels are usually used.

3.3.1
External Configuration
Usually, the distal end of semirigid ureteroscopes has a diameter between 6 F and 10 F. These dimensions allow performing ureteroscopic maneuvers without dilation of the intramural ureter. The sheath of most semirigid ureteroscopes has a progressively increasing diameter from the distal to the proximal end, where it reaches a caliber varying between 7.8 F and 14.5 F. This design facilitates the progressive dilatation of the ureter as the ureteroscope is advanced. The working length of the models used in practice is of over 31 cm. This length allows the approach of the distal and middle ureter, and in women it is sufficient to also approach the renal pelvis. For ureteroscopic interventions on the pyelocaliceal system in men, an endoscope’s length of approximately 40 cm is required.
The distal end of semirigid ureteroscopes has a circular or oval configuration ( Fig. 3.5 ). Recently, ureteroscopes with a triangular end have been described ( Fig. 3.6 ), facilitating the approach of the ureteral orifice.


3.3.2
Optical Systems
The optical systems of semirigid ureteroscopes include optic fibers similar to those used in flexible instruments. Due to the relatively large diameter, these instruments contain a large number of fibers, ensuring a higher image quality.
Initially, the image offered by these instruments had a low quality due to the low resolution and the “honeycomb” aspect produced by the optic fibers. The modern fiber layout and the progress of the video systems have solved these problems ( ).
Due to the flexibility of the optic fiber systems, these ureteroscopes can be angled, while at the same time ensuring a straight working channel that is necessary for rigid working instruments. Furthermore, the physical properties of this type of optical system allow the positioning of the viewfinder in the axis of the instrument, as well as eccentric to it ( Fig. 3.7 ).

In recent years, in the attempt to optimize the endoscopic image, digital semirigid ureteroscopes have been developed (video-ureteroscopes). These have at the distal end a CMOS- or CCD-type sensor that receives the information and transmits it in a digital format.
3.3.3
Working Channel
The working channel of modern semirigid ureteroscopes has a caliber between 2.1 F and 6.6 F. In general, the ureteroscopes have at least one channel with a diameter of 3.4 F that allows the passage of most accessory instruments, as well as a proper irrigation ( Fig. 3.8 ). Today, semirigid instruments with two working channels are usually used.

3.4
Flexible ureteroscopes
Although the first flexible endoscope was mentioned by Victor , flexible fibroscopes have been introduced relatively recently into current urological practice.
These ureteroscopes have a similar structure to other types of flexible endoscopes: deflection mechanism, working channel, noncoherent fiber optic fascicle(s) for transmission of light, and a coherent fascicle of optic fibers for image transmission ( Fig. 3.9 ).

3.4.1
External Configuration
The distal part of flexible ureteroscopes is usually conical, with a smooth surface. Some ureteroscopes have a beveled, triangular end, facilitating the approach of the ureteral orifice and reducing the risk of parietal lesions.
3.4.2
Deflection Mechanism
The first flexible ureteroscopes did not have active deflection capabilities, their deformation being achieved only passively, due to the external sheath’s elasticity. These mechanical properties only allowed the visualization of certain areas of the upper urinary tract, also accessible in most cases by rigid and semirigid ureteroscopes. Therefore, adding active deflection ( Fig. 3.10 ) came as a natural addition, within the efforts to provide the new generation of flexible ureteroscopes with a superior maneuverability inside the upper urinary tract.

Active deflection is obtained by handling a mobile piece located on the ureteroscope’s body with the thumb. Pushing it upward or downward ( Fig. 3.11 ) determines the tensioning of metal fibers that stretch along the entire working segment of the ureteroscope, achieving bidirectional deflection of the distal end.

This can be achieved in the direction of the pushing motion (an “intuitive” or “logical” deflection type, found in most American models), or against the direction of action (“counterintuitive,” “inversed logical,” or “international” deflection type, found in most European or Japanese models).
Modern flexible ureteroscopes have at least one area of active deflection, usually bidirectional. The high flexibility of the outer sheath in the distal segment allows for the appearance of a secondary, passive deflection area ( Fig. 3.12 ).

This is achieved by pushing the distal segment of the ureteroscope against the superior wall of the renal pelvis or against the implantation area of the middle or upper calyx in the pyelon, reason for which it can be limited by certain anatomic particularities or by the presence of certain pathological modifications (e.g., high-grade hydronephrosis).
The difficulties that arise during the attempts to approach certain areas of the pyelocaliceal system (especially the lower calyx) have required the upgrade of the active deflection system. A first concept was to create models with two active deflection segments that had superior maneuverability and were able to approach lesions that are sometimes inaccessible to the single deflection models ( ).
The first flexible ureteroscope to have these features was the ACMI DUR-8 Elite model (ACMI Corporation, Southborough, MA, USA) ( Fig. 3.13 ) that, in addition to the 170°/180° upward/downward deflection of the DUR-8 model, allowed it to obtain an additional secondary active downward deflection of 130°. The secondary deflection is achieved by actioning a second mobile piece placed on the opposite side of the flexible ureteroscope’s body.

The Karl Storz Company (Karl Storz Endoscopy, Tuttlingen, Germany) adopted a different concept for improving maneuverability. In the Storz Flex-X (11278AU1) ( Fig. 3.14 ) and Storz Flex-X2 models, the amplitude of the active deflection was increased (to 270° in both directions), as was its radius (the so-called “exaggerated deflection”) ( Fig. 3.15 ).


Similarly, the new models of flexible ureteroscopes from Olympus (Olympus, Melville, NY, USA) and Richard Wolf (Richard Wolf, Knittlingen, Germany) offer amplitudes of the active deflection of over 270°.
3.4.3
Optical and Light Transmission Systems
In conventional flexible ureteroscopes, the transmission of light and images is achieved through optic fiber fascicles. These are glass fibers of approximately 8 μm, having a central part with a higher refraction index than the individual coating. Due to this property ( Fig. 3.16 ), transmission of light becomes possible, even when the fibers are bent, a phenomenon called total internal reflection ( ).

If it is intended for the transmission of images, in order for them to be real, the optic fiber fascicle must have a coherent structure, with an identical disposition of the fibers at both ends. The resulting image is composed of the small images that are transmitted through each optic fiber.
Due to the fact that light is not transmitted through the individual coating, the final composed image will present a “honeycomb” effect (also named the “moiré” effect) ( Figs 3.17 and 3.18 ). The use of special filters eliminates this effect, but with the cost of further lowering the image quality.


The resolution is directly proportional to the number of optical fibers in the fascicles. Modern technology has allowed packing a greater number of fibers into bundles, thus increasing the quality of the images.
Despite these technological advances, the images transmitted through the optic fiber systems of flexible ureteroscopes continue to have a lower resolution compared to those transmitted through the optical systems of rigid and semirigid ureteroscopes. The images, however, are sufficient enough to carry out the endoscopic procedures in good conditions.
For the transmission of light, the coherent disposition of the bundle’s fibers is not required. The number of these noncoherent fascicles varies according to the model.
The field of view of flexible ureteroscopes varies between 60° and 90°. A narrow field of view may be compensated for by using active deflection. For most instruments the viewing angle is 0°. Some models have a 90° angle that allows for a better visibility of the working instruments ( ).
In the latest generation of flexible ureteroscopes, the conventional image transmission system has been replaced with a digital one. The ACMI DUR-D model, launched in September 2006, presents a CMOS-type digital sensor at its distal end. This receives the information and transmits it in digital format, through a single wire, to a processor that decodes it and projects it on the monitor. In addition, the noncoherent optic fiber fascicles used for the transmission of light have been replaced with a white light LED located at the distal end. Subsequently, other models of such flexible videoscopes were launched: the Olympus URF-Vo (Olympus, Melville, NY, USA) ( Figs 3.19 and 3.20 ) and the Storz Flex-XC (Karl Storz Endoscopy, Tuttlingen, Germany) ( Fig. 3.21 ).



The new generation of digital flexible ureteroscopes promises a superior flexibility and a much better visibility due to the replacement of the conventional optical system with all its inconveniences. Also, by removing these fragile components, the premises for a superior durability of the new endoscopes are created.
3.4.4
Working Channel
The working channel ensures the circulation of the irrigation fluid and allows the insertion of the accessory instruments and the probes or fibers of the energy sources for lithotripsy. Logically, the irrigation flow will be lowered directly proportional to the dimension of the accessory instruments or of the fibers that are inserted.
Most models of flexible ureteroscopes that are currently used have a 3.6 F working channel ( Table 3.1 ). An exception is represented by the Wolf 7330.072 model that has a 4.5 F working channel, in the conditions in which the diameter of the endoscope’s tip is of 9 F.
Manufacturing company | Storz | ACMI | ACMI | ACMI | Wolf | Mitsubishi | Olympus |
---|---|---|---|---|---|---|---|
Model | 11274AA | AUR-7 | DUR-8 | DUR-8 Elite | 7330.072 9 F | 971101 | URF-3 |
Diameter (F) | |||||||
Distal end | 7.5 | 7.2 | 6.75 | 8.7 | 6 | 7.9 | 6.9 |
Sheath (maximum) | 8.7 | 11 | 8.7 | 10.1 | 9 | 7.9 | 8.4 |
Working length (mm) | 700 | 650 | 650 | 640 | 600 | NA | 700 |
Optics | |||||||
Visual field (degrees) | 90 | 80 ± 5 | 80 | 80 ± 5 | 60 | 70 | 90 |
Visual angle (degrees) | 0 | 9 | 9 | 9 | 0 | 0 | 0 |
Image dimension (cm 2 ) | 20.4 | 22 | 22 | 22 | 22 | – | 25 |
Optical fibers | Standard | Standard | Standard | Standard | Quartz | Quartz | Standard |
Pixels/fiber | 3000 | – | 3400 | 3400 | 5000 | 6000 | 5500 |
Working channel | 3.6 | 3.6 | 3.6 | 3.6 | 4 | 3.6 | 3.6 |
Deflection mechanism | |||||||
Amplitude (degrees) | 170/120 | 120/160 | 170/180 | 300/310 | 160/130 | 134/170 | 180/180 |
International | Yes | No | No * | No * | Yes | Yes | Yes |
Secondary deflection | Passive | Passive | Passive | Active (130° downward) | Passive | Passive | Passive |
* The ACMI DUR-8 BA and DUR-8 Elite BA models have an international type deflection.
The Wolf Viper model (Richard Wolf, Knittlingen, Germany) has two separate working channels, so that the insertion of the accessory instruments no longer influences the flow of the irrigation fluid.
3.4.5
Future Perspectives
Solving the visibility and, partially, the reliability problems with the development of the digital flexible ureteroscopes were also unable to resolve the maneuverability and accessibility issues.
Minimization of the chips from the tip with the implicit reduction of the ureteroscope’s dimensions is still a problem under evaluation. Reducing the influence of the accessory instruments on irrigation and deflection is also a direction of research.
An instrument that approaches the entire problem in a different manner is the Polyscope (Lumenis, Yokneam, Israel). This is an 8 F modular, maneuverable flexible ureteroscope, with a separate optical system with a 10,000 pixel resolution and a length of 1500 mm. The optical system is inserted through a single-use multilumen catheter. This configuration ensures, according to the authors, a better cost-efficiency ratio and a lower risk of damaging the optical system during its use. However, the new device is still awaiting validation in current clinical practice ( Table 3.2 ).
Manufacturing company | Olympus | Storz | ACMI |
---|---|---|---|
Model | URF-Vo | Flex-XC | DUR-D |
Diameter (F) | |||
Distal end | 8.5 | 8.5 | 8.7 |
Sheath (maximum) | 9.9 | 8.5 | 9.3 |
Working length (mm) | 670 | 700 | 650 |
Optics | |||
Visual field (degrees) | 90 | 90 | 80 |
Visual angle (degrees) | 0 | 0 | 0 |
Working channel | 3.6 | 3.6 | 3.6 |
Deflection mechanism | |||
Amplitude (degrees) | 180/275 | 270/270 | 250/250 |
International | Yes | Yes | Yes |
3.4.2
Deflection Mechanism
The first flexible ureteroscopes did not have active deflection capabilities, their deformation being achieved only passively, due to the external sheath’s elasticity. These mechanical properties only allowed the visualization of certain areas of the upper urinary tract, also accessible in most cases by rigid and semirigid ureteroscopes. Therefore, adding active deflection ( Fig. 3.10 ) came as a natural addition, within the efforts to provide the new generation of flexible ureteroscopes with a superior maneuverability inside the upper urinary tract.

Active deflection is obtained by handling a mobile piece located on the ureteroscope’s body with the thumb. Pushing it upward or downward ( Fig. 3.11 ) determines the tensioning of metal fibers that stretch along the entire working segment of the ureteroscope, achieving bidirectional deflection of the distal end.

This can be achieved in the direction of the pushing motion (an “intuitive” or “logical” deflection type, found in most American models), or against the direction of action (“counterintuitive,” “inversed logical,” or “international” deflection type, found in most European or Japanese models).
Modern flexible ureteroscopes have at least one area of active deflection, usually bidirectional. The high flexibility of the outer sheath in the distal segment allows for the appearance of a secondary, passive deflection area ( Fig. 3.12 ).

This is achieved by pushing the distal segment of the ureteroscope against the superior wall of the renal pelvis or against the implantation area of the middle or upper calyx in the pyelon, reason for which it can be limited by certain anatomic particularities or by the presence of certain pathological modifications (e.g., high-grade hydronephrosis).
The difficulties that arise during the attempts to approach certain areas of the pyelocaliceal system (especially the lower calyx) have required the upgrade of the active deflection system. A first concept was to create models with two active deflection segments that had superior maneuverability and were able to approach lesions that are sometimes inaccessible to the single deflection models ( ).
The first flexible ureteroscope to have these features was the ACMI DUR-8 Elite model (ACMI Corporation, Southborough, MA, USA) ( Fig. 3.13 ) that, in addition to the 170°/180° upward/downward deflection of the DUR-8 model, allowed it to obtain an additional secondary active downward deflection of 130°. The secondary deflection is achieved by actioning a second mobile piece placed on the opposite side of the flexible ureteroscope’s body.

The Karl Storz Company (Karl Storz Endoscopy, Tuttlingen, Germany) adopted a different concept for improving maneuverability. In the Storz Flex-X (11278AU1) ( Fig. 3.14 ) and Storz Flex-X2 models, the amplitude of the active deflection was increased (to 270° in both directions), as was its radius (the so-called “exaggerated deflection”) ( Fig. 3.15 ).


Similarly, the new models of flexible ureteroscopes from Olympus (Olympus, Melville, NY, USA) and Richard Wolf (Richard Wolf, Knittlingen, Germany) offer amplitudes of the active deflection of over 270°.
3.4.3
Optical and Light Transmission Systems
In conventional flexible ureteroscopes, the transmission of light and images is achieved through optic fiber fascicles. These are glass fibers of approximately 8 μm, having a central part with a higher refraction index than the individual coating. Due to this property ( Fig. 3.16 ), transmission of light becomes possible, even when the fibers are bent, a phenomenon called total internal reflection ( ).

If it is intended for the transmission of images, in order for them to be real, the optic fiber fascicle must have a coherent structure, with an identical disposition of the fibers at both ends. The resulting image is composed of the small images that are transmitted through each optic fiber.
Due to the fact that light is not transmitted through the individual coating, the final composed image will present a “honeycomb” effect (also named the “moiré” effect) ( Figs 3.17 and 3.18 ). The use of special filters eliminates this effect, but with the cost of further lowering the image quality.


The resolution is directly proportional to the number of optical fibers in the fascicles. Modern technology has allowed packing a greater number of fibers into bundles, thus increasing the quality of the images.
Despite these technological advances, the images transmitted through the optic fiber systems of flexible ureteroscopes continue to have a lower resolution compared to those transmitted through the optical systems of rigid and semirigid ureteroscopes. The images, however, are sufficient enough to carry out the endoscopic procedures in good conditions.
For the transmission of light, the coherent disposition of the bundle’s fibers is not required. The number of these noncoherent fascicles varies according to the model.
The field of view of flexible ureteroscopes varies between 60° and 90°. A narrow field of view may be compensated for by using active deflection. For most instruments the viewing angle is 0°. Some models have a 90° angle that allows for a better visibility of the working instruments ( ).
In the latest generation of flexible ureteroscopes, the conventional image transmission system has been replaced with a digital one. The ACMI DUR-D model, launched in September 2006, presents a CMOS-type digital sensor at its distal end. This receives the information and transmits it in digital format, through a single wire, to a processor that decodes it and projects it on the monitor. In addition, the noncoherent optic fiber fascicles used for the transmission of light have been replaced with a white light LED located at the distal end. Subsequently, other models of such flexible videoscopes were launched: the Olympus URF-Vo (Olympus, Melville, NY, USA) ( Figs 3.19 and 3.20 ) and the Storz Flex-XC (Karl Storz Endoscopy, Tuttlingen, Germany) ( Fig. 3.21 ).



The new generation of digital flexible ureteroscopes promises a superior flexibility and a much better visibility due to the replacement of the conventional optical system with all its inconveniences. Also, by removing these fragile components, the premises for a superior durability of the new endoscopes are created.
3.4.4
Working Channel
The working channel ensures the circulation of the irrigation fluid and allows the insertion of the accessory instruments and the probes or fibers of the energy sources for lithotripsy. Logically, the irrigation flow will be lowered directly proportional to the dimension of the accessory instruments or of the fibers that are inserted.
Most models of flexible ureteroscopes that are currently used have a 3.6 F working channel ( Table 3.1 ). An exception is represented by the Wolf 7330.072 model that has a 4.5 F working channel, in the conditions in which the diameter of the endoscope’s tip is of 9 F.
Manufacturing company | Storz | ACMI | ACMI | ACMI | Wolf | Mitsubishi | Olympus |
---|---|---|---|---|---|---|---|
Model | 11274AA | AUR-7 | DUR-8 | DUR-8 Elite | 7330.072 9 F | 971101 | URF-3 |
Diameter (F) | |||||||
Distal end | 7.5 | 7.2 | 6.75 | 8.7 | 6 | 7.9 | 6.9 |
Sheath (maximum) | 8.7 | 11 | 8.7 | 10.1 | 9 | 7.9 | 8.4 |
Working length (mm) | 700 | 650 | 650 | 640 | 600 | NA | 700 |
Optics | |||||||
Visual field (degrees) | 90 | 80 ± 5 | 80 | 80 ± 5 | 60 | 70 | 90 |
Visual angle (degrees) | 0 | 9 | 9 | 9 | 0 | 0 | 0 |
Image dimension (cm 2 ) | 20.4 | 22 | 22 | 22 | 22 | – | 25 |
Optical fibers | Standard | Standard | Standard | Standard | Quartz | Quartz | Standard |
Pixels/fiber | 3000 | – | 3400 | 3400 | 5000 | 6000 | 5500 |
Working channel | 3.6 | 3.6 | 3.6 | 3.6 | 4 | 3.6 | 3.6 |
Deflection mechanism | |||||||
Amplitude (degrees) | 170/120 | 120/160 | 170/180 | 300/310 | 160/130 | 134/170 | 180/180 |
International | Yes | No | No * | No * | Yes | Yes | Yes |
Secondary deflection | Passive | Passive | Passive | Active (130° downward) | Passive | Passive | Passive |
* The ACMI DUR-8 BA and DUR-8 Elite BA models have an international type deflection.
The Wolf Viper model (Richard Wolf, Knittlingen, Germany) has two separate working channels, so that the insertion of the accessory instruments no longer influences the flow of the irrigation fluid.
3.4.5
Future Perspectives
Solving the visibility and, partially, the reliability problems with the development of the digital flexible ureteroscopes were also unable to resolve the maneuverability and accessibility issues.
Minimization of the chips from the tip with the implicit reduction of the ureteroscope’s dimensions is still a problem under evaluation. Reducing the influence of the accessory instruments on irrigation and deflection is also a direction of research.
An instrument that approaches the entire problem in a different manner is the Polyscope (Lumenis, Yokneam, Israel). This is an 8 F modular, maneuverable flexible ureteroscope, with a separate optical system with a 10,000 pixel resolution and a length of 1500 mm. The optical system is inserted through a single-use multilumen catheter. This configuration ensures, according to the authors, a better cost-efficiency ratio and a lower risk of damaging the optical system during its use. However, the new device is still awaiting validation in current clinical practice ( Table 3.2 ).
Manufacturing company | Olympus | Storz | ACMI |
---|---|---|---|
Model | URF-Vo | Flex-XC | DUR-D |
Diameter (F) | |||
Distal end | 8.5 | 8.5 | 8.7 |
Sheath (maximum) | 9.9 | 8.5 | 9.3 |
Working length (mm) | 670 | 700 | 650 |
Optics | |||
Visual field (degrees) | 90 | 90 | 80 |
Visual angle (degrees) | 0 | 0 | 0 |
Working channel | 3.6 | 3.6 | 3.6 |
Deflection mechanism | |||
Amplitude (degrees) | 180/275 | 270/270 | 250/250 |
International | Yes | Yes | Yes |
3.5
Energy sources
3.5.1
Ballistic Lithotripsy
Ballistic lithotripsy represents a stone destruction method in which the pulsatile compression produced by air or electromechanic forces is transmitted through a metallic rod. The first ballistic lithotriptor was represented by the Swiss lithoclast, introduced into practice at the beginning of the 1990s. The pulsations produced using compressed air lead to the movement of a metallic sphere that induces impulses of the probe with a frequency of 12 cycles/s, determining the repeated contact between the probe and the stone ( ). Subsequently, the electrokinetic lithotriptor was developed ( Fig. 3.22 ), which works by creating an electromagnetic field that determines vibrations of the probe with a frequency of 15–30 cycles/s. Comparative studies regarding the efficiency of the two types of ballistic lithotripsy have shown similar results with regard to stone fragmentation, as well as equivalent rates of proximal migration of the fragments and of complications ( ).

The probes used by the ballistic lithotriptor may have a diameter between 2.4 F and 6 F, and can be used for semirigid or rigid ureteroscopy. Compared to the electrohydraulic, ultrasonic, or laser lithotripsy, ballistic lithotripsy has a lower risk of ureteral perforations ( ), the mean rate of occurrence for this complication being lower than 1% across different studies ( ).
Another advantage of ballistic lithotripsy is represented by the relatively low costs and the easy maintenance of the equipment ( ).
The disadvantages of ballistic lithotripsy are determined by the rigidity of the probes, which does not allow their use during flexible ureteroscopy. Also, ballistic lithotripsy is associated with a rate of ascendent stone migration varying between 2% and 17%.
Recently, ballistic lithotriptors have been improved by attaching a suction pump that facilitates the evacuation of fragments ( ) and by using nitinol probes that allow their use during flexible ureteroscopy ( ).
Data regarding the benefits of associating aspiration during lithotripsy for preventing ascendent migration are limited ( ).
3.5.2
Ultrasonic Lithotripsy
Ultrasonic lithotripsy ( Fig. 3.23 ) is a safe and efficient procedure that achieves a controlled stone destruction and allows for the rapid evacuation of the resulted fragments under endoscopic control.

The idea of using ultrasounds in lithotripsy belongs to . The attempt to fragment the stones using sound waves with a frequency of 0.8 kHz failed. In 1955, Coates achieved in vitro lithotripsy, using 15 kHz sound waves. After 15 years, Trehorst is the first to fragment a bladder stone in vivo using ultrasounds. In 1979, Marberger and Alken, using energies of 23–25 kHz, introduced ultrasound lithotripsy in the percutaneous treatment of renal lithiasis.
The principle of this method consists of transforming electrical energy into ultrasonic energy. The electrical current produced by a generator is transmitted to the transducer, determining the excitation of a piezoelectric crystal. The crystal vibrates at a specific frequency, generating an acoustic wave with the frequency of 23–25 kHz. At the operating frequencies, high-pitch sounds can be heard, having different metallic tones that vary according to the amount of liquid that has been suctioned and to the pressure gradient exerted by the sonotrode on the stone. However, there is also a high level of sound, imperceptible to the human ear, that can reach an intensity of 98 dB ( ).
The resultant ultrasonic waves are transmitted longitudinally through the sonotrode to the stone. In contact with the probe, the stone vibrates until it is fragmented. Placing the sonotrode in contact with the urothelium causes minimal lesions, due to the fact that the tissues do not resonate at the vibrating energy ( ). Although heating of the probe’s tip occurs during lithotripsy, at an irrigation flow of 30 mL/min, the rise in temperature is of maximum 1.4°C ( ). The flow of the irrigation fluid during ureteroscopy can be limited, and for this reason ultrasonic lithotripsy is recommended especially during percutaneous interventions. A suction pressure of 60–80 cm H 2 O is sufficient to maintain a proper irrigation flow during lithotripsy. Higher suction pressures may lead to the emergence of air bubbles, with the consecutive impairment of visibility.
The lithotripsy probes have a diameter between 2.5 F and 12 F. The 2.5-F probes are not fitted with an irrigation channel because this can lead to heating. Bending of the probe determines a loss of energy at the convexity area, with the consecutive reduction of efficiency ( ).
The major advantage of ultrasonic lithotripsy is represented by the efficient combination between stone destruction and fragment removal. Those smaller than 2 mm are suctioned through the probe’s channel along with the irrigation fluid, while larger fragments can be extracted with forceps or with the basket probe.
The rigidity and diameter of the probes limit the use of this technology in the treatment of ureteral stones. However, the success rate of ultrasonic lithotripsy reported in the literature varies between 69% and 100% ( ). This technique may be used with good results for large ureteral stones and, in the case of the steinstrasse syndrome, for the easy extraction of fragments. Favorable results have also been reported for distal ureteral stones that can be approached with rigid ureteroscopes ( ).
3.5.3
Electrohydraulic Lithotripsy
Electrohydraulic lithotripsy ( Fig. 3.24 ) is an efficient and safe renal and ureteral lithotripsy method, but is used less frequently than the ultrasonic method. Taking into account the electrodes’ flexibility, the largest field of application is represented by antegrade or retrograde flexible endoscopy.

The first electrohydraulic lithotriptor, Urat-I (YPAT-1), was developed in 1955 by Yutkin, at the Kiev University, being initially used only in the Eastern European Bloc ( ). In 1960, Rose and Goldberg used electrohydraulic lithotripsy to fragment bladder stones. The first clinical application of this type of lithotripsy for renal stones was performed during a pyelolithotomy by . Subsequently, Lawson, Clayman, and other authors successfully used the method for large renal stones.
In 1985, Lytton and coworkers reported the use of electrohydraulic lithotripsy in the ureteroscopic treatment of ureteral lithiasis, without early or late postoperative complications.
Although the first electrohydraulic probes had a large diameter (9 F), the diameter was reduced to 1.5–5 F as a result of technical advances.
Using small caliber probes ( Fig. 3.25 ) allows for better irrigation. Different probe diameters have comparable efficiency, although the electric resistance of the larger probes is lower ( ). Electrohydraulic lithotripsy was first used during flexible ureteroscopy by .

Electrohydraulic lithotripsy allows for stone fragmentation through shockwaves generated by an electrical discharge in a liquid medium. The probes consist of two coaxial electrodes, isolated from each other and from the external medium. The electrical discharge between them generates a spark at the tip of the probe, releasing thermal energy that develops a large amount of caloric energy in a small space.
In the liquid medium, the intense heat determines the vaporization of a small quantity of the irrigation fluid. Due to the gas bubble formation at the tip of the probe, the liquid is pushed with force, hence resulting the hydraulic shockwaves. The impact of the shockwaves with the stone is sufficient to crack it. A second and third shockwave are produced indirectly, as a result of the first wave’s recoil. These sequences are carried out with a rhythmicity of 1/800 per second, as long as the electrode is activated.
Depending on the distance between the lithotripsy probe and the stone’s surface, the gas bubble may collapse in a symmetrical manner (if the distance is of approximately 1 mm), generating a powerful shockwave, or asymmetrically (if the distance is of approximately 3 mm), propelling a jet of liquid ( ).
Due to the risk of damaging the optical system, the electrohydraulic lithotripsy probe should be discharged at least 2–5 mm from the tip of the ureteroscope ( ).
The amount of energy obtained by the electrical discharge at the tip of the probe depends on the voltage and capacitance. Vorreutger’s experimental studies showed that the maximum pressure, as well as the speed with which this is obtained, depends only on the voltage, the capacitance influencing the persistence of the shockwave over time ( ; Vorreuther and Engelmann, 1995). Therefore, through the variations of these two parameters, shockwaves with maximum pressure and with different degrees of persistence over time are obtained, although the influence of these patterns on the efficiency of the lithotripsy is still insufficiently assessed ( ).
Although initially it was considered that the optimal irrigation fluid is represented by a 1/6–1/7 saline solution, electrohydraulic lithotripsy may also be performed using standard saline ( ). When using pure water as an irrigation fluid, the efficiency drops due to the generation of an inefficient spark. It is also important for the temperature of the irrigation fluid to be around 37°C.
The use of electrohydraulic lithotripsy comes with a series of advantages:
- •
reduced acquisition and usage costs in comparison with the other lithotriptors
- •
widely accessible method
Unfortunately, there are a series of disadvantages that limit the performances of the method:
- •
a higher aggression on the surrounding tissues and on the optical systems of the ureteroscopes
- •
the possibility of deteriorating the probes during use, with the need to remove the detached fragments (parts of the external isolating layer, distal metallic ends, etc.)
- •
single-use probes
- •
poorer results for the fragmentation of stones with certain chemical compositions
The major disadvantage of electrohydraulic lithotripsy is the need to maintain a safe distance in order to prevent mucosal lesions or ureteral perforation. In a study regarding the results of electrohydraulic lithotripsy using 9 F probes in the treatment of distal ureteral stones, obtained a 90% success rate, but with an incidence of urinary leakage of 40%.
Despite the technical advances, ureteral perforation continues to represent a frequent complication. reported, in a prospective study on a group of 72 patients, a 17.6% rate of perforations after electrohydraulic lithotripsy, in comparison with 2.6% for pneumatic lithotripsy. Vorreuther (1995) suggested that the mechanism of lesion occurrence consists in the expansion of bubbles, a phenomenon that takes place even if the probe does not come into direct contact with the ureteral mucosa. The diameter of the cavitation bubbles depends on the energy used and can exceed 1.5 cm in instances of energies higher than 1300 mJ. For this reason, the risk of perforations is increased in case of rough stones, which require high energy levels. Even in the case of small caliber probes, perforation can occur if a high number of impulses is used close to the mucosa. In a comparative in vitro study, observed that electrohydraulic lithotripsy is associated with a high rate of ureteral perforation, in comparison with pulsed laser or with ballistic lithotripsy. The risk of this complication increases in case of impacted stones with important edema or when the visibility is reduced due to bleeding secondary to the lithotripsy ( ).
Similar to the other lithotripsy methods, electrohydraulic fragmentation determines the retrograde propulsion of the fragments ( ). A disadvantage compared to the use of the Ho:YAG laser is represented by the dimensions of the resulted fragments, especially in case of stones larger than 15 mm.
The advantages of electrohydraulic lithotripsy include the flexibility of the probes (especially the ones with a low caliber of 1.6–1.9 F), which allows performing intracorporeal lithotripsy by rigid or flexible ureteroscopy.
For each case, an average number of 1–1.3 probes is required, with the exception of rough stones (calcium oxalate monohydrate) that can require more than two probes ( ).
3.5.4
Lasers
A series of lasers, with different wavelengths, have application in urology ( Fig. 3.26 ).

The basic principle of laser technology (light amplification by stimulated emission of radiation) is represented by photon emission, produced by the stimulation of atoms by external energy sources. Due to this excitation, the electrons shift their orbit, their recovery to their initial state being achieved with emission of energy under the form of photons.
The laser was introduced into urological practice for the first time in 1968 by Mulvaney and Beck, who used a ruby laser to fragment stones ( ). This type of laser generates a continuous beam, with the emission of excessive heat, a fact that prevents its use in practice.
Lasers with pulsatile-type emission are currently used for lithotripsy. These lasers deliver energy in short bursts, therefore obtaining a high power without heating the application area, thus avoiding tissue damage. In theory, lasers with continuous-type emission, such as neodymium:YAG (Nd:YAG), could also be used for stone fragmentation, but the overheating of the application area produced by the emission of energy for a long period of time limits their use for this purpose ( ).
The first laser that became used as an energy source for lithotripsy was the “pulsed-dye” type. This uses a cumarinic dye that, stimulated by light quanta, emits green light pulses with a duration of 1 μs and a wavelength of 504 nm. This wavelength was chosen because it is absorbed by most stones (excluding cystine calculi), without being absorbed by the surrounding tissues ( ). The “pulsed-dye” laser works by producing a plasma bubble, whose expansion and collapse generates a shockwave that can determine the fragmentation of the stone. This type of effect is called the photoacoustic effect ( ) and characterizes most pulsatile lasers, with the exception of the holmium:YAG (Ho:YAG) and erbium:YAG (Er:YAG) lasers.
Another type of laser that can be used for lithotripsy is FREDDY (frequency-doubled double-pulse Nd:YAG), a modified variant of Nd:YAG that can also emit at 532 nm (half of its wavelength). FREDDY is a solid laser with long pulses, double frequency, emitting wavelengths at 532 and 1064 nm that produce stone fragmentation by a photoacoustic mechanism ( ). This presents the advantages of low costs and, due to its wavelength, minimal risk of tissular lesions. The laser uses silicon fibers that are highly elastic, a property that makes them ideal for use with flexible ureteroscopes ( ). The efficiency of FREDDY lithotripsy is influenced by the stones’ chemical composition, being lower for cystine or calcium oxalate monohydrate ( ).
The Ho:YAG laser ( Fig. 3.27 ), with pulsed emission, is currently considered the most efficient energy source for intracorporeal lithotripsy.

Unlike the previously presented types, the Ho:YAG laser has a wavelength of 2140 nm, pulses with a substantially longer duration (250–350 μs), generates nonspherical plasma bubbles (pear-shaped), and produces weak shockwaves. Moreover, some studies show that lithotripsy begins before the collapse of the plasma bubble and the production of the shockwave ( ).
All this data led to the theory that the true mechanism of lithotripsy in the case of the Ho:YAG laser is the photo-thermal effect that determines the vaporization of the stones, the photoacoustic effect having an insignificant role ( ).
Most of the energy of Ho:YAG lasers is superficially absorbed, determining a low penetrability between 0.5 mm and 1 mm ( ). Lithotripsy does not occur when the fiber is applied on the stone at a 90° angle. The Ho:YAG laser can be used on flexible endoscopes, allowing the fragmentation of stones regardless of their localization in the upper urinary tract. The efficiency of Ho:YAG laser lithotripsy varies between 91% and 100% and is not influenced by the chemical composition of the stone. Also, lithotripsy determines the formation of small fragments that do not require additional extraction maneuvers. The mean rates of perforations and of postoperative stenoses are of 1.1 and 1.2%, respectively.
Another advantage of this type of laser is represented by the possibility of using it for incisions of the upper urinary tract. The low penetrability, of only 0.5–1 mm, provides the Ho:YAG with a very good safety profile, currently being one of the preferred modalities for performing these incisions.
The major disadvantage in using the Ho:YAG laser is represented by the high costs of the equipment and fibers.
Due to the photo-thermal effect, various chemical reactions take place during lithotripsy, depending on the chemical composition of the stone. Thus, during lithotripsy of uric acid stones, production of cyanide has been demonstrated, but in low amounts and without clinical significance ( ).
A new type of laser is the Er:YAG ( Fig. 3.28 ), which acts through a photo-thermal effect similar to that of the Ho:YAG laser. Er:YAG has a 2940 nm wavelength, which is absorbed more efficiently by urinary stones than the 2140 nm wavelength of the Ho:YAG ( ). Experimental studies are currently still under way with regard to the development of fibers for this type of laser, for use during endoscopic interventions.

Nd:YAG ( Fig. 3.29 ) is a continuous emission laser with a wavelength of 1064 nm. The main tissular effect is represented by coagulation necrosis. Nd:YAG has a tissue penetration depth of 5–6 mm and excellent hemostasis capabilities. However, due to this penetrability, its safety margin is much lower than that of the Ho:YAG laser, imposing caution during its use in the upper urinary tract.

The Nd:YAG laser can be used to perform incisions or for tumor tissue destruction. For optimal effects, the laser fiber ( Fig. 3.30 ) must not come into contact with the tumor.

3.5.5
Energy Sources for Electrosurgery
Electrosurgery applies the use of alternative radiofrequency currents for cutting and/or coagulating tissues.
The electrosurgical devices are monopolar or bipolar. In the case of the first type, the electric current from the electrode placed on the working instrument reaches the site of the lesion and from here, through the patient’s body, reaches a second electrode, placed externally, most frequently on the buttock or thigh. In the case of bipolar systems, the current from the site of the lesion returns to a second electrode that is also located on the working instrument. This particularity reduces the extent of the deep tissular lesions or of those lateral to the application area ( ).
Electrosurgery generators are able to generate three types of current: cutting, coagulation, and combined. Therefore, with the aid of the electric current, one can perform the cauterization of bleeding lesions, the incision of upper urinary tract stenoses, and resection and/or coagulation of tumoral tissue, etc.
The electric current can be applied at the site of the lesion using a wide palette of accessory instruments.
Uretero-resectoscopes ( Fig. 3.31 ) are rigid endoscopes with a diameter ranging from 10.5 F to 13 F, with a mobile working element similar to that of the resectoscopes used in low endourology. Electrodes for tumor resection or knives for electric incisions (hot-knife) ( Fig. 3.32 ) or for cold-blade incisions (cold-knife) of the stenosis areas can be adapted on the working element.


The electrodes can be used for incision, cauterization, or electrovaporization. They have dimensions ranging from 3 F to 5 F, can be monopolar or bipolar, and can be used on rigid or semirigid ureteroscopes, as well as on flexible ones. Currently there are several types of such electrodes: Hulbert (Cook Urological, Spencer, IN), Bugbee™ and RiteCut™ (Gyrus ACMI, Southborough, MA), and Stryker (Stryker, San Jose, CA), among others. The RiteCut cutting device ( Fig. 3.33 ) has the particularity of presenting an angulation of the distal end, which makes it easier to apply to the wall of the upper urinary tract, increasing the precision and safety of the incisions. The 3 F caliber and the high flexibility allow for its efficient use during flexible ureteroscopy.

Another device for applying electric current to the upper urinary tract is the Acucise® (Applied Medical, Rancho Santa Margarita, CA), a hybrid between a monopolar electrode and a dilatation balloon ( Fig. 3.34 ). The system is composed of a 6/10 F catheter, with a low-pressure distal balloon (1 atm) with a caliber of 7/13 F (24 F when inflated) to which a filiform electrode is longitudinally applied. This configuration allows one to apply the cutting thread in the desired location and to perform a linear incision, at the same time associating the dilatation and hemostasis effects of the inflated balloon.

3.5.1
Ballistic Lithotripsy
Ballistic lithotripsy represents a stone destruction method in which the pulsatile compression produced by air or electromechanic forces is transmitted through a metallic rod. The first ballistic lithotriptor was represented by the Swiss lithoclast, introduced into practice at the beginning of the 1990s. The pulsations produced using compressed air lead to the movement of a metallic sphere that induces impulses of the probe with a frequency of 12 cycles/s, determining the repeated contact between the probe and the stone ( ). Subsequently, the electrokinetic lithotriptor was developed ( Fig. 3.22 ), which works by creating an electromagnetic field that determines vibrations of the probe with a frequency of 15–30 cycles/s. Comparative studies regarding the efficiency of the two types of ballistic lithotripsy have shown similar results with regard to stone fragmentation, as well as equivalent rates of proximal migration of the fragments and of complications ( ).

The probes used by the ballistic lithotriptor may have a diameter between 2.4 F and 6 F, and can be used for semirigid or rigid ureteroscopy. Compared to the electrohydraulic, ultrasonic, or laser lithotripsy, ballistic lithotripsy has a lower risk of ureteral perforations ( ), the mean rate of occurrence for this complication being lower than 1% across different studies ( ).
Another advantage of ballistic lithotripsy is represented by the relatively low costs and the easy maintenance of the equipment ( ).
The disadvantages of ballistic lithotripsy are determined by the rigidity of the probes, which does not allow their use during flexible ureteroscopy. Also, ballistic lithotripsy is associated with a rate of ascendent stone migration varying between 2% and 17%.
Recently, ballistic lithotriptors have been improved by attaching a suction pump that facilitates the evacuation of fragments ( ) and by using nitinol probes that allow their use during flexible ureteroscopy ( ).
Data regarding the benefits of associating aspiration during lithotripsy for preventing ascendent migration are limited ( ).
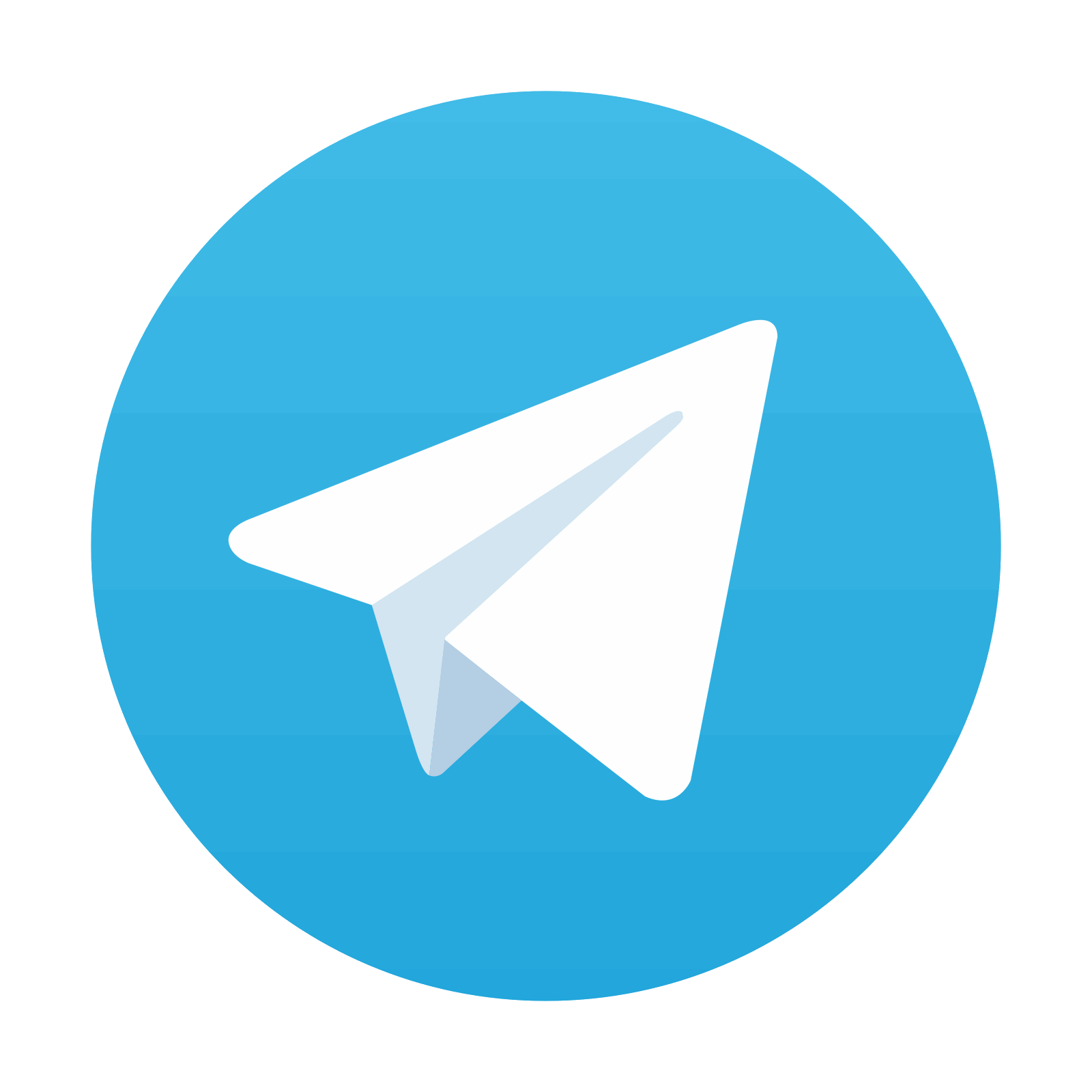
Stay updated, free articles. Join our Telegram channel

Full access? Get Clinical Tree
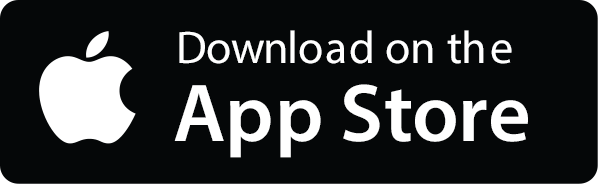
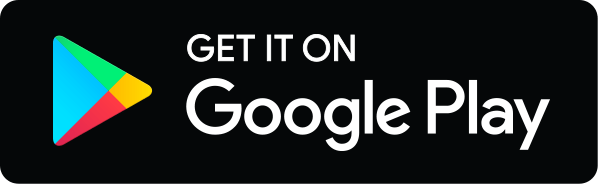