div class=”ChapterContextInformation”>
14. Urologic Instrumentation: Endoscopes and Lasers
Keywords
LaserCystoscopeUreteroscopeBenign prostate hyperplasiaNephrolithiasisEnhanced cystoscopyNephroscopeIntroduction
The impact of endoscopic access on the field of urology cannot be overstated. In an increasing number of urologic pathology, endoscopic techniques have replaced morbid open procedures such as open pyelolithotomy, open simple prostatectomy, and stricture repair [1]. This increasing effectiveness is due in large part to ever improving endoscopic visualization and miniaturization of instruments. This chapter covers the fundamental principles of lower and upper urologic endoscopy. In addition, we discuss laser energy sources which have revolutionized the capabilities for endoscopic management . We also review new advances and the future potential of endoscopic intervention. It is our hope that upon reading this chapter one will not only have a firm grasp of the fundamentals of urologic endoscopy and manipulation, but a command sufficient to handle any situation.
Lower Urinary Tract: Cystourethroscopy
Cystourethroscopy is the sine qua non of evaluation of lower urinary tract disorders. It is mandatory in the workup of hematuria and invaluable in assessment of lower urinary tract symptoms. In the most abstract sense, all cystoscopy involves a means of (1) generating an image and (2) a light source.
The first cystoscopes utilized poor light sources and did not magnify their images. In 1805, during the Napoleonic Wars, German army surgeon Philip Bozzini created the first known cystoscope. The image generator was a “viewing funnel” projecting to a sharkskin covered box and the light source was a candle within the box. By 1853, after further incremental improvements, Antoine Desmormeaux performed the first urologic endoscopic manipulation when he excised a urethral papilloma using his device, a endoscope with the light source in the handle. In 1877, Maximillian Carl-Friedrich Nitze and Joseph Leiter teamed up to produce a cystoscope where the light source was located at the tip of the instrument. By 1930, Physicist Harold Hopkins developed glass fibers capable of carrying images and light. As glass is better at transmitting light than air, Hopkins replaced the tube of air with a tube of glass and by 1959, with Karl Storz, developed the first fiberoptic cold-light source. This improved both light source and image generation [2].
With further development of fiber optic bundles we reached today’s cystoscopes. Cystoscopes come in two forms, flexible and rigid. The diversity of options for manipulation and models are too numerous to adequately enumerate, so we will heel to the basic abstraction of the components of a modern cystoscope: (1) a means to create an image (2) a light source and (3) in modern cystoscopes there is also a means to provide irrigation and sometimes drainage.
Rigid Cystoscopy
The rigid cystoscope consists of a telescope and sheath which acts as a flow channel for irrigation. Within or connected to the sheath one can place a working bridge, obturator, or working element. Traditionally, an obturator is used to reduce trauma associated with insertion and blunt the sharp edges of the sheath on insertion to prevent shearing. Once inside the bladder, the obturator is removed and a working bridge is inserted to allow passage of treatment implements or a working element is attached to allow for manipulation of tissue with instrumentation.
The traditional cystoscope is a “rod and lens” construction, with a single fiber optic or lens. This allows for a higher resolution image than the “fiber bundle ” of a flexible cystoscope. Semi-rigid ureteroscopes utilize a bundled fiber approach, as do some smaller cystoscopes. Understanding if an endoscope is a fiber based or rod and lens scope allows the surgeon to adjust focus and expected manipulation; a fiber bundle scope will have a honeycomb appearance but allows for some deflection of the scope without distortion of the image.

Richard Wolf 4.5/6.5 fr pediatric cystoscope, note shorter length
Within the sheath sits the telescope. Universally, the light source for cystoscopes remain either within the handle (for digital scopes) or a separate light box. The light is then transmitted through a fiber cable. This allows for the production of more lumens than a light within the tip of the scope. The rigid lens then comes in multiple angles. The 0° lens allows the best view of the urethra and is used primarily for urethral manipulation such as direct visualized internal urethrotomy. The 30° lens provides visualization of the base and anterolateral aspect of the bladder and is the standard for most endoscopic bladder manipulation in urology. Most endoscopic working elements are designed such that the active element is always within view of a 30° lens. A 70° lens allows for improved visualization of the dome and anterior bladder neck, though makes visualization of instruments difficult. The angled lens and light source fiber represent “blind spots” in the scope that the surgeon must remember during the procedure. Through these blind spots a surgeon can create trauma to or impact tissue without visualizing its presence.
In general, the surgeon should pick the sheath size that is the smallest to perform the desired task. This is a function of the costs and benefits of a particular size. For example, as discussed above, the light source is transmitted through a fiber conduit in the cystoscope. Therefore, smaller scopes will accommodate smaller light conduits, decreasing lighting and therefore visualization. Additionally, smaller scopes tend to have less robust irrigation also leading to worse visualization. This can lead to increased operative time for which there is evidence of increasing risk of stricture [3]. Conversely, it is a clinical principle that manipulation with larger instruments through a urethra increases the risk for urethral or fossa navicularis stricture. There is some data to support this principle, though limited [4]. Gunes et al. performed a retrospective study of 61 men undergoing transurethral resection of prostate using either a 24 or 26 fr resectoscope. The rate of bulbar stricture was 3% versus 11%, respectively, and statistically significant on univariate analysis [5]. Ultimately, we recommend the largest sheath that remains comfortable while providing adequate visualization and manipulation.
Flexible Cystoscopy
In comparison, flexible cystoscopes do not have the option for continuous irrigation. Yet, they present significant advantages for patient tolerance and visibility with their ability to deflect up to 220°. They are also better tolerated by patients, particularly male patients where the flexion of the flexible cystoscope can more easily overcome the bladder neck and prostatic urethral curves. In a flexible cystoscope, the irrigation connection and working port are on the instrument’s shaft and typically connect to a single common rubber-lined working channel within the scope. Functionally, this puts the cystoscope at risk for damage if instrumentation is passed with the scope in the deflected position; the instrument can perforate the inner channel.
Traditional analog flexible cystoscopes utilize a bundled fiber approach leading to an eyepiece. This is then connected to a light cord and camera or can be viewed directly. Increasingly, flexible cystoscopes utilize digital image generation. The digital cystoscope operates by a “chip on stick” approach where a video sensor is placed on the working tip of the cystoscope. The first digital cystoscopes were present in the 1970s and reached full commercial viability around 2005 [6]. The digital cystoscope allows for a higher resolution image and avoids the honeycomb effect of an analog bundled fiber. Using an early version of ACMI digital cystoscopes against analog cystoscopes, Quayle et al. performed a simple in-vitro study of resolution. The cystoscopes were placed a fixed position inside a cell culture plate with a set of numbers printed in a size 4 font in the back. Participants were asked to use the cystoscopes to identify the numbers. As the solution concentration increased, the digital cystoscope had visualization of 100% even as the best analog scope had 60% visualization [6]. The other relative merits, including price and durability are discussed in the ureteroscope section.
Despite the improved quality, a consideration should be kept for portability. Digital cystoscopes generally require large towers and, at the least, a reliable external power source. However, technology advances in consumer electronics, especially in the era of smart phones, has allowed analog cystoscopes to gain new portability. Multiple teams have developed attachments that allow for an analog cystoscope to be attached to a smartphone either to provide a standalone video platform or to connect with a larger screen. For example, the Endockscope is an attachment developed at the University of California Irvine that allows pairing between a standard analog cystoscope and an iPhone [7]. The quality is equivalent to that of a standard digital cystoscope [8]. Though, a formal adapter is not always necessary as Robinson et al. demonstrated the use of the smartphone camera light as a light source in the absence of an external power source [9].
Enhanced Cystoscopy
Enhanced cystoscopy adds an additional layer to the fundamental image generation and lighting of cystoscopy. Enhanced cystoscopy is performed using either narrow band imaging or fluorescent cystoscopy.
Narrow band imaging filters white light into two discrete bands, one blue at 415 nm wavelength and one green at 540 nm. Both bands are absorbed by hemoglobin. The shorter band penetrates the superficial mucosa and is absorbed by the superficial capillary vessels, giving a brown color. The longer band penetrates deeper into the bladder wall and is absorbed by deeper vasculature and gives a green color. Therefore, under the filtered light the superficial vessels will be dark brown and the deeper vessels appear green. Concentrated enhancement of vasculature would suggest a malignant growth. For example, flat lesions such as carcinoma in situ are very dense in vasculature but may have relatively normal appearing mucosa. Under the filter, the increased vasculature would be seen as a brown collection. Papillary lesions with deep vasculature would have a green plexus [10]. It has a role in the surveillance of non-muscle invasive bladder cancer as it has been shown to decrease recurrence in lower risk lesions [11, 12].
Fluorescent cystoscopy, also called photodynamic cystoscopy, involves the instillation of either 5-aminolaevulinic acid (ALA) or hexaminolevulinate (HAL) into the bladder. HAL and ALA are photosensitizers which result in preferential accumulation of protoporphyrins in rapidly proliferating cells such as malignant bladder tumors. They are subsequently converted to photoactive porphyrins, which emit a red fluorescence under blue light (360–450 nm). For example, the HAL solution is typically made up of 100 mg of HAL with 50 ml of diluent. It is allowed to dwell in the bladder for 1–3 h prior to performing cystoscopy with a specially designed cystoscope and processing system, such as the KARL STORZ D-Light C Photodynamic Diagnostic (PDD) system which enables both WLC and BLC (wavelength 360–450 nm) fluorescence cystoscopy. Under cystoscopic examination using this system the entire bladder is evaluated under both white and blue light. Under blue light, abnormalities are defined by the detection of red, homogeneous fluorescence [13]. In a phase III trial of patients with a history of non-muscle invasive bladder cancer, using a rigid cystoscope version of the system, about 20% of high grade non-muscle invasive were seen only with blue light [14]. Interestingly, in one subanalysis of 246 patients in the United States within the phase III trial for blue light fluorescent cystoscopy, patients did not report any increase in pain from the catheterization procedure required for instillation of HAL. Additionally, just over half the patients were willing to pay $100 or more for the perceived improvement in surveillance, while 30% prefered to pay no additional for the blue light [15].
The improved detection has made fluorescent cystoscopy part of the American Urologic Association/Society of Urologic Oncology guidelines. In patients with non-muscle invasive bladder cancer undergoing TURBT, the clinician should offer blue light. Additionally, blue light now has a role in patients who have normal cystoscopy but positive cytology as part of enhanced evaluation [12].
Disposable Cystoscopes
With concerns over infection control and cost increasing, multiple companies have worked towards the development of disposable and semi-disposable cystoscope and ureteroscope equipment. For endoscopy, the difficulty in sterilization and decontamination is in the flow channels and working sheaths. The CST-5000 is a flexible cystoscope manufactured by Cogentix (Orangeburg, NY) that contains a single scope with a disposable cover sheath. The sheath contains a flow and working channel. Theoretically, eliminating the need for scope downtime in decontamination will increase speed of analysis. However, in one randomized trial of an earlier version of the cystoscope in 100 patients head-to-head with the standard 16 fr cystoscope, there was marginal though statistically significant poorer optical quality, handling, and ease of insertion. The turnover time was reduced between 4 and 30 min and there was a similar cost per procedure with standard cystoscopes. Although, potentially significantly decreased repair costs due to the less complicated permanent mechanisms [16]. An alternative model is use specific cystoscopes, such as the Isiris by Coloplast (Humlebæk , Denmark). The Isiris has a built in stent grasper and is adequate for stent retrieval, though due to flow limitations associated with its design would not be adequate for routine cystoscopic surveillance and has no working channel for other instrumentation [17].
Future Technologies
To review, we have added light, better image generation, more portability, and increased image processing. Further development remains adding even more information to the options available. Potential future directions include improved means of data storage from endoscopy, such that the creation of 3D reconstruction of the bladder with white light cystoscopy [18]. Future directions also include the use of more off the shelf technology, therefore improving portability and decreasing cost. One of the most exciting future developments in our view may come from increasing computing power and machine learning allowing for improved image processing and interpretation beyond the capabilities of the surgeon’s eye. Such technologies may include optical coherence tomography (OCT). OCT allows for a non-invasive real time high resolution imaging of tissue in cross section to a depth of 2 mm through the analysis of the backscatter and absorption properties of near-infra-red light (890–1300 nm) It has been demonstrated in concept in bladder lesions and has been shown in other fields for a number of years [19, 20]. Further very early clinical trials have demonstrated that OCT is also very good at distinguishing Ta from T1 to T3 lesions with nearly 100% sensitivity and over 90% specificity [21]. A similar technology confocal laser endomicroscopy has been utilized in gastroenterology and is finding a place in urology [21].
Upper Urinary Tract: Ureteroscopy
Flexible Ureteroscopes
The field of ureteroscopy has grown immensely since the first flexible ureteroscope was described in 1964 [22]. Advancements in maneuverability, optics, and miniaturization, has allowed surgeons to access what was previously inaccessible. In 1971, Takayasu and colleagues published their use of a 2.5 mm flexible ureteroscope that was advanced into the ureter and kidney [23]. Although impressive in its own right, this scope only had 30° of passive deflection. Further improvements allowed the development of actively deflectable ureteroscopes with working channels that could maintain irrigation as well as instrumentation for treatment of upper urinary tract pathology [24]. Fuchs and Fuchs published one of the early large series of flexible ureteroscopy to treat stone disease in 208 patients utilizing a 10.4F flexible ureteroscope. Although this scope had a contemporary 3.7F working channel, the 160° of flexion was limited to one plane (70° in the second plane) [25]. Regardless, the technology was ripe for expansion.
Selection of currently available flexible ureteroscopes
Manufacturer | Model | Working channel | Tip size | Shaft size | Optics | Deflection (up°/down°) |
---|---|---|---|---|---|---|
Richard Wolf | Cobra vision | 3.6F and 2.4F | 5.2F | 9.9F | Digital | 270/270 |
Richard Wolf | Boa vision | 3.6F | 6.6F | 8.9F | Digital | 270/270 |
Karl Storz | Flex-xc | 3.6F | a | 8.5F | Digital | 270/270 |
Karl Storz | Flex-X2 | 3.6F | a | 7.5F | Fiberoptic | 270/270 |
Olympus | V2 | 3.6F | 8.4F | 8.6F | Digital | 285/285 |
Olympus | P6 | 3.6F | 4.9F | 7.95F | Fiberoptic | 270/270 |
Boston Scientific | Lithovue™ (single-use) | 3.6F | 7.7F | 9.5F | Digital | 270/270 |
Pusen | Uscope (single use) | 3.6F | 9.0F | 9.5F | Digital | 270/270 |

Karl Storz Flex-Xc digital flexible ureteroscope (©2018 courtesy of KARL STORZ SE & Co. KG, Tuttlingen, Germany)

Olympus P6 fiberoptic flexible ureteroscope (©2018 courtesy of Olympus Corporation, Tokyo, Japan)
Flexible ureteroscope deflection has improved significantly, allowing consistent access to lower pole calyces. Almost all scopes have deflection of 270° in both directions, while the Olympus V2 has slightly more at 285°. However, the digital flexible ureteroscopes, owing to their increased size, do have some slightly reduced maneuverability in practice [28].

Boston Scientific Lithovue™ single-use digital ureteroscope (©2018 courtesy of Boston Scientific, Marlborough, MA)
Semi-Rigid Ureteroscopes
Although Hugh Hampton Young is credited with the first rigid ureteroscopy in 1912 using a cystoscope in a child megaureter, it was not until almost 80 years later when semi-rigid ureteroscopy became commonplace. Dretler and Cho described using a 7.2F semi-rigid ureteroscope, more successfully in women than men, which allowed for broader management of ureteral pathology including stones and strictures [31]. Transition to fiberoptic imaging bundles allowed for larger working and irrigation channels while maintaining a similar size, as noted by Abdel-Razzak and Bagley in 1993 [32].

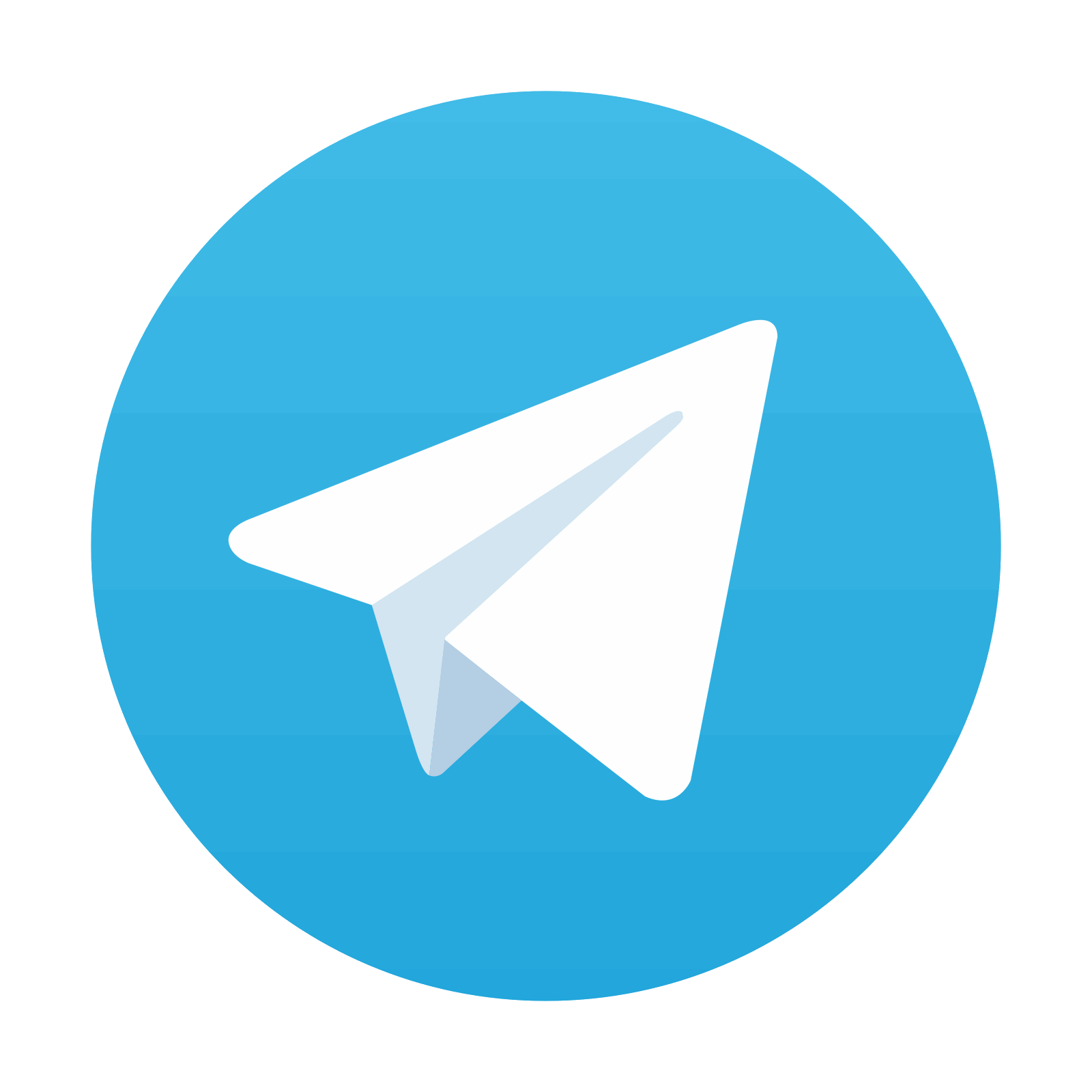
Stay updated, free articles. Join our Telegram channel

Full access? Get Clinical Tree
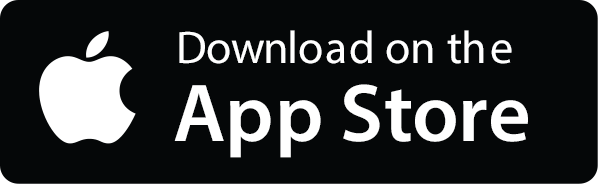
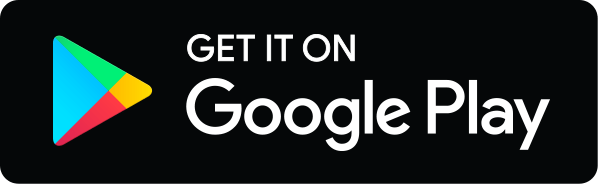