div class=”ChapterContextInformation”>
5. Ureteral Physiology and Pharmacology
Keywords
Ureteral peristalsisProstaglandinsUreteral obstructionUreteral stentsAlpha blockersUreteral Anatomy
The ureters are retroperitoneal structures that carry urine from the kidney to the bladder. They travel inferiorly from the renal pelvis at the ureteropelvic junction (UPJ), course anteriorly along psoas major, pass over the pelvic brim at the level of the common iliac artery bifurcation, and then run along the lateral pelvic sidewall to enter the urinary bladder. In adults they are typically 22–30 cm in length and 1.5–6 mm in diameter. Radiologically, the ureter is divided into three segments: proximal (UPJ to sacroiliac joint), middle (overlying sacrum), and distal (lower border of sacroiliac joint to bladder) [1].
The terminal ureter near the bladder is enveloped by a muscular layer called the Waldeyer sheath and then pierces the bladder. It travels 1.2–2.5 cm intramurally in adults before opened up into the bladder inferiorly and medially along the trigone as the ureteral orifice. Detrusor fibers coalesce with the Waldeyer sheath such that increased intravesical pressure prevents reflux of urine from the bladder to the ureter [1].
Blood supply to the ureter above the pelvic brim (proximally) arises medially from the renal artery, abdominal artery, gonadal artery, and common iliac artery. Below the pelvic brim (distally), the blood supply to arises laterally from the branches off the internal iliac artery [1].

Basic retroperitoneal anatomy with cross section of the ureter
Initiation of Peristalsis
Precise coordination of ureteral smooth muscle is required for downstream propagation of urine between the kidneys and the bladder. As with all smooth muscle, the contraction of the ureter is the result of depolarization of the cell membrane. In the ureteral smooth muscle cell, the resting membrane potential is −33 to −70 mV as a result of the distribution of potassium (K+) ions across the cell membrane. When stimulated, the membrane becomes less permeable to K+ and more permeable to calcium (Ca2+). The Ca2+ influx into the cell then activates calmodulin, which binds to myosin light chain kinase and leads to myosin phosphorylation. Phosphorylated myosin then migrates up actin filaments, resulting in smooth muscle contraction [4].

Autorhythmicity is first generated by atypical smooth muscle cells. These pacemaker signals are then modulated by surrounding interstitial cells of Cajal-like cells (ICC-like cells). Modulation occurs under the influence of multiple factors, including neuronal, prostaglandin, and hormonal stimulation. These modulated signals finally pass onto typical smooth muscle cells that result in a coordinated firing action, initiating ureteral peristalsis
Mediators that increase intracellular calcium cause ureteral smooth muscle contraction. Interactions with 1,4,5-triphosphate and diacylglycerol cause increased contraction, whereas mediation of G-protein coupled receptor complexes via cAMP and cGMP reduce contraction by lowering calcium levels [11].
Additional work demonstrates that peristalsis is initiated not by neurons but by smooth muscle itself, as experiments using tetrodoxin or autonomic nerve blockers fail to block ureteral contractions [12]. In addition, denervated transplant ureters have been shown to maintain contractility [13] and normal antegrade peristalsis has been shown to continue after reversal of the ureter in situ [14].
Modulation of Peristalsis
While initiation of ureteral contraction is to some degree independent from the nervous system, modulation of peristalsis relies heavily on the autonomic and sensory nervous system, as well as prostaglandins. Ureteral contraction can vary with regard to rate and contractility.
The autonomic nervous system influences ureteral contraction through both parasympathetic and sympathetic fibers. The parasympathetic nervous system acts on muscarinic receptors, with acetylcholine as its major neurotransmitter via postganglionic cholinergic fibers. The cholinergic innervation is especially rich in the distal and intravesical ureter [15]. Significant evidence indicates that the effect of such cholinergic stimulation varies by species, with decreased ureteral activity noted in dogs and increased activity noted in a pig model [16, 17]. However in humans, cholinergic agonists have demonstrated an excitatory effect on the ureter and renal pelvis, increasing both the frequency and force of contractions [18–20]. This is supported by excitatory effects on the ureter found with the use of anticholinesterases such as physostigmine and neostigmine [18, 21], along with some inhibitory effects of ureteral contraction noted with atropine, a competitive muscarinic antagonist [22].
The sympathetic nervous system affects ureteral function by modulating and mediating ureteral contraction. The ureter contains both excitatory alpha-adrenergic along with inhibitory beta-adrenergic receptors [19, 23]. There are four α1-adrenergic receptor subtypes (α1A to α1D), with the α1A subtype being the primary receptor subtype that participates in contraction of the human ureter [24–26]. It is understood that agents that activate these α1-adrenergic receptors, such as norepinephrine and phenylephrine, increase ureteral and renal pelvic contractile activity [18, 19, 23]. Furthermore, the highest density of α1-adrenergic receptors are found in the distal ureter, as evidenced by the greater contractile force seen in the distal versus proximal human ureter with the administration of phenylephrine, an alpha-adrenergic agonist [26]. Conversely, stimulation of the beta-adrenergic receptors causes ureteral and renal pelvic relaxation, as seen with the use of beta-adrenergic agonists such as isoproterenol and orciprenaline [27–29]. Further support of these findings was seen when norephinephrine, a primary alpha-adrenergic agonist with some beta-adrenergic agonistic activity, was found to increase the force of ureteral contraction. However, when administered with phentolamine, a potent alpha-adrenergic antagonist, norepinephrine was found to decrease the force of ureteral contractions. This reversal of action is likely due to the unopposed beta-agonistic effect of norepinephrine on the ureter given the alpha-blockade provided by phentolamine [23, 27].
Sensory nerves are able to modulate peristalsis via capsaicin-sensitive fibers. The sensory nerves found in the ureter are unmyelinated C fibers and poorly myelinated A-delta fibers [9]. Sensory afferent nerve fibers release several molecules that influence peristalsis. Among these are tachykinins and calcitonin gene-related-peptides (CGRP). Tachykinins are released in response to painful stimuli and cause smooth muscle contraction via G-protein coupled receptors, specifically the NK receptors in the ureter [30, 31]. Examples of tachykinins are neurokinins A, neurokinin K, and substance P [32]. Therefore, the presence of neurokinins and substance P are associated with increased contraction in the proximal and distal ureter [32, 33, 34]. Neurokinin A has been shown to be the most potent of the tachykinins, and treatment of ureters in vitro with a neurokinin antagonist decreased ureteral contraction by 80% [34]. Capsaicin-sensitive sensory nerves also contain and release CGRP in response to low levels of capsaicin, which inhibit electrical and contractile activity in the ureter [31]. CGRP cause ureteral relaxation by opening ATP-sensitive K+ channels, which cause membrane hyperpolarization and resultant blocking of the Ca2+ channels needed for generating a contraction [35, 36].
In addition to the nervous system, prostaglandins (PG) have been shown to play a role in ureteral contractility. PGs originate from arachadonic acid as a result of cyclooxygenase (COX) activity. COX exists in two isoforms: COX-1 and COX-2. The expression of COX-1 is relatively consistent, whereas COX-2 expression is heavily influenced by outside stimuli such as inflammation and obstruction. The effect of PG release varies between obstructed and unobstructed ureters. COX-2 expression is upregulated in obstructed ureters as compared to unobstructed ureters [37]. There are several subtypes of PG, and each has been studied independently regarding their effect on ureteral contraction. It has been shown in vitro that PGF2α, PGD2, and TXA2 increase ureteral contraction. PGE2 is unique in that it has been shown in pig and human models to have a condition-dependent effect on ureteral contractility, namely, inducing contractility in the obstructed ureter while promoting relaxation in the unobstructed ureter [38].
Physiologic Implications of Obstruction
Obstruction , whether from an intrinsic or extrinsic source, results in an increase in ureteral stretch due to the increase both in the volume of retained urine and intraluminal pressure. Afferent sensory neurons react to this stretch and contractility, eliciting a significant pain response [39]. The effect of obstruction on ureteral physiology is largely dependent on the duration of obstruction. Initially in obstruction, there is a transient increase in the amplitude and frequency of peristaltic contractions. Within a few hours after obstruction, intraluminal pressure reaches a peak and then declines to a level slightly higher than baseline [40]. This is largely attributable to changes in renal hemodynamics causing reduced renal blood flow, reduced glomerular filtration rate, and accordingly reduced intraluminal pressure [41].
Over time however, with persistent obstruction, ureteral diameter and length increases due to continued buildup of retained urine. The ureteral smooth muscle itself undergoes hypertrophy and increased contractile force, however due to the large increases in diameter, the ureter is unable to coapt its lumen to propel the urinary bolus forward [40]. Chronically obstructed ureters furthermore start to exhibit a reduction in the number of interstitial smooth muscle cells, resulting in a lack of peristaltic integration [42]. Abnormalities of innervation and an increase in collagen expression have also been identified in obstructed ureteral segments [43]. Furthermore, as illustrated in animal models, prolonged obstruction in excess of 6 weeks results in complete and irreversible loss of function in the obstructed renal unit [44].
The Effects of Ureteral Stenting
Ureteral stents provide drainage of the upper tracts in cases of obstruction. There have been multiple studies investigating flow patterns in the stented ureter. There had been conflicting prior reports of stents achieving drainage via larger luminal size with more fenestrations [45], while other in vitro studies showed drainage occurring around the stent versus through it [46]. Subsequent studies later demonstrated that fluid actually drains both through and around the stent, with similar flow patterns amongst all double pigtail stents regardless of specialty stent design or luminal size [47].
Ureteral stenting has been shown to impair short-term ureteral peristalsis in several animal models. In a porcine model of the stented ureter, normal peristalsis disappeared for days 1 and 2, and then became irregular after day 5 [48]. Evidence of ureteral dilation and ineffective peristalsis was seen to persist 3 weeks after stenting in pigs [49] and up to 16 weeks in dogs [50]. Drainage certainly is improved in obstructed ureters post stenting, however this is likely due to passive drainage secondary to hydrostatic pressure in the collecting system [51].
The histopathological changes to the ureter with stenting are significant. Stenting of normal rabbit ureters revealed mild hydroureteronephrosis with short-term stenting, progressing to moderate to severe hydroureteronephrosis with prolonged stenting over 3 weeks. Short-term stenting for less than 1 week did not show any harm, however prolonged stenting resulted in renal function deterioration in 2 of 18 renal units due to severe hydroureteronephrosis or infection. Histological examination revealed dilation of the entire collecting system and renal tubules, infiltration of the kidney and ureter with inflammatory cells, mucosal ulceration, and ureteral muscular hypertrophy [52]. To date there have been no physiologic studies to show the effects of stented ureter over the long term, therefore the timeline for reversal of these changes has not been elucidated.
Ureteral Pharmacology
The significant visceral pain brought about by ureteral obstruction has been largely attributed to the increase in associated ureteral contractility, spasm, and pressure. It has been shown that partial ureteral obstruction causes a 478% increase in the amplitude of ureteral contractions in a rat model, eliciting the severe associated colicky pain [39]. Several pharmacologic therapies therefore have been studied in an attempt to reduce the severity of these symptoms.
Opioids are commonly used to control the symptoms associated with ureteral obstruction. They work by activating mu-receptors and thus block the afferent pain pathways caused by ureteral obstruction. Interestingly, some studies have shown that narcotic analgesics increase both the tone and amplitude of ureteral contractions [18, 53]. Other studies however have failed to observe any type of effect on ureteral function [22, 54]. Overall, it appears that the role of opioids in the treatment of colic is largely dependent on their central nervous system actions and is not related to ureteral relaxation.
The main PGs (PGE1, PGE2, and PGF2α) are derived from arachidonic acid via COX-1 and COX-2. As mentioned previously, they have a variety of different biologic functions however for the most part promote ureteral contractility. Furthermore, COX-2 activity in particular has been shown to be upregulated in obstructed and inflamed ureters [55]. Agents such as nonsteroidal anti-inflammatory drugs (NSAIDs) have been successful in inhibiting ureteral contractility in multiple studies [56]. Both nonselective COX inhibitors (such as diclofenac and indomethacin) along with selective COX-2 inhibitors (such as celecoxib) have been shown to be equally efficacious in inhibiting contractions in both the pig and human ureter [57, 58]. Two randomized controlled trials assessed stone passage rates with diclofenac and indomethacin. Both trials demonstrated that patients did not experience improved stone passage rates or decreased time to passage. They did however require significantly less amount of narcotic analgesic requirement [59, 60]. Despite the clear effect on ureteral contractility, the clinical use of NSAIDs should be considered cautiously due to their effect on renal function. PGs promote renal vasodilation, therefore use of these COX inhibitors has the potential to cause renal insufficiency [17].
Phosphodiesterase (PDE) inhibitors block the degradation of cAMP and cGMP in smooth muscle, leading to smooth muscle relaxation by activation of protein kinase A and phosphorylation of myosin light chain kinase. The action of PDE inhibitors on the ureter causes a reduction in the frequency of peristaltic waves [61], and in vitro experiments have shown a reversal of tension along human ureteral smooth muscle, with vardenafil having the highest efficacy [62]. Furthermore, the presence of isoforms PDE-1, 4, and 5 were isolated in cytosolic solutions of human ureteral tissue [63], with PDE-4 being identified as having the greatest effect on ureteral relaxation [64]. Rolipram, a PDE-4 inhibitor, caused marked ureteral relaxation in a rabbit in vivo model without any of the deleterious hemodynamic effects seen with non-specific PDE-inhibitors, making this a promising target for further investigation [64]. In a recent meta-analysis, PDE-5 inhibitors were found to be superior to placebo in the rates of successful medical expulsive therapy for distal ureteral stones. There is also some evidence to suggest a reduction in the episodes of colic along with the need for analgesia, however further study is required to solidify this conclusion [61].
Calcium channel blockers inhibit the influx of extracellular calcium needed to generate an action potential and thereby limit the degree of smooth muscle contraction. They have therefore been studied extensively in the setting of obstructing ureteral calculi to minimize ureteral spasm and pain while encouraging spontaneous passage [65]. Calcium channel blockers, such as nifedipine, verapamil, and diltiazem, have been shown to inhibit ureteral contraction in guinea pigs and humans [66, 67]. Nifedipine and verapamil in particular were found to inhibit fast phasic contractions while preserving slow phasic contractions, thus preventing painful ureteral spasm while maintaining normal peristalsis [68]. With respect to stone passage, randomized controlled trials have shown efficacy when nifedipine is combined with corticosteroid therapy. One randomized double-blind trial of 86 patients comparing nifedipine and versus placebo (with methylprednisolone given to both) demonstrated an increased stone expulsion rate (86% vs. 65%) and less mean time to passage (11.2 days vs. 16.5 days) in the nifedipine group versus placebo group, respectively [69]. Another randomized trial compared a control group treated with analgesia alone versus a nifedipine and prednisone treatment arm. The treatment arm similarly was found to have a higher passage rate than the control arm (86% vs. 54%) after 7 days of treatment [70]. In a more recent series, 96 patients with distal ureteral stones received either deflazacort and nifedipine or conservative management. Stone expulsion rate in the nifedipine group was 79% compared to 35% in the conservatively managed group. Mean time to stone passage was 7 days in the nifedipine group versus 20 days in the conservative group [71]. Despite this evidence of increased stone passage rate, there is no evidence that calcium channel blockers contribute to pain control from obstruction. One study evaluated nifedipine versus placebo in 30 patients with renal colic and found no significant difference in pain control [72]. With respect to steroid therapy, they were found to provide some benefit as expulsive therapy when combined with other agents but not alone [73].
Alpha adrenergic antagonists, or alpha blockers, work by blocking adrenergic activation of smooth muscle cells, resulting in a reduction in both the frequency and amplitude of contractions. As described earlier, α1-adrenergic receptors are found throughout the ureter with the highest concentration being in the distal ureter. Therefore, in the setting of ureteral calculi, alpha blockers can aid in facilitating spontaneous passage. Alpha blockers can be broadly grouped as either selective or nonselective, depending on their affinity especially for α1A and α1D receptors, which are abundant in the ureter [74]. Both selective (tamsulosin) and nonselective (terazosin, doxazosin) alpha blockers have been shown to be equally efficacious in reducing ureteral spasm and improving stone passage rates [75]. There is evidence to suggest analgesic benefit of alpha blockers, as seen with the use of tamsulosin in patients post shockwave lithotripsy and particularly with the development of steinstrasse [76, 77].
Numerous randomized studies have examined tamsulosin compared to either nifedipine or placebo for expulsive therapy. In these studies, the stone passage rate was 97–100% compared to 64–70% in the placebo groups [78, 79]. Tamsulosin offered a 20% increase in passage rate when compared to nifedipine [80]. In 2006, Hollingsworth and colleagues published a meta-analysis of nine randomized controlled trials investigating the use of nifedipine and alpha blockers. Compared with the control groups, patients treated with nifedipine or alpha blockers had an overall 65% greater likelihood of stone passage with an absolute risk reduction of 31% [73]. A subsequent meta-analysis from the combined AUA/EUA ureteral stone guidelines demonstrated a statistically significant absolute increase of 29% in stone passage rate of tamsulosin when compared to controls. Nifedipine had an absolute increase of 9% when compared to controls, which was deemed to not be statistically significant [81]. A more recent meta-analysis of 47 randomized controlled trials demonstrated higher and faster expulsion rates when the alpha blocker and nifedpine groups were pooled and compared to controls. Lower analgesic requirements, fewer colic episodes, and fewer hospitalizations were also observed with the treatment groups [82]. A Cochrane review was also conducted recently of 67 studies with over 10,000 participants comparing alpha blockers to placebo. Stone clearance in the alpha blocker group was significantly higher (RR 1.45, 95% CI 1.36–1.55), and remained high still (RR 1.16, 95% CI 1.07–1.25) after a subset of better quality subset of placebo controlled trials was extracted from the main body. Similar to previous analyses, the alpha blocker groups experienced shorter expulsive times by 2–4 days, used less analgesia, and required fewer hospitalizations. The need for surgery was found to be similar between the two groups. Subgroup analyses further suggested that alpha blockers could be less effective for stones <5 mm than for larger stones >5 mm [83].
A multicenter, randomized, placebo-controlled trial (referred to as the SUSPEND trial) recently published some contradictory findings. 1167 patients at over 24 centers in the UK with a single ureteral stone ≤10 mm in size were randomly assigned to placebo, nifedipine, or tamsulosin groups. Spontaneous stone passage within 4 weeks, without the need for additional interventions, was defined as the primary outcome. The percent of patients who did not require further intervention by 4 weeks was 80% in the placebo group, 81% in the tamsulosin group, and 80% in the nifedipine group. No difference was noted between the active treatment and placebo groups, or between the tamsulosin and nifedipine groups. No differences were noted in secondary outcomes of pain score or time to stone passage. The authors concluded that tamsulosin and nifedipine were not effective at decreasing the need for further treatment to achieve stone clearance in 4 weeks [84]. The quality of the trial was considered to be very good as it was well-powered, had centralized randomization, and was multicenter. A notable criticism of the trial was that the rates of stone clearance for stones >5 mm was slightly more pronounced for tamsulosin, however because the majority of the patients assessed (24.8%) had stones >5 mm. The trial may have therefore been underpowered to detect the utility of medical expulsive therapy for stones >5 mm, and that the potential benefits of medical therapy may have been diluted by inclusion of smaller stones [85]. Other criticisms included lower response rates for questionnaires to evaluate the secondary outcomes and well has lack of monitoring for medication adherence [86]. Overall however, this trial does challenge the current dogma of medical expulsive therapy. Whether future trials yield similar findings will remain to be seen.
Other pharmacologic agents have been contenders for further study. Neurokinin receptor antagonists have been studied in vitro as alternative agents to modulate ureteral contractility. The three neurokinin receptors, NK-1, NK-2, and NK-3 have affinity for substance P, neurokinin A, and neurokinin B, respectively [87]. Blockage of these receptors prevents phospholipase C synthesis and ultimately calcium influx into the smooth muscle cell. The result is ureteral relaxation. NK-2 is the predominant receptor in the human ureter, and its inhibition in vitro prevents ureteral contractility [87]. NK-2 blockade has yet to be studied clinically, but if safe may decrease obstruction-related pain and increase stone passage rates.
Nitric oxide (NO) is a major inhibitory neurotransmitter in the ureter. Based on axons that stain positive for nitric oxide synthase in the human ureter, it has been suggested that nitric oxide may play a role in ureteral relaxation [88]. In vitro, it has been shown that NO inhibits ureteral contractility in rats [89]. Interestingly, NO seems to play a specific role at the ureterovesical junction, where it has been postulated to regulate the valve-like effect in this area [90].
The effect of various substances on the ureter
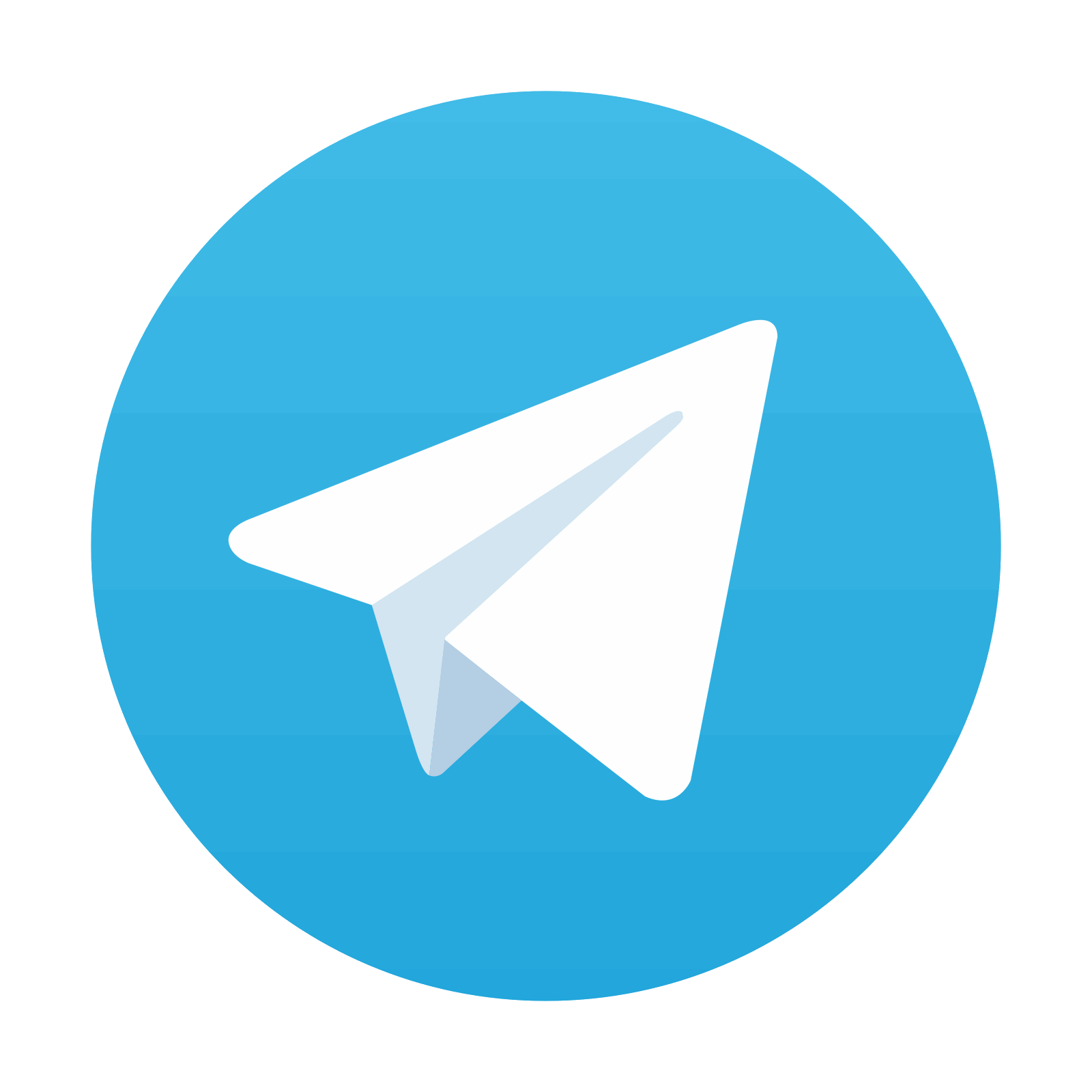
Stay updated, free articles. Join our Telegram channel

Full access? Get Clinical Tree
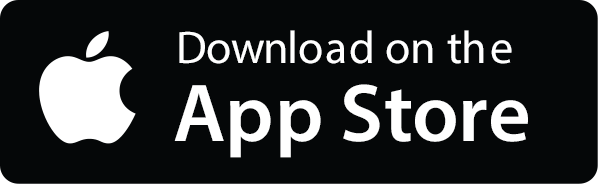
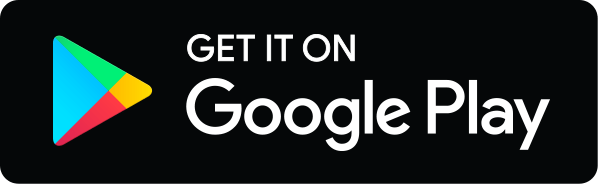
