Fig. 1
Model of T-lymphocyte activation and sites of action for immunosuppressive agents (Reprinted with expressed permission from Russell H. Wiesner) (Wiesner and Fung 2011)
In the direct pathway, the hepatic allograft and its surrounding tissue will express dendritic cells; these are professional antigen-presenting cells (APC). MHC molecules are expressed on APCs, which are recognized by host T lymphocytes. The indirect pathway involves a similar process; however, in this pathway, host T lymphocytes recognize processed donor alloantigen peptides expressed on host APCs and MHC molecules. There is evidence that both of these pathways occur as part of the immunologic response in the liver transplant recipient (Martinez and Rosen 2005). In the early posttransplant period, it is likely the direct pathway predominates and is a major factor in acute rejection. This stage can be aborted by antilymphocyte antibodies.
In contrast, the amount of T lymphocytes responding in the indirect pathway is low; compared to the direct pathway, they make up only 5–10 % of the total alloresponse (Valujskikh et al. 2001; Heeger and Dinavahi 2012). Despite this, indirectly primed T lymphocytes are integral to the rejection process and play an important role in chronic allograft dysfunction (Heeger and Dinavahi 2012).
Lymphocyte Activation
Signal two is lymphocyte activation, also known as costimulation, a process in which ligands on the APC cell bind to T-lymphocyte receptors. CD80 and CD86 markers on dendritic cells engage CD28 receptors on T lymphocytes (T-lymphocyte receptor, TCR); the receptor complex is then internalized and activates signal transduction pathways (Wiesner and Fung 2011). The TCR complex binds immunophilin; this stimulates calcineurin which activates nuclear factor of T-lymphocyte activation (NFAT). NFAT then translocates to the cell nucleus and signals IL-2 transcription (Fig. 1). Two immunosuppressive drugs target immunophilin, and cyclophilin and FK-binding protein (FKBP) are targets of cyclosporine and tacrolimus.
Clonal Expansion
Signal three is clonal expansion; transduction pathways from signal two activate numerous molecules, including IL-2 and other cytokines. Newly synthesized IL-2 is produced by T lymphocytes and binds to IL-2 receptors on the T-lymphocyte surface in a paracrine and autocrine fashion. This stimulates the downstream pathway of the mammalian target of rapamycin (mTOR) which is the trigger for cell proliferation (Fig. 1) (Martinez and Rosen 2005). Immunosuppressive drugs target multiple steps at this process, both basiliximab and daclizumab are monoclonal antibodies against the IL-2 receptor, and sirolimus and everolimus are mTOR inhibitors that block cell proliferation.
Graft Inflammation
The last step in the immunological rejection response is graft inflammation. Activated T lymphocytes precipitate differentiation in their ability to mediate effector functions (i.e., secrete cytokines and kill) and by modifying an array of cell surface molecules, including L-selectin (lymph node homing receptor) and chemokine receptors. This allows cells to migrate from lymphoid organs and circulate throughout the periphery. Activated T lymphocytes express a multitude of chemokine receptors which attract various immune cells to the site of inflammation, the allograft. The result is an inflammatory milieu with toxic and vasoactive mediators. Effector T lymphocytes reencounter their specific alloantigens on the graft and initiate effector mechanisms leading to cytolysis (Heeger and Dinavahi 2012).
Phases of Immunosuppression
Liver transplant immunosuppression can be classified into two distinct phases: induction and maintenance. Induction immunosuppression usually refers to a period of intense immunosuppression given in the first 30 days after transplant when the risk of organ rejection is highest (Kirk 2006). Maintenance immunosuppression refers to the immunosuppressive regimen an organ transplant recipient is continued on after the initial 30 days; in liver transplant recipients, there is usually a decrease in the number of drugs and dosages needed to prevent rejection (Wiesner and Fung 2011). With the exception of autoimmune liver disease and hepatitis C virus, most LTRs may receive similar regimens with steroid induction with or without adjunctive antibody therapy, followed by two maintenance agents and eventual steroid withdrawal (Fig. 2).


Fig. 2
Example of induction and maintenance immunosuppression regimens in liver transplant recipients
Induction
Induction regimens in the USA have typically referred to the use of antilymphocyte-depleting agents; this is only used in a small percentage of liver transplant recipients, but in the past decade, the use of induction has increased. In 2003, the overall use of induction immunosuppression regimens was approximately 20 %; in 2013, nearly 30 % of liver transplant recipients were given induction therapy (Fig. 3). More commonly peri- and postoperatively, these patients are started on a common drug regimen that includes high-dose corticosteroids with a rapid taper, a calcineurin inhibitor, and an antimetabolite agent such as mycophenolate (Wiesner and Fung 2011).
The role of antilymphocyte-depleting agents has been clearly defined in other organ transplants such as kidney transplant; however, in liver transplant recipients, the data is not as clear. Traditionally, induction therapy has been given as an adjunct to conventional immunosuppression to prevent the risk of rejection, to delay or minimize calcineurin exposure to prevent renal dysfunction, or to facilitate steroid avoidance and decrease the risk of HCV recurrence.
Induction therapy as an adjunct to conventional immunosuppression refers to adding a lymphocyte-depleting or a nonlymphocyte-depleting agent as an adjunct to a conventional calcineurin-based regimen. The most commonly used lymphocyte-depleting agent in the USA is antithymocyte globulin (Thymoglobulin); this is a polyclonal rabbit, derived antibody preparation which targets multiple epitopes on T lymphocytes. The result is nonspecific T-lymphocyte depletion with a dose-dependent lymphopenia that suppresses T lymphocytes for up to 90 days post administration (Boillot et al. 2009). (A more in–depth explanation of pharmacology and monitoring will be explained in the section “ Immunosuppressive Drugs ”.)
Antithymocyte globulin induction to prevent rejection in liver transplantation has been evaluated in two small, randomized controlled trials. Boillot et al. evaluated antithymocyte globulin induction added to conventional maintenance therapy with tacrolimus/mycophenolate/prednisone vs. conventional therapy without induction, in 93 patients (Boillot et al. 2009). The primary outcome of rejection at 5 years was not different between groups. Bogetti et al. evaluated antithymocyte globulin induction added to maintenance of tacrolimus/prednisone in 22 patients (Bogetti et al. 2005). Compared to tacrolimus/prednisone alone, induction did not improve rejection rates at 3 months. Neither study showed differences in safety outcomes, including infection and malignancy. This limited data suggests that adding antithymocyte globulin induction therapy does not reduce rejection risk in liver transplant recipients.
Alemtuzumab (Campath) is a monoclonal antibody that targets the CD52 T-lymphocyte receptor that depletes mature lymphocytes for up to 1 year post administration. Experience using alemtuzumab in liver transplantation is limited. An NIH-sponsored study showed that alemtuzumab induction permits weaning to spaced tacrolimus monotherapy with comparable efficacy outcomes as conventional immunosuppression in de novo liver transplant recipients (Marcos et al. 2004). However, despite comparable outcomes between groups in the subgroup with hepatitis C virus, a large number of those exposed to alemtuzumab experienced complications or graft loss related to hepatitis C virus reactivation. Similar trials evaluating alemtuzumab induction followed by tacrolimus monotherapy in liver transplant yielded similar efficacy results, but excluded patients with hepatitis C virus (Tzakis et al. 2004; Tryphonopoulos et al. 2005). A recent retrospective case-controlled study of 140 patients without hepatitis C virus found that alemtuzumab followed by tacrolimus monotherapy, compared to conventional therapy, was associated with comparable efficacy outcomes, less hypertension and fewer cases of rejection, but a 43.6 % relative risk increase for infections, particularly viral infections (Levitsky et al. 2011). Overall, while alemtuzumab appears to permit less intense maintenance immunosuppression in liver transplantation, its increased risk of infection and hepatitis C reactivation remains concerning.
The lymphocyte-nondepleting agents, basiliximab (Simulect) and daclizumab (Zenapax), selectively target the activated T-lymphocyte IL-2 receptor, CD25, which stops downstream signaling of T-lymphocyte proliferation and activation, and effects are sustained for 1–2 months post administration. Several studies have evaluated interleukin-2 receptor antagonists (IL2RAs) as induction agents to prevent acute rejection in liver transplantation. A meta-analysis including 12 randomized controlled trials and 3,251 patients showed that IL2RA induction was associated with significant reduction in rejection at 1 year (23 % vs. 28 %) (Wang et al. 2010). The difference was significant for the subgroup of eight studies of daclizumab, but not for the four studies of basiliximab. Regardless of IL2RA, the overall improvement in rejection rates did not translate to an improvement in graft or patient survival. A recent Cochrane review comparing any antibody induction therapy to no induction found induction to be associated with statistically significantly less acute rejection (RR 0.85, CI 0.75–0.96), but nonsignificant differences in death or graft loss (Penninga et al. 2012).
Induction therapy has been used to facilitate calcineurin inhibitor minimization. It is known that calcineurin inhibitors cause renal dysfunction. Studies have shown that there is an 8 % incidence of chronic renal failure at 1 year post-OLT and 18 % at 5 years posttransplant. Also, an elevated creatinine 1 month posttransplant carries an increased risk of developing chronic renal failure (Gonwa et al. 2001). The presence of renal dysfunction is associated with significant morbidity and mortality after liver transplant.
The use of antithymocyte globulin induction to delay the initiation of calcineurin inhibitors has been evaluated in two retrospective studies. Tchervenkov et al. showed that antithymocyte globulin induction and delayed CNI introduction were associated with a modest, yet significantly improved, serum creatinine at 6 months (1.39 vs. 1.56 mg/dL) (Tchervenkov et al. 2004). Similarly, Soliman et al. showed modest improvements in serum creatinine and estimated glomerular filtration rates at 1 year (1.26 vs. 1.37 mg/dL and 81 vs. 75 mL/min) with antithymocyte globulin induction (Soliman et al. 2007). Both studies found reduced rejection rates with antithymocyte globulin induction, suggesting that antithymocyte globulin may permit the safe delay of CNI introduction in an attempt to improve short- to midterm renal function.
The use of IL2RAs to delay CNI has also been evaluated. The ReSpECT study was a prospective, randomized controlled trial comparing three arms: daclizumab with delayed reduced-dose tacrolimus/mycophenolate/prednisone, delayed reduced-dose tacrolimus/mycophenolate/prednisone, or standard-dose tacrolimus/prednisone (n = 517) (Neuberger et al. 2009). At 26 weeks, the estimated GFR was significantly improved in the group that received daclizumab (difference of 14.5 mL/min) compared to standard-dose tacrolimus, without differences in other efficacy or safety endpoints. Furthermore, a meta-analysis subgroup including six randomized controlled trials, with durations ranging 3–24 months, showed that the addition of IL2RAs to delayed or reduced CNIs was associated with improved renal function (estimated GFR difference 6.29 mL/min, 3 studies, n = 641) and lower rates of renal dysfunction (RR 0.46; CI 0.27–0.78, 5 studies, n = 778) (Goralczyk et al. 2011). A recent Cochrane review showed that any antibody induction was associated with higher serum creatinine (0.04 mg/dL; CI 0.003–0.08 mg/dL), although GFR was not significantly affected (Penninga et al. 2012). These data suggest that IL2RAs can be used to safely delay the initiation of CNIs, and this strategy is associated with improved short- to midterm renal function.
Last, induction regimens have been employed as a strategy to facilitate steroid avoidance. Along with the numerous metabolic effects of steroids, they have been associated with recurrence of hepatitis C, a major cause of liver transplantation and graft failure. Antithymocyte globulin and IL2RAs have been successful in steroid-free induction with no difference in acute cellular rejection; however, a difference in hepatitis C recurrence or adverse metabolic effects of steroid use has not been shown (Eason et al. 2001; Turner and Knechtle 2013). The use of alemtuzumab as an induction agent to minimize steroid use is not recommended because it has been associated with an increased incidence of hepatitis C recurrence (Turner and Knechtle 2013).
Maintenance Immunosuppression
Maintenance immunosuppression typically refers to the immunosuppressive regimen a liver transplant recipient (LTR) is started on after the first 30 days. However, one should think of a maintenance regimen in the immediate postoperative state. Early initiation of CNI regimens has shown to prevent acute rejection and prolong graft survival. The addition of antimetabolite agents confers additional immunosuppression while augmenting the long-term side effects of CNIs. The clinician must also take into account a recipient’s underlying liver disease. For instance, LTRs transplanted for autoimmune hepatitis will require higher doses of immunosuppression, often with the complement of low-dose steroids indefinitely. In contrast, patients transplanted for alcoholic cirrhosis and steatohepatitis will require lower doses of immunosuppression and can often be maintained low-dose dual-drug regimens or monotherapy. In rare instances, LTRs can maintain operational tolerance, whereas they have good graft function off all immunosuppression. Figure 3 shows the current immunosuppressive regimens in the USA. An in-depth review of the available immunosuppressive medications and their use in maintenance of the liver transplant recipient, as well as therapeutic monitoring and short- and long-term side effects, will be explored in the following section on “Immunosuppressive Drugs.”
Immunosuppressive Drugs
Corticosteroids
Given the abundance of glucocorticoid receptors throughout the body, corticosteroids have numerous pharmacologic actions. The most important immunosuppressive mechanism of corticosteroids occurs via steroid-receptor complex binding to DNA sequences (glucocorticoid response elements, GRE), which subsequently inhibit transcription of cytokine-encoding genes. Through inhibition of nuclear translocation of nuclear factor-κB (NF-κB), the expression of numerous cytokines, including IL-1, IL-2, IL-3, IL-6, TNF-α, and IFN-γ a, is inhibited.
Adverse effects of corticosteroids include short-term and long-term effects. Short-term adverse effects include hyperglycemia, hypertension, gastrointestinal discomfort, psychiatric disturbances, insomnia, increased appetite, and transient leukocytosis. Long-term adverse effects include infection, diabetes, hypertension, dyslipidemia, weight gain, Cushing’s syndrome, insomnia, depression, osteopenia/osteoporosis, glaucoma/cataracts, and cosmetic changes to skin and hair. A comparison of the adverse effects of maintenance immunosuppressants, including corticosteroids, is shown in Table 1.
Table 1
Adverse drug reactions of maintenance immunosuppressive agents
Steroids | TAC | CSA | MPA | AZA | mTORi | Belatacept | |
---|---|---|---|---|---|---|---|
Nephrotoxicity | ++ | ++ | |||||
Proteinuria | ++ | ||||||
Infection | + | + | + | + | + | + | + |
Malignancy | + | + | + | + | + | ||
Diabetes | ++ | ++ | + | + | |||
Dyslipidemias | ++ | + | ++ | ++ | |||
Hypertension | ++ | + | ++ | ||||
Weight gain | ++ | ||||||
Osteopenia | ++ | ||||||
Bone marrow suppression | ++ | ++ | + | ||||
Impaired wound healing | + | ++ | |||||
GI disturbances | + | + | ++ | + | + | ||
Cosmetic changes | + | + | + | + | |||
Neurotoxicity | + | ||||||
Glaucoma/cataracts | + | ||||||
Infusion reactions | + | ||||||
PTLD | + | + | + | + | + | ++ |
Corticosteroids have been the cornerstone of immunosuppression since the advent of liver transplantation. They are almost universally administered preoperatively. Despite its antirejection benefit, numerous studies have been performed to evaluate the impact of steroid minimization on reducing their numerous adverse effects. A meta-analysis of 21 trials, including 2,590 patients, demonstrated that steroid-free maintenance regimens were associated with similar rejection rates and patient and graft survival, when compared to conventional steroid-based immunosuppression (Sgourakis et al. 2009). Overall, steroid minimization was associated with a more favorable safety profile, including reduced posttransplant diabetes, CMV infection, and serum cholesterol. Of note, rejection rates were lower in patients that received antibody induction in place of steroid maintenance; however, protocols that employed steroid withdrawal without replacement favored maintenance steroids to reduce rejection. In sum, steroid minimization is safe in liver transplantation, especially when replaced with antibody induction.
The role of steroids in liver recipients with hepatitis C virus (HCV) remains controversial. Data is conflicting on whether steroid-free regimens, early steroid withdrawal, or late steroid withdrawal is best for HCV recurrence rates. Humar et al. showed that when steroids were withdrawn on postoperative day 6, HCV recurrence rates were 30 % lower at 1 year than when compared to conventional immunosuppression with steroid withdrawal at 6 months (Humar et al. 2007). The THOSIN study showed that a steroid-free regimen was associated with fewer liver biopsies with grade 4 portal inflammation or grade 3 or 4 fibrosis through 2 years, when compared to a regimen which withdrew steroids at 90 days (Lladó et al. 2008). Conversely, Vivarelli et al. compared steroid withdrawal at 3 months vs. slow tapering over 24 months (Vivarelli et al. 2007). In this trial, slow tapering of steroids was associated with a lower incidence of advanced fibrosis and improved advanced fibrosis-free survival. Surprisingly, in a large randomized trial enrolling 295 patients, the HCV-3 study found no significant difference in HCV outcomes with the steroid-free regimen when compared to the conventional therapies (Klintmalm et al. 2011). Despite the controversial findings of these studies, the bulk of the data, including a meta-analysis subgroup of a 14 trials, supports safe and beneficial steroid withdrawal in liver transplant recipients with HCV (Sgourakis et al. 2009).
Calcineurin Inhibitors
The calcineurin inhibitors, cyclosporine (CSA) (Neoral, Sandimmune, Gengraf) and tacrolimus (TAC) (Hecoria, Prograf, Astagraf XL, common name: FK506), bind to intracellular receptor proteins, cyclophilin and FK-binding protein (FKBP), respectively. The resulting CNI-receptor complex binds to calcineurin, a protein phosphatase responsible for the dephosphorylation and translocation of nuclear factor of activated T lymphocytes (NFAT). NFAT is a gene transcription factor integral to the expression of cytokines. Specifically, IL-2 expression is suppressed and T-lymphocyte activation is inhibited.
Cyclosporine is available in an intravenous formulation and two different oral formulations. The bioavailability of conventional CSA is more dependent on food intake, gastrointestinal motility, and bile for absorption than the CSA microemulsion [modifed] dosage form. Tacrolimus is available in an intravenous formulation, as well as an immediate release and extended release oral capsules. Intravenous formulations of CNI can be given in a 1:3 dosage ratio to oral formulations. Pharmacokinetic similarities of the CNI include their variable bioavailability, high protein binding, and metabolism by gastrointestinal and hepatic P-glycoprotein (PGP) and CYP3A4/5 isoenzymes. The elimination half-lives (t½) of CNI are similar, with CSA t½ ~8 h and TAC t½ ~8–12 h.
The most serious adverse effect of CNI is their dose- and duration-dependent nephrotoxicity. Acutely, CNIs lead to vasoconstriction of afferent arterioles mediated by an increase in vasoconstrictors (endothelin and thromboxane) and reduction of vasodilators (prostacyclin, prostaglandin E2, and nitric oxide) (Naesens et al. 2009). Chronically, irreversible tubular atrophy, interstitial fibrosis, arteriolar hyalinosis, and glomerulosclerosis may be observed on biopsy. In one series, more than 25 % of patients show histologic evidence of CNI-induced nephrotoxicity (CIN) after 1 year on therapy, and CIN was almost universal after 10 years (Nankivell et al. 2003). The incidence of chronic renal failure has been reported at 22 % 5 years after liver transplant (Sharma et al. 2009). Additionally, due to their vascular effects, CNIs are associated with systemic hypertension, with a higher incidence reported with CSA than TAC. Electrolyte abnormalities such as hyperkalemia, hypomagnesemia, hypocalcemia, and hyperuricemia are also common. Thrombotic microangiopathy (TMA) resembling thrombotic thrombocytopenic purpura (TTP) has been reported with CNI use.
Nonrenal adverse effects of CNI include diabetes, dyslipidemia, neurotoxicity, and cosmetic effects. Both CNIs are toxic to pancreatic islet cells and may lead to posttransplant diabetes mellitus (PTDM), although this is observed in a higher frequency with TAC than CSA. Dyslipidemia is more often associated with CSA. Neurotoxic effects occur more frequently with TAC and range from headache and tremors to seizures and reversible posterior leukoencephalopathy syndrome (RPLS). Cosmetic effects of CSA include gingival hypertrophy and hirsutism, whereas TAC is associated with hair loss and alopecia. Both CNIs are associated with an increased risk of infection and malignancies including posttransplant lymphoproliferative disorder (PTLD).
Given the wide inter-patient and intra-patient variability of CNI, therapeutic drug monitoring is integral to their safe and effective use. Trough (C0) levels of TAC are strongly correlated with area under the curve (AUC0–12) and may be used to assess treatment (Schiff et al. 2007). Unfortunately, CSA C0 levels are poorly correlated to AUC0–12, and pharmacokinetic data suggests that monitoring abbreviated AUC0–4 or C2 levels (i.e., concentration 2 h post-dose) may be preferable (Keown et al. 1996; Cantarovich et al. 1998). Despite this, owing to the logistic difficulty of obtaining AUC or C2 levels, coupled with a lack of data to demonstrate clinical superiority of C2 vs. C0 levels, C0 level monitoring remains the most commonly employed method in practice. Target levels of CNI are not universally accepted, but should take into consideration time from transplant, cause of liver disease, infection risk, and concomitant immunosuppression. Earlier studies utilized high initial target levels for CSA (e.g., C0 250–400 ng/mL); however, more modern studies utilize more modest targets (e.g., C0 150–250 ng/mL), with later targets as low as 75–100 ng/mL. Likewise, initial TAC targets in earlier studies were more aggressive (e.g., C0 10–20 ng/mL) than more modern targets (e.g., 7–12 ng/mL), with later targets as low as 4–6 ng/mL.
Cyclosporine
Prior to the approval of cyclosporine in 1983, 1-year survival after a liver transplant was approximately 30 % under immunosuppression with azathioprine and corticosteroids (Starzl et al. 1974). The earliest reports of CSA use, while small in size and duration, showed survival rates greater than 70 % in 1 year when used in conjunction with steroids (Starzl et al. 1981). Longer-term data supported these findings and led to the more than decade-long “cyclosporine” era of liver transplantation. Today, cyclosporine remains a reasonable alternative for patients intolerable of tacrolimus.
Tacrolimus
Since its FDA approval in 1994, tacrolimus eventually replaced cyclosporine as the first-line CNI in liver transplantation. The registry study was an open-label randomized trial of 478 de novo liver recipients comparing TAC to CSA, with concomitant azathioprine and corticosteroids. At 1 year, patient and graft survival rates were comparable, but a 10 % relative risk reduction in rejection, and more profound reductions in steroid-resistant and refractory rejections, was observed with TAC (US Multicenter FK506 Liver Study Group 1994). These results were echoed by the comparable European registry study of 545 patients, which found an 18 % relative risk reduction in rejection at 1 year (European FK506 Multicentre Liver Study Group 1994). The TMC study was an open-label randomized trial in 606 de novo liver recipients, comparing TAC-based to CSA-based regimens. The primary composite endpoint of transplantation, death, or refractory rejection occurred less frequently with TAC vs. CSA at 1 year (RR 0.63, CI 0.48–0.84) and 3 years (RR 0.75, CI 0.6–0.95) (O’Grady et al. 2002; O’Grady et al. 2007). Furthermore, a meta-analysis of 16 trials (n = 3,813) found that TAC was associated with significantly more favorable outcomes with regard to patient survival, graft survival, rejection, and steroid-resistant rejection, albeit with a higher risk for diabetes (Haddad et al. 2006).
Antimetabolites
Mycophenolic Acid
Mycophenolic acid (MPA) (CellCept, Myfortic) is a reversible inhibitor of inosine monophosphate dehydrogenase (IMPDH), a critical enzyme in de novo purine synthesis. It is available as mycophenolate mofetil (MMF) which undergoes rapid hydrolysis to MPA and enteric-coated mycophenolate sodium (EC-MPS) which is equivalent to active MPA. Peak MPA levels are observed 0.9–1.3 and 2.0–2.5 h after MMF and EC-MPS administration, respectively. Food reduces absorption of MPA and is best taken on an empty stomach. Mycophenolate undergoes hepatic glucuronidation to inactive mycophenolic acid glucuronide (MPAG), a portion of which is converted back to MPA and reabsorbed, leading to second peaks in the concentration-time curve approximately 5–6 h after administration. MPA is excreted renally; however, dose adjustment in renal impairment is not necessary.
The main dose-limiting toxicities of MPA are gastrointestinal in nature and include nausea, vomiting, dyspepsia, and diarrhea. Leukopenia, anemia, and thrombocytopenia are also frequently observed and may require dose reduction or interruption. Importantly, MPA is associated with congenital malformations when taken during pregnancy. In patients electing to become pregnant, MPA should be withheld at least 6 weeks prior to conception. All pregnancies occurring in patients on or previously exposed to MPA should be reported to the Mycophenolate Pregnancy Registry.
Therapeutic drug monitoring of MPA is possible, though not commonly utilized. Studies of kidney recipients in the early posttransplant period have shown that calculating the area under the curve (AUC0–12) predicts the risk of rejection and toxicity (van Gelder et al. 1999). Trough (C0) levels are poorly correlated with AUC and do not accurately predict rejection or toxicity (Mardigyan et al. 2005). A target AUC0–12 of 30–60 mg h/L has been suggested to prevent rejection; however, the logistic difficulty in obtaining multiple accurate levels and its paucity of supporting data have deterred its widespread use (van Gelder et al. 2006).
Since its approval in 2000, mycophenolate has largely replaced azathioprine. The superiority of MMF over AZA as an adjunctive agent was determined by a randomized, double-blind trial of 565 patients (Wiesner et al. 2001). In part of combination therapy with CSA and steroids, 6-month acute rejection rates in the MMF arm were 38.5 % compared to 47.7 % in the AZA arm. Patient survival, graft survival, and adverse events were comparable between groups. Of note, the MMF dose studied (3 g/day) is higher than what is typically employed in clinical practice. A smaller randomized trial of 63 patients found lower rejection rates with MMF 2 g/day compared to AZA, though the study may have been too small to detect a significant difference (Fischer et al. 2000; Sterneck et al. 2000).
The use of enteric-coated mycophenolate sodium in liver recipients has not been compared to mycophenolate mofetil in a randomized controlled trial; however, several small open-label studies have shown comparable outcomes after conversion for gastrointestinal adverse effects (Cantisani et al. 2006; Dumortier et al. 2006). Doses of EC-MPS 720 mg are considered therapeutically equivalent to MMF 1000 mg.
Azathioprine
Azathioprine (AZA) (Azasan, Imuran) is a purine analog prodrug. After administration, AZA is rapidly hydrolyzed to 6-mecaptopurine (6MP), which is then converted to active 6-thioguanine (6TGN) which incorporates into DNA to inhibit nucleotide synthesis. A portion of 6-mercaptopurine is also converted to 6-thiouric acid, an inactive metabolite, by xanthine oxidase. The main toxicity of AZA is bone marrow suppression. Pancreatitis has also been rarely reported. Despite its pregnancy category, AZA may be safely used in pregnant transplant recipients.
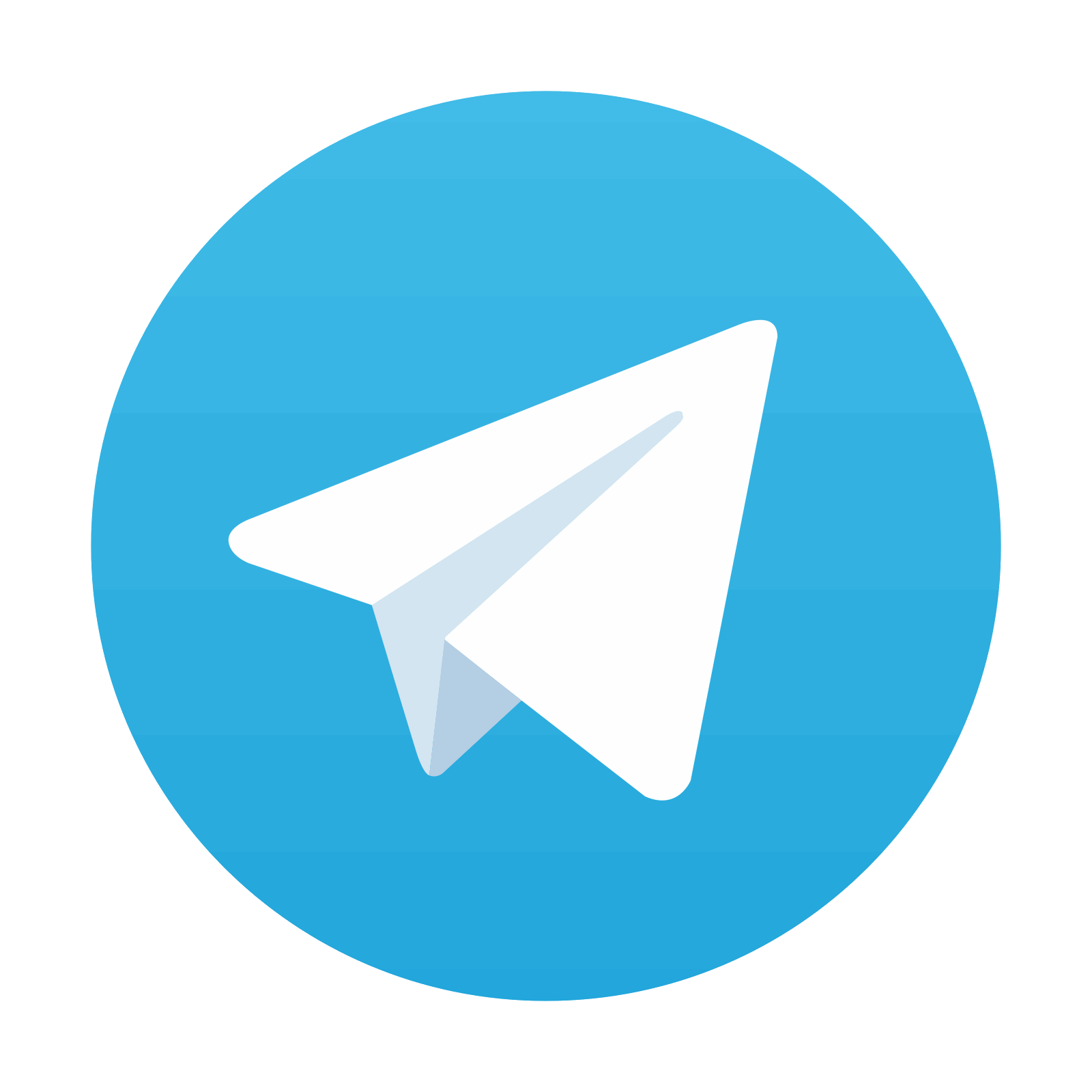
Stay updated, free articles. Join our Telegram channel

Full access? Get Clinical Tree
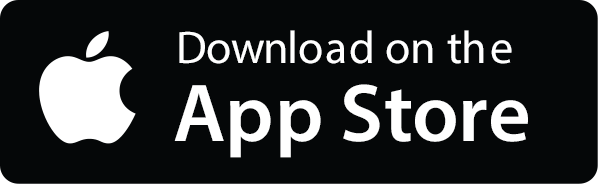
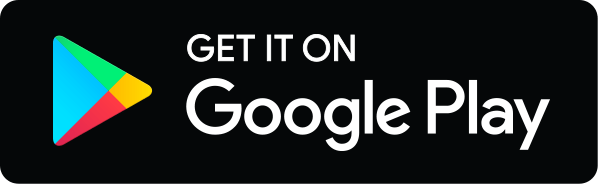