Fig. 1 a,b
a Axial computed tomography (CT) and b fused [18F]- fluorodeoxyglucose (FDG) positron emission tomography computed tomography (PET/CT) images in a patient who had undergone transrectal ultrasound-guided prostate biopsy a few days earlier demonstrate abnormal FDG uptake in a prostatic abscess (arrows)
[18F]-FDG PET has also been investigated for evaluating patients with biochemical recurrence after radical prostatectomy (Fig. 2) [6]. Also, in patients with castrateresistant metastatic disease, a decline in [18F]-FDG avidity of metastases treated with certain chemotherapeutic agents has been correlated with improved clinical response, whereas higher [18F]-FDG uptake in tumors has been associated with shorter progression-free and overall survival [3, 4].
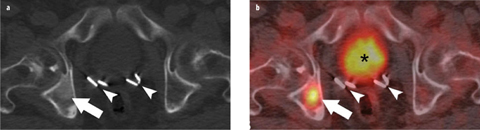
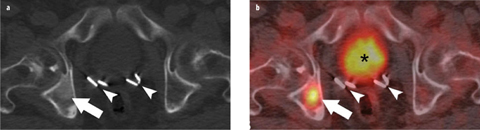
Fig. 2 a,b
a Axial computed tomography (CT) and b fused [18F]-fluorodeoxyglucose (FDG) positron emission tomography computed tomography (PET/CT) images demonstrate a ground-glass-appearing density lesion in the right acetabulum on CT, with abnormal [18F]-FDG accumulation (arrow) consistent with a bone metastasis in a patient with rising prostate-specific antigen (PSA) after prostatectomy. Prostatectomy clips (arrowheads) and physiological [18F]-FDG excretion in the urinary bladder (asterisk) are also shown
Sodium Fluoride (NaF) PET
Sodium fluoride (NaF), typically labelled with 18F, is rapidly cleared from plasma in a biexponential manner. After a single pass, essentially, the entire amount of tracer administered is retained by bone. Bone uptake is related to chemisorption, with exchange of the 18F- ion for the OH- ion on the surface of the hydroxyapatite matrix of bone to form fluoroapatite and migration of 18F- ion into the crystalline matrix. The diagnostic performance of PET with [18F]-NaF is superior to that of standard bone scintigraphy, yielding higher-quality images with rapid blood clearance and high bone-to-background ratio and requiring less time from tracer administration to imaging (Fig. 3). Even-Sapir and colleagues [7] compared technetium-99m (99mTc)-methylene diphosphonate planar bone scintigraphy, single-positron-emission computed tomography (SPECT), [18F]-NaF PET, and [18F]-NaF PET/CT in 25 men with newly diagnosed prostate cancer, with Gleason scores ⩾8 or prostate-specific antigen (PSA) levels ⩾20 ng/ml or nonspecific sclerotic lesions on CT, as well as 19 patients referred for evaluation of suspected disease recurrence or progression. In a patient-based analysis, sensitivity and specificity were 70% and 57%, respectively, for planar bone scintigraphy, 92% and 82%, respectively, for multiple field of view (FOV) SPECT, 100% and 62%, respectively, for [18F]-NaF PET, and 100% and 100%, respectively, for [18F]-NaF fluoride PET/CT. The high sensitivity and specificity of [18F]-NaF PET/CT allows detection of occult bone metastases that are missed on standard bone scintigraphy (Fig. 3), with important implications for patient management.
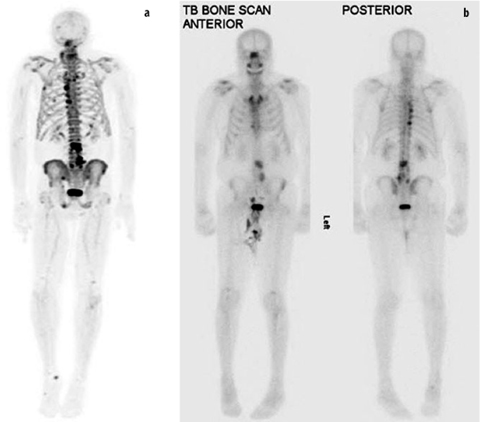
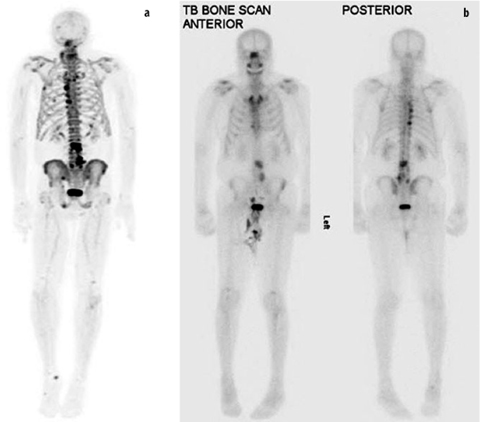
Fig. 3 a,b
a Three-dimensional maximum intensity projection (MIP) from [18F]-sodium fluoride (NaF) positron emission tomography (PET) demonstrates an increased number and conspicuity of multiple bone lesions compared with anterior and posterior planar views from b technetium-99m (99mTc)-methylene diphosphonate bone scintigraphy in a patient with metastatic prostate cancer
[18F]-NaF PET has also been used to monitor treatment response. A small pilot study of five patients with castrate- resistant metastatic prostate cancer showed that semiquantitative [18F]-NaF PET was more accurate than qualitative comparison of scans in assessing response of bone metastases to 223Ra-chloride (Alpharadin, Algeta) therapy and correlated better with PSA and alkaline phosphatase changes [8]. Despite potential advantages of [18F]-NaF PET/CT over standard bone scintigraphy in quantifying treatment response, it should be emphasized that the therapy-induced “flare” phenomenon described with bone scintigrams is also present on [18F]-NaF [9].
Choline PET
Choline can be labeled with 11C or 18F. It enters the cell through choline transporters and is the precursor for the biosynthesis of phospholipids, which are major components of the cellular membrane. Overexpression of choline kinase as a result of increased cellular membrane synthesis in tumors forms the biological basis for choline accumulation in prostate cancer [10].
In primary prostate cancer, reported detection rates using choline PET vary widely, probably in part because of heterogeneity in the different study populations with regard to tumor location, size, aggressiveness (e.g., Gleason score) and PSA kinetics [11, 12]. For detecting prostate cancer recurrence, sensitivities ranging between 38% and 98% have been reported using [11C]- and [18F]- choline PET [13, 14]. Similar diagnostic performance for detecting prostate cancer have been reported in studies comparing [11C]-choline or [18F]-fluorocholine with [11C]-acetate and [18F]-NaF PET/CT [15, 16]. In prostate cancer bone metastases, the extent of choline uptake is inversely related to lesion attenuation on CT (lytic lesions on CT demonstrated higher choline uptake than blastic lesions) [17].
Acetate PET
Acetate is preferentially transported across the cellular membrane through the monocarboxylate transporter. The major sources of acetate consumption are Krebs cycle and metabolic pathways related to production of phospholipids in cellular membranes. Acetate is typically labelled with [11C]. An [18F]-labeled formulation of acetate is also available (the 110-min half life of 18F is usually preferred over the 20-min half life of [11C]), but experience with this tracer is limited [18]. Cancer-induced upregulation of fatty-acid synthase is the biologic basis for acetate uptake in prostate cancer. As with other tracers, there can be considerable overlap among uptake levels in primary prostate cancer, benign prostatic hyperplasia, and normal prostate gland, but generally, [11C]-acetate uptake appears to be greater in cancerous than in noncancerous tissue [19]. Detection rates of up to 75% have been reported when using [11C]-acetate for tumor recurrence assessment [20, 21]. As with all other imaging modalities, detection rates are dependent on serum PSA levels (in one study, positive findings occurred in 59% of patients with serum PSA levels >3 ng/ml and in 4% of patients with serum PSA levels ⩽3 ng/ml) [22].
Fluorothymidine (FLT) PET
FLT is phosphorylated by thymidine kinase (TK) 1, which is retained in proliferating cells without DNA incorporation [23]. FLT is typically labelled with 18F. Kukuk et al. [24] used PET to determine the pharmacokinetics of [18F]-FLT, [18F]-FDG, and [11C]-choline in hormone-dependent and hormone-independent prostate cancer xenograft mouse models. The highest levels of [18]-FLT and [18F]-FDG uptake were found in hormone-independent tumors. [18F]-FDG uptake was also high in hormonedependent tumors but decreased significantly after androgen deprivation therapy. A potential disadvantage to the use of [18F]-FLT PET in assessing prostate cancer is the high level of physiologic accumulation in bone marrow, which may hinder detection of prostate cancer bone metastases.
Fluoro-5-Methyl-1-è-D-Arabinofuranosyluracil (FMAU) PET
FMAU is a thymidine analog phosphorylated by TK and incorporated in DNA. Whereas FLT is phosphorylated by the cytosolic TK1, FMAU is phosphorylated by the mitochondrial TK2 [25]. FMAU is typically labelled with 18F and shows little physiologic accumulation in bone marrow and urinary tract, which has theoretical advantages for imaging prostate cancer. Sun et al. [26], in a small study, showed that [18F]-FMAU accumulated in locally recurrent prostate cancer tumors (tumor-to-background pelvis activity ratio 2.3–6.3) as well as in prostate cancer bone metastases (tumor-to-background normal bone activity ratio 2.4–3).
Androgen Receptor (AR) PET
The androgen receptor (AR) plays a central role in the pathogenesis of castrate-resistant prostate cancer, and novel therapies directed against the androgen receptor have shown a clear survival benefit in this patient population. The effects of androgens are exerted via the nuclear AR, which is a ligand-dependent transcription activator involved in cellular proliferation and differentiation [27]. Almost all patients initially respond to androgen deprivation, but virtually all patients will eventually progress to a castration-resistant clinical state, which is thought to result from bypassing or sensitizing the AR pathway. Several ligands for the AR have been developed, including 16β-[18F]-fluoro-5κ-dihydrotestosterone ([18F]-FDHT) [28]. Initial results from a study comparing [18F]-FDHT and [18F]-FDG PET in men with castration-resistant prostate cancer has shown that there are AR-predominant, glycolysis-predominant, and AR-glycolysis-concordant phenotypes (Fig. 4), which may have important prognostic and therapeutic implications [29]. Moreover, despite encouraging antitumor activity with enzalutamide in men with castration-resistant prostate cancer (e.g., decline in PSA, documented response in soft-tissue disease, stabilization of bone disease, and conversion from unfavorable to favorable circulating tumor cell counts), the enzalutamide- induced [18F]-FDHT uptake changes in tumor did not necessarily parallel changes in tumor [18F]-FDG uptake, suggesting that [18F]-FDHT may be a pharmacodynamic marker as opposed to a treatment-response marker in this setting [30]. Further research is being conducted to elucidate the role of FDHT-PET in patients with advanced prostate cancer.
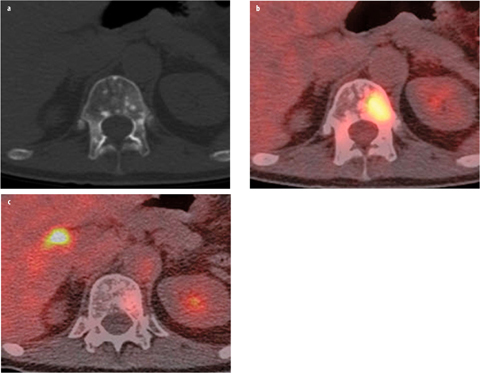
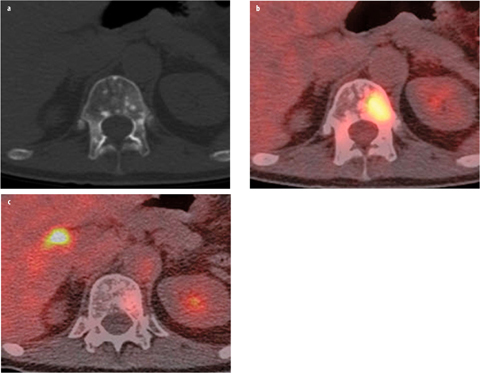
Fig. 4 a–c
a Axial computed tomography (CT), b fused [18F]-fluorodeoxyglucose (FDG) positron emission tomography computed tomography (PET/CT), and c fused [18F]-fluoro-5κ-dihydrotestosterone (FDHT) PET/CT images demonstrate the biological diversity of bone metastases in patients with castration-resistant prostate cancer. Subtle ground-glass-appearing and miliary density lesion is present in the T12 vertebral body, with marked glycolytic activity but minimal androgen expression, as evidenced by uptake on FDG but not on FDHT PET
Amino Acid PET
Enhanced amino acid transport and protein synthesis in tumor cells is the basis for labeling natural or synthetic amino acids for imaging with PET. Different amino acids have been used for this purpose. In one study [31], [11C]- methionine was compared with [18F]-FDG in ten patients with progressive prostate cancer. Index lesions demonstrated uptake of both tracers, but compared with [18F]- FDG, [11C]-methionine showed a higher tumor-to-blood ratio, more rapid tumor uptake after tracer administration, and a flatter uptake profile. There is increasing interest in the use of the synthetic amino acid anti-1-amino- 3-[18F]-fluorocyclobutyl-1-carboxylic acid (FACBC), an l-leucine analog [32]. The extent to which this tracer accumulates in prostate cancer tissue correlates with the expression level of alanine-, serine-, and cysteine-preferring system-mediated amino acid transport. Also, the tracer does not get incorporated into proteins. Schuster et al. [33] described their initial experience with anti- [18F]-FACBC PET in prostate cancer. Visual analysis detected malignancy in all four men with local recurrence, corresponding to 40 of 48 prostate sextants, and in seven of nine pelvic nodal stations. More studies are being conducted to determine the potential incremental value of amino acid imaging over other established tracers, including evaluation of FACBC for detecting recurrent prostate cancer.
Prostate-Specific Membrane Antigen (PSMA) PET
PSMA is a dimeric integral membrane glycoprotein highly expressed on prostate cancer cells and its expression is associated with prostate cancer progression and prognosis. PSMA protein expression can be imaged using labeled monoclonal antibodies (mAb) or small molecules. The most prominent of the mAb are 7E11 (indium-111 [111In]-labeled capromab pendetide; ProstaScint®) and the more recently developed J591. 7E11 is a murine mAb specific for an epitope on the PSMA intracellular domain. As the binding site for this tracer is intracellular, it is only accessible if there is membrane disruption (e.g., dead or dying cells). As a result, the number of available targets for tracer binding is limited (i.e., tracer does not bind to cancer cells without membrane disruption); thus, SPECT with 111In-7E11 has shown low sensitivity for detecting viable tumor sites. Furthermore, 111In-capromab pendetide does not bind to viable prostate cancer sites in bone (the most common site of metastatic disease), and in contrast to PET, SPECT remains only semiquantitative in the clinical setting.
Recently, 7E11 was imaged using a new approach in which 7E11 is labelled with the PET nuclide zirconium- 89 (89Zr) [34]. 89Zr is ideally suited for targeted imaging probes, especially antibodies, as it has unique physical decay properties and energy (t1/2=78.43 h, β+=22.3%,Eβ+, max=901 KeV, Eγ=909 KeV). The greater half-life provides sufficient activity at the required circulation times for optimal targeting to disease sites, enabling imaging at later time points, including 120 h postinjection, an imaging window difficult to achieve with the shorter-lived PET nuclides such as 18F. The effects of chemotherapy, chemical castration, or radiotherapy may be monitored by observing 7E11 uptake, which increases with progressing cell membrane disruption resulting from treatment-induced cell death [34]. The uptake of 89Zr-7E11 correlates strongly with markers of cell death and apoptosis [34].
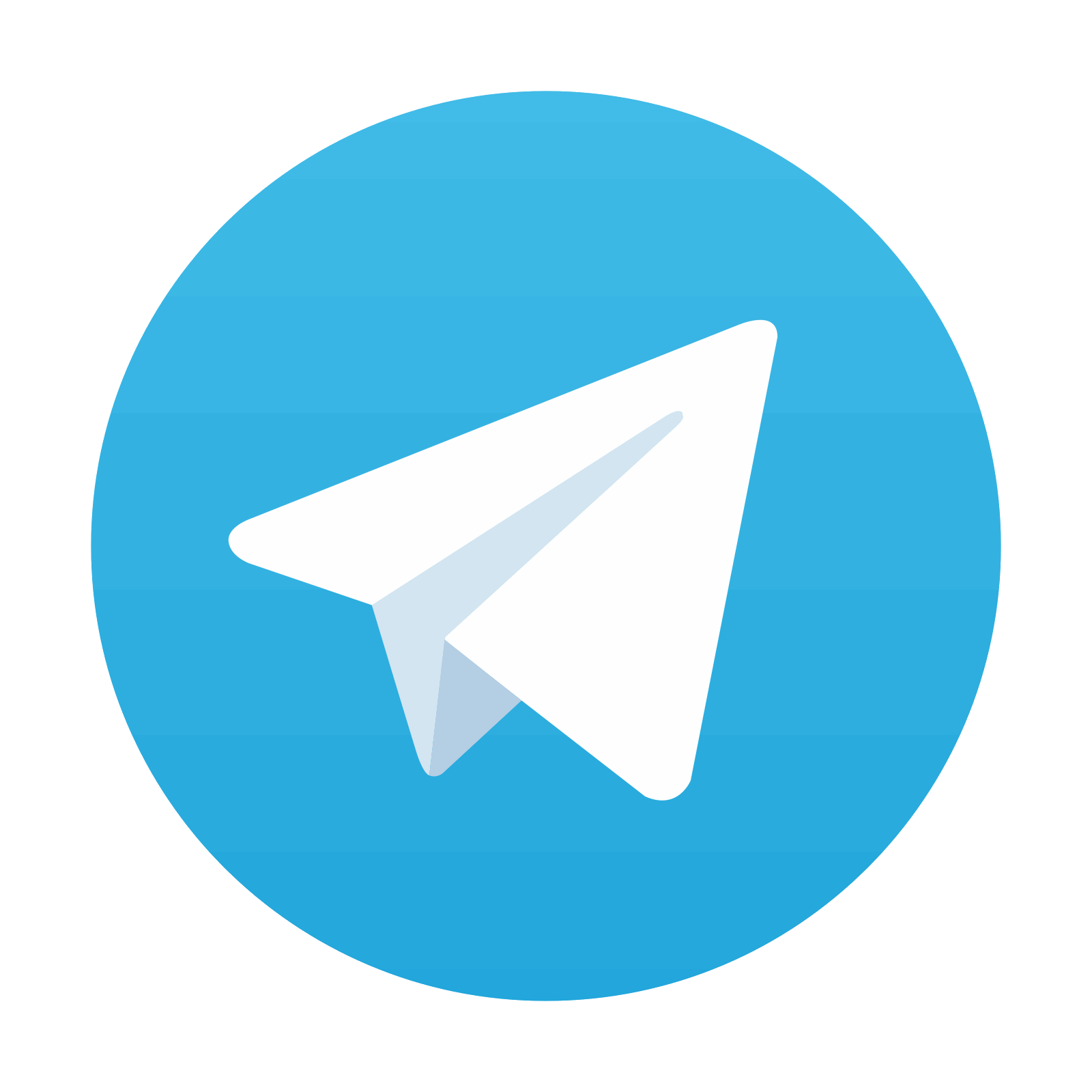
Stay updated, free articles. Join our Telegram channel

Full access? Get Clinical Tree
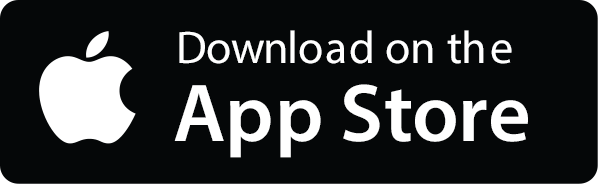
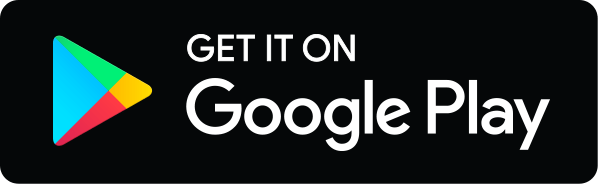