133 Michael R. Folkert,1 Neil B. Desai,1 & Yoshiya Yamada2 1 Department of Radiation Oncology, University of Texas Southwestern Medical Center, Dallas, TX, USA 2 Department of Radiation Oncology, Memorial Sloan Kettering Cancer Center, New York, NY, USA Prostate cancer remains the most common cancer in men; for 2018, an estimated 164 690 cases will be diagnosed (19% of all cancers), with 29 430 deaths (9% of all cancers in men) [1]. Only 10% of prostate cancer diagnoses in the prostate specific antigen (PSA) testing era are high‐risk prostate cancers, which justifies approaches that focus treatment on the prostate itself and minimize impact on quality of life. According to the National Comprehensive Cancer Network (NCCN) guidelines [2], reasonable approaches to the management of non‐metastatic prostate cancer include surgical approaches and radiation approaches (external beam radiation therapy (EBRT), brachytherapy, or a combination of both), potentially supplemented by androgen‐deprivation therapy. EBRT using relatively few sessions of highly focused, high‐dose‐per‐session radiation therapy (hypofractionation, generally referred to as stereotactic body radiation therapy (SBRT) or stereotactic ablative radiosurgery (SABR)) [3, 4] is becoming more widely accepted as well, but is practiced primarily in experienced centers. Brachytherapy is an approach that allows permanent (low dose rate, or LDR) or temporary (high dose rate, or HDR) delivery of radioactive isotopes within the prostate, which deliver localized therapeutic dose though decay of radioactive isotopes and emission of radiation. Other treatment modalities, including cryotherapy [5] and radiofrequency ablation (RFA) [6] appear to be effective in select cases, but are not yet considered standard of care. In this chapter we review the history, techniques, and outcomes of brachytherapy approaches in the management of prostate cancer. Prostate brachytherapy is one of the oldest radiation therapy techniques, first described by Pasteu and Degrais in 1913 [7] and brought into regular use by Young, the pioneer of the radical perineal prostatectomy [8, 9]. Interstitial techniques were originally performed with digital guidance alone using transperineal radium needles as reported by Barringer, a urologist practicing at the New York Memorial Hospital in his 1924 publication, detailing cases starting as early as 1915 [10]. This needle‐based approach was later supplanted by less expensive gold‐encapsulated seed treatments [11]. Due to poor visualization of the target, these techniques yielded poor oncologic outcomes, and were not widely adopted. Subsequently, an open surgical retropubic approach was utilized by Whitmore and colleagues at Memorial Sloan Kettering Cancer Center starting in 1970, which also included a bilateral pelvic lymphadenectomy [12, 13]. Local control was still poor with this technique (only 44% at 10 years in node‐negative patients), which was felt to be due to suboptimal seed distribution within the prostate gland. In addition, the procedure required a laparotomy, and soon lost favor compared to modern prostatectomy and EBRT approaches. Image‐guided (ultrasound‐based) transperineal prostate brachytherapy was introduced by Holm et al. in 1983 [14] and subsequent developments in multiplanar transrectal ultrasound (TRUS) allowed for safe and effective transperineal placement of radioactive sources [15–17]. Subsequently, multiple techniques have been developed incorporating imaging both before (preplanning) and during (intraoperative) LDR brachytherapy implantation procedures to deliver optimal therapy, and robust long‐term outcomes and toxicity data have been generated through prospective trials and large institutional experiences. In addition, new techniques for temporary HDR brachytherapy have been developed, after Yoshioka et al. [18] reported the favorable results of HDR brachytherapy as monotherapy for various risk groups of localized prostate cancer in 2000. Guidelines for LDR and HDR prostate brachytherapy have been established by the American Brachytherapy Society (ABS) [19, 20] and its role in the management of prostate cancer is further delineated in the NCCN guidelines [2]. Technical standards for prostate brachytherapy are also well established, with formal roles for the physician and medical physicist and reporting guidelines set by the American Association of Physicists in Medicine (AAPM) [21]. Dosimetric parameters and reporting for permanent prostate brachytherapy are also carefully defined in the AAPM Task Group reports 43 and 137 [22, 23]. In terms of radiation‐based treatment approaches in the management of prostate cancer, brachytherapy is appealing in that it is the most conformal treatment available for the prostate, focusing the vast majority of radiation exposure on the target organ while limiting dose to the rest of the body more than any other radiation technique, including advanced charged‐particle therapy. In addition, the treatment can be delivered in a single session with certain approaches. Prostate brachytherapy is generally an outpatient procedure and the patient may resume 100% of physical activity within 24 hours after implantation and treatment delivery is complete. Appropriate patient selection is crucial in the application of prostate brachytherapy. Guidelines have been set forth by the American Urological Association (AUA) [24] and the ABS [19, 20]. According to the ABS, there are several absolute contraindications to TRUS‐guided permanent prostate brachytherapy, including inability to tolerate spinal or general anesthesia; the presence of distant metastases (identified clinically or on metabolic or cross‐sectional imaging); large defects from a prior transurethral resection of the prostate (TURP) that would preclude effective seed placement; life expectancy of less than 10 years; or the presence of the ataxia telangiectasia (AT) mutation, which would predispose to excessive toxicity from radiation. Other considerations include a high International Prostate Symptom Score (IPSS) [25] of >20 (although it was noted that most prospective studies have used a cutoff score of 15 or 18), history of prior radiation therapy to the pelvis, large median lobes (e.g. Figure 133.1), prostate volume >60 ml at the time of implant, or inflammatory bowel disease [26–29]. For patients with enlarged prostates that would present a technical challenge to implant (due to pubic arch interference, for example, as shown in Figure 133.2), cytoreduction with androgen‐deprivation therapy (ADT) is an option, with average reduction in prostate volume of approximately 30% after 3–4 months [30, 31]. Both bicalutamide and luteinizing hormone‐releasing hormone analogs (LHRHa) can provide effective ADT‐based cytoreduction; in a study by Petit et al. [32] median cytoreduction was 26% for bicalutamide and 32% for LHRHa methods, with greater sexual function‐related toxicity in the LHRHa group. Figure 133.1 Median lobe enlargement. (a) Axial and (b) sagittal views, MRI T2‐weighted fast spin echo sequences. Figure 133.2 Pubic arch interference in transperineal prostate brachytherapy. Prostate brachytherapy is ideal for the management of prostate cancer confined to the prostate gland, similar to radical prostatectomy. The risk of extraprostatic disease should be considered when selecting treatment. ABS recommendations based on NCCN risk group suggests that prostate brachytherapy alone without supplemental EBRT or ADT is appropriate for low‐risk prostate cancer, as well as intermediate‐risk prostate cancer; of note, for those patients with high‐ to intermediate‐risk disease, supplemental EBRT and/or ADT should be considered [33]. Brachytherapy alone (monotherapy) is not recommend for patients with high‐risk prostate cancer, and supplemental EBRT and ADT are favored [20]. The recommendations are mirrored in NCCN guidelines for the management of prostate cancer [2]. According to the ABS guidelines, there is no absolute lower or upper age limit for prostate brachytherapy, and studies have not shown a significant difference in outcome for patients treated at a younger age (<60 years) compared to patients treated over 60 years of age [34]. A common issue raised when deciding between radiation and surgery‐based approaches in the management of prostate cancer is the risk of a secondary cancer from exposure to radiation therapy, particularly bladder and rectal cancers [35–37]. However, when examining the risk of secondary cancers by treatment modality, it has been noted that brachytherapy does not carry the same association with secondary cancers as EBRT, likely due to its highly conformal nature with minimal exposure to normal tissue [36, 38–40]. The possibility of an increased risk of secondary cancers, especially if brachytherapy is combined with EBRT, should still be discussed with each patient. Brachytherapy (both LDR and HDR) may be used as salvage therapy in the setting of a localized prostate recurrence [2]. Brachytherapy implantation is generally performed according to the method described by Holm et al. [14, 15] via a transperineal approach with real‐time ultrasound visualization of the prostate. The TRUS probe is generally mounted on a stepper unit attached to a stabilizer arm, which supports the probe and template grid and facilitates a calibrated caudal/cephalad motion (Figure 133.3). Accurate placement of the needles is achieved with this technique, which allows real‐time adjustment of implanted needles relative to the target organ (Figure 133.4). Once needle placement is confirmed, the radioactive isotopes may be delivered through them into the substance of the prostate gland, either permanently (LDR approaches using 125I, 103Pd, 131Cs, see Figure 133.5) or temporarily (HDR approaches generally using 192Ir afterloader) (Figure 133.6). Figure 133.3 Transrectal ultrasound probe mounted on stepper/stabilizer unit for brachytherapy procedures. Figure 133.4 Implanted needles in the prostate gland visualized on (a) fluoroscopic and (b) ultrasound imaging. Figure 133.5 Postprocedure fluoroscopic images of implanted seeds: (a) 125I implant; (b) 103Pd implant. Figure 133.6 Typical afterloader used in high dose rate brachytherapy procedures (Varian Varisource IX afterloader, Varian Inc.). Perhaps the most common isotope used for permanent prostate brachytherapy is iodine‐125 (125I) first introduced in 1965, which is often sold in the form of silver iodide deposited on a silver rod, sealed in a titanium shell. 125I emits photons from electron capture decay (half‐life (T½) of 59.4 days) with energies ranging from 27.4 to 35.5 keV, as well as lower energies resulting from interaction with the seed materials (average energy 27.4 keV). Palladium‐103 (103Pd) was introduced in 1986. 103Pd decays in the same manner as 125I but with a shorter half‐life (T½ of 17 days), emitting photons with energies of 20.1 and 23 keV. Cesium‐131 (131Cs) was introduced in 2004 for permanent prostate brachytherapy. 131Cs has the shortest half‐life of the standard commercially available radionuclides (T½ of 9.7 days) with photon energies ranging from 29.5 to 34.4 keV (average energy 30.4 keV), similar to 125I. This allows dose delivery over a shorter overall time interval, thus limiting the period during which patients must follow radiation safety precautions [19, 21]. It has been hypothesized that a more rapid rate of dose delivery would result in a greater activity against high‐grade tumors due to their faster doubling time [41], but this has not been borne out in multiple studies. In a prospective randomized trial comparing 125I versus 103Pd implants by Wallner et al. [42] there was no difference in 3‐year actuarial biochemical control rates for patients with low‐risk prostate cancer. In a more recent study by Kollmeier et al. that included patients with low‐, intermediate‐, and high‐risk localized prostate cancer [43], no differences were noted in long‐term genitourinary or gastrointestinal toxicity or 5‐year biochemical relapse‐free survival for patients treated with 125I versus 103Pd implants. The “strength” of the source, measured by its activity, may play a role; in a randomized trial by Narayana et al. [44] higher strength 125I implants were found to have improved dosimetric coverage at a lower overall seed cost, with shorter operating room times; the study was not designed to assess long‐term toxicity or clinical outcome. As optimal dosimetry requires placement of seeds in a distribution that provides optimal coverage of the prostate gland while reducing dose to critical nearby structures (urethra, bladder/bladder neck, and rectum), careful planning is required to determine appropriate seed placement. This can be done in advance of the actual operative procedure (preplanning) or on the same day of the procedure (intraoperative planning) [45]. In general, patients undergo a gentle bowel preparation prior to the day of their planning procedure with Fleet’s enemas or laxatives; patients undergoing both planning and treatment on the same day following an intraoperative protocol require a more thorough bowel preparation such as HalfLytely. Percutaneous brachytherapy treatment planning is performed by placing the patient in extended lithotomy position, and introducing the TRUS probe into the rectum (Figure 133.3). The prostate and surrounding organ anatomy is surveyed to identify possible issues during treatment, such as pubic arch interference (see, for example, Figure 133.2), which would interfere with anterior needle placement; this can also be assessed in part by prior cross‐sectional (CT or MRI) imaging [46]. The “volume study” used to generate the plan is constructed by acquiring consecutive axial ultrasound images at 5 mm intervals from the base of the prostate to the apex, with a superimposed and calibrated template on each image. Longitudinal images are also acquired to ensure that no axial slices were missed and to verify the base to apex length measurement [21] (Figure 133.7). Figure 133.7 Three‐dimensional reconstruction of prostate volume study. Bladder depicted in yellow, prostate in light green, urethra in dark green, and rectum in blue. The prostate is encompassed by a dose cloud in red generated by the implanted seeds. The preplanning approach generally calls for linked or stranded seeds to be placed based on an implant plan generated from the TRUS‐based “volume study” acquired prior to the actual procedure date, although a preplan can also be used to generate a manual/individually placed seed distribution. One advantage of techniques using stranded seeds is that the seeds are much less likely to migrate from their planned positions within and around the prostate; this has been evaluated in a prospective trial by Reed et al. [47] in which they compared two groups of patients; one group used seeds held in a #1 Vicryl strand, and the other used loose seeds. They did not identify a significant dosimetric difference between the two approaches, although there was significantly more seed loss in the loose seed cohort. It is unclear if this seed loss is clinically significant. Saibishkumar et al. also studied the clinical impact of the stranded versus loose seed approach [48] and found that the use of stranded seeds increased unfavorable dosimetric parameters for the rectum and urethra, and resulted in a trend towards increased acute toxicity rate. Intraoperative planning, in comparison, is performed at the time of the implant, and accounts for patient anatomy in the anesthetized state; this process can be done both prior to needle placement [49] or after the needles are placed [45]. While many variations exist for this form of treatment, plans performed prior to needle placement generally utilize preloaded seeds and spacers or stranded seeds, whereas plans generated after needle placement utilize an applicator (such as the Mick Applicator shown in Figure 133.8, Mick Radio‐Nuclear Instruments Inc., now an Eckert & Zigler BEBIG Company, Mount Vernon, NY, USA) that allows individual, manual seed placement according to the plan under direct real‐time ultrasound visualization. Both techniques have been evaluated against preplanned seed placement. Shah et al. compared preplanned brachytherapy against intraoperative, pre‐needle plans and found that the intraoperative plans provided superior dosimetric and biochemical control outcomes [49]. Zelefsky et al. compared preplanned brachytherapy against intraoperative 3D optimized plans following needle placement and found that the intraoperative technique improved both target coverage as well as reducing dose to the urethra and rectal wall [45]. Investigators have also incorporated a “dynamic‐adaptive” planning approach that takes into account the contribution of each manually placed seed as the procedure is performed, allowing for intraoperative modification of treatment to reduce the risk of a suboptimal plan [50]. Figure 133.8 Mick applicator, seed cartridges, and needle placement template grid. Clinical outcomes following brachytherapy administration have been shown to be strongly related to the quality of the implant. This assessment of quality is performed following implant administration in the setting of LDR brachytherapy with 3D imaging of the prostate performed at a set time point after treatment; generally 30 days of 125I implants, although earlier assessments may also be performed (especially for 103Pd and 131Cs, with their more rapid decay). Multicenter pooled studies have been performed to evaluate the prognostic impact of post‐implant dosimetry on ultimate outcome. In a report by Stone et al. [51], data from 3928 permanent brachytherapy implants at six institutions was analyzed, and doses of ≥140 Gy were correlated with improved biochemical control. The dose to the prostate is assessed in terms of the minimum dose delivered to 90% of the prostate volume (D90) as measured on CT imaging [52]. Another multi‐institutional analysis of patients with T1–T2 prostate cancer treated with permanent seed implantation corroborated this conclusion, showing that D90 < 130 Gy was associated with increased risk of biochemical recurrence [53]. Other target dosimetric parameters often assessed include the prostate volume receiving 100% of the prescribed dose (V100) and the prostate volume receiving 150% of the prescribed dose (V150) [22, 23]. Although permanent interstitial brachytherapy with radioactive seeds has been a standard treatment for localized prostate cancer for decades, HDR brachytherapy, using a temporary source introduced using a remote afterloader, is a much more recent advance (see Figure 133.5). HDR brachytherapy is defined as the delivery of radiation at a dose rate of >12 Gy per hour [20]. The technique for HDR temporary prostate brachytherapy is similar to that of LDR brachytherapy performed with intraoperative planning; needle implantation is performed with an anesthetized patient in extended lithotomy position under TRUS guidance. Treatment needles can be placed directly, or “pathfinder” needles may be placed and then replaced with flexible plastic guide catheters. The treatment needles or guide catheters are held in place with a template locked and sutured to the perineum. In some institutions, they may be held in place by a rigid foam or dental sealant. Flexible cystoscopy is generally performed to ensure that the treatment needles/catheters are not inserted into the urethra or through the bladder wall. Imaging is then acquired for treatment planning (ultrasound, CT, or MRI), and a computer‐optimized treatment plan is generated (Figure 133.9). The remote afterloader is then attached to the needles or catheters and treatment is delivered; the implant can then be removed or left in place if additional fractions are planned [54]. Figure 133.9 High dose rate prostate implant plan; 17 needles placed. Foley catheter in place in center. HDR brachytherapy may offer many advantages over LDR brachytherapy, including increased likelihood of tumor control due to the high biological effectiveness of high‐dose fractions (assuming a low α/β ratio for prostate cancer), data suggesting reduced rates of acute and late toxicity relative to permanent seed implantation [55], improved conformality of dose to the prostate (potentially superior to SABR/SBRT [56]), and no permanent radiation sources within the prostate (reducing radiation safety concerns from dose delivery to the patient’s family and associates) [57]. Both the ABS [20] and the American College of Radiology (ACR) have provided guidelines (appropriateness criteria) for the use of HDR brachytherapy in the management of prostate cancer [58]. In terms of patient selection, the ABS recommendations are similar to those for LDR brachytherapy, although an additional absolute contraindication was added for preexisting rectal fistula. Brachytherapy is a reasonable option as salvage treatment for locally recurrent prostate cancer initially treated with EBRT. Because brachytherapy delivers highly conformal therapy, bladder, and in particular urethral and rectal doses can be stringently minimized while high doses can be given to tumor‐bearing prostate tissue, making brachytherapy an excellent treatment option for patients who have failed EBRT. Both LDR and HDR techniques have been reported for the salvage of local radiation failures. Burri et al. [59]
Image‐guided Prostate Brachytherapy
Introduction
History of prostate brachytherapy
Patient selection for prostate brachytherapy
Technical issues in prostate brachytherapy
Approach, visualization, and access for prostate brachytherapy
Low dose rate permanent prostate brachytherapy
Source selection for low dose rate prostate brachytherapy
Pre‐ and intraoperative planning and treatment delivery for LDR brachytherapy
LDR prostate brachytherapy implant quality assessment
High dose rate temporary prostate brachytherapy
Salvage brachytherapy
Stay updated, free articles. Join our Telegram channel

Full access? Get Clinical Tree
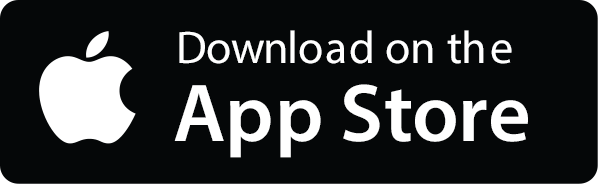
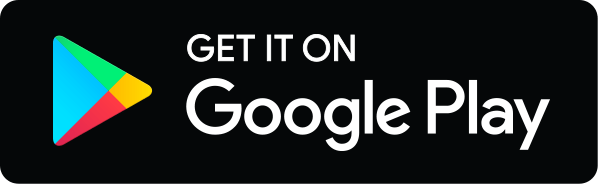