Epidemiology
Hypoplastic left heart syndrome (HLHS) accounts for 5 percent of all congenital heart anomalies and is responsible for 25 percent of cardiac deaths in the first week of life. Its incidence is 1.8 in 10,000 live births, with 25 percent of cases showing associated noncardiac malformations and 5 percent associated with chromosomal abnormalities.
Morphology
HLHS includes a wide spectrum of anatomic abnormalities with the common feature of hypoplasia of the left ventricle (LV) and the ascending aorta. At one end of the spectrum there may be some mild LV hypoplasia, mild aortic stenosis, and aortic coarctation. At the other end there is complete absence of the LV, aortic atresia, and aortic arch interruption.
Pathophysiology
Systemic venous return is channeled via an interatrial communication to the right ventricle (RV) and, through the pulmonary artery (PA) and patent ductus arteriosus (PDA), to the systemic and pulmonary circulations. Balanced physiology ensues, with QP:QS varying according to systemic and pulmonary vascular resistance (PVR) as well as with the unrestrictive nature of the atrial septal defect (ASD).
Clinical features
As a result of ductal closure in the postnatal period, HLHS is uniformly fatal if not treated. Evidence of pulmonary overcirculation is evident on clinical examination once PVR diminishes in early post-natal life.
Diagnosis
Prenatal echocardiography can be used to detect unbalanced ventricles as early as 20 weeks of gestational age. Postnatal echocardiography readily establishes the diagnosis and guides medical and surgical decision-making.
Treatment
Prostaglandin E1 (PGE1) infusion is the cornerstone of early resuscitation, coupled with balloon atrial septostomy in case of a restrictive ASD. A three-stage single-ventricle palliation approach has been adopted by most centers. The first stage involves aortic arch reconstruction and establishment of a reliable source of pulmonary blood flow, and the second and third stages consist of sequential partitioning of the systemic and pulmonary circulations. Transplantation has been used as the initial approach or, more commonly, for those who fail palliative strategy. In high-risk neonates, initial palliation with a hybrid approach (stenting of coarctation and PA banding) should be considered.
Outcomes
Operative survival of stage I Norwood palliation depends on several variables, including anatomic factors (diameter of ascending aorta, operative weight, restrictive ASD, associated anomalies), and nears 80 percent in several series. Operative mortality between 5 and 10 percent has been observed for second- and third-stage palliation. Long-term complications [ventricular failure, atrioventricular (AV) valve regurgitation, arrhythmias, and protein-losing enteropathy, among others] dictate late prognosis and the need for reintervention or transplantation.
Hypoplastic left heart syndrome (HLHS) is a challenging disease that includes a wide spectrum of anatomic abnormalities with the common feature of left ventricular (LV) hypoplasia and hypoplasia of the ascending aorta. At one end of the spectrum there may be mild (LV) hypoplasia, mild aortic stenosis, and aortic coarctation. At the other end of the spectrum, there may be complete absence of the LV, aortic atresia, or an association with interrupted arch. All these neonates present a serious challenge to congenital heart surgeons, cardiologists, anesthesiologists, critical care specialists, nurses, and families. HLHS is uniformly fatal in the absence of intervention. There is now little argument about the utility of staged operative repair in these children as a result of continued improvements in operative techniques and perioperative care.
Therapy for HLHS has been one of the great successes in the treatment of congenital heart disease (CHD). Before the 1980s, HLHS was a uniformly lethal condition. Over the past 30 years, palliation of HLHS has become a standard operation in nearly all institutions. In 1952, Lev1 first described maldevelopment of the left-sided cardiac structures in combination with a small ascending aorta and transverse arch. By 1958, Noonan and Nadas had further defined the syndrome to include a variety of cardiac malformations of left heart structures.2 The first report of any attempt to palliate a patient with mitral atresia was in 1961 by Redo and associates, who performed an atrial septectomy using inflow occlusion through a right thoracotomy; the patient died soon after the operation.3 In 1968, Sinha and coworkers outlined management principles that remain in use today that include creation of an unobstructed atrial communication, unrestrictive systemic flow, and control of pulmonary blood flow.4 Cayler and colleagues described an anastomosis between the right pulmonary artery (PA) and the ascending aorta with banding of both right and left PAs.5 Interestingly, 40 years later, PA banding is being employed for selected children in whom medical conditions or anatomic substrates render them unsuitable for stage 1 Norwood reconstruction. This first-stage hybrid procedure involves stenting the ductus arteriosus and atrial septal defect, with placement of bilateral PA bands.6,7 Litwin, Mohri, and others all performed unsuccessful operations that were variations of these principles, which nevertheless contributed to the development of the knowledge of the disease and its palliation.8,9 In 1977, Doty and Knott described primary reconstruction that included atrial septation and a right atrium (RA)-to-PA Fontan circuit.10 Again, though no patients survived, this experience confirmed that one-stage reconstruction would not be successful because of high neonatal pulmonary vascular resistance (PVR). Levitsky, Behrendt, and others described multiple, possible surgical procedures.11,12 Although the procedures demonstrated no long-term success, they established the principle of staged reconstruction as first described for tricuspid atresia by Fontan and Kreutzer. This included a strategy of initial palliation followed by eventual separation of the systemic and pulmonary circulations.13,14 However, it was William Norwood who provided the seminal contribution in 1980 and in 1983, with the first report of a successful staged approach.15,16 The Norwood procedure remains the primary reconstructive approach to this day.
From a molecular standpoint, the developmental mechanisms that underlie HLHS remain obscure, although steady progress continues.17 Multiple genetic loci are associated with HLHS including the chromosomal abnormalities of trisomy 13 and 18, Turner (XO), and Jacobson (11q deletion) syndromes. Single gene defects with potential associations include GJA1,18 NKX2-5,19 NOTCH1,20 and HAND1.21 Furthermore, using a genome wide linkage approach, two loci (10q and 6q) have been associated with HLHS, suggesting that new technologies may provide important information.22 Embryologically, there are additional clues. The severe hypoplasia of left heart structures is associated with, and likely a consequence of, limited flow during development secondary to a primary abnormality of either LV inflow or outflow. Primary defects of myocardial growth are unlikely to be a mechanism for this disease, since the myocardium appears normal.23,24 In addition, in approximately 5 percent of patients with aortic atresia, an unrestrictive ventricular septal defect coexists and, in such cases, there is nearly always normal development of the LV and mitral valve.
Patients can be categorized on the basis of atrioventricular (AV) and semilunar valvular morphology into three primary subsets: (1) aortic atresia with mitral atresia (40 percent), (2) aortic stenosis with mitral stenosis (30 percent), and (3) aortic atresia with mitral stenosis (30 percent) (Fig. 76-1). Aortic stenosis with mitral atresia is rare. HLHS variants include a malaligned AV canal; double-outlet right ventricle (RV) with mitral atresia; tricuspid atresia with transposed great arteries, and a univentricular heart with aortic stenosis. There is frequently leftward and posterior deviation of the septal attachment of septum primum, but this is unlikely to be a common developmental mechanism, since it is seen commonly in other CHD patients. Usually, the superior and inferior venae cavae (SVC and IVC) are connected normally to the RA, although in about 15 percent of patients a left SVC draining to the coronary sinus is present. Other structural abnormalities of the heart are rare, with less than 5 percent of patients demonstrating valvular dysplasia. Also rare (less than 5 percent) is the association with abnormalities of pulmonary venous return or interruption of the aortic arch. Abnormalities in brain development have been reported with increasing frequency in children with severe CHD, and may define a high-risk group for operative repair.25 The pulmonary vascular tree also harbors abnormalities, with an increase in number and muscularity of vessels.26,27
Figure 76-1
Anatomy and physiology of hypoplastic left heart syndrome. Hypoplastic left-sided structures force pulmonary venous blood to flow from the left atrium through the interatrial communication to the right atrium (downward arrow). Blood from the right ventricle flows both to the pulmonary circulation via the pulmonary artery (upward arrow) and to the systemic circulation via the ductus arteriosus. Maintenance of ductal patency is critical for survival. The surgical principles of repair aim at maintaining this stable physiology. (Reprinted with permission from Gruber P, Spray T, et al. Hypoplastic left heart syndrome. In: Kaiser LK, Kron IL (eds). Mastery of Cardiothoracic Surgery, 2nd ed. Philadelphia: Lippincott Williams & Wilkins, 2007:936.)

A normal fetus has a parallel circulation that adequately supports single-ventricle physiology before birth. Three communications (the ductus venosus, foramen ovale, and ductus arteriosus) shunt oxygenated placental blood largely past the hepatic and pulmonary beds to supply the splanchnic circulation. HLHS is well supported in this situation and, as a result, it is rarely a cause of fetal demise. HLHS may be a secondary result of early obstructive lesions of either mitral or aortic valvular development, and this hypothesis has been supported in animal models of mitral or aortic stenosis with resulting LV hypoplasia.24 However, the primary cause of the obstructive flow lesion that may secondarily lead to HLHS is unknown. Interestingly, there are no known genetic animal models that recapitulate HLHS despite the existence of a large number of mutations that affect valvular development. This argues for a complex early multifactorial event or a transient early insult.
HLHS is a uniformly fatal disease if untreated. It represents 5 percent of all cases of CHD and is responsible for nearly 25 percent of cardiac deaths in the first week of life. Among 10,000 live births, approximately 1.8 are born with HLHS, with a slight male predominance. Of these, 25 percent will also have a noncardiac anomaly, and 5 percent a chromosomal abnormality (most typically trisomy 13, 18, or 21). Syndromic lesions are rare but, among these patients, Turner syndrome (monosomy X) is the most common. The recurrence risk is 2.2 percent for one affected sibling and 6 percent for two affected siblings, suggesting a genetic predisposition but arguing against a simple effect.
The presentation of infants with CHD has changed dramatically over the past 15 years. In most large centers, the majority of patients are identified through prenatal echocardiography; this early identification has not been correlated consistently with a better outcome. Although some tachypnea and mild cyanosis may be present, it is not until the ductus arteriosus begins to close that children with HLHS manifest evidence of impaired systemic perfusion with pallor, lethargy, and diminished femoral pulses. Cardiac examination reveals a dominant RV impulse, a single second heart sound, and often a nonspecific soft systolic murmur. Electrocardiographic examination reveals RA enlargement and RV hypertrophy. Chest x-ray occasionally shows mild cardiomegaly and increased pulmonary vascular markings.
Physical examination of children with HLHS is usually normal. The examination is determined by the underlying anatomy as well as the chronicity of the disease (ductal closure and PVR). Poor perfusion, weak distal pulses that may not be present because of the size of the ductus, acidosis, and a sepsis-like picture may confound the diagnosis. In the absence of risk factors or laboratory findings consistent with sepsis, one should look for left-sided obstructive lesions.
There are no specific laboratory indicators of HLHS. These patients usually exhibit normal values. With ductal closure and malperfusion, end-organ compromise may be reflected by evidence of metabolic acidosis as well as altered hepatic and renal function tests.
At 20 weeks of gestation, a fetal echocardiogram has reasonable visualization of the cardiac structures. It is, however, neither feasible nor cost-effective to screen all pregnancies. Therefore, a selective approach is currently taken by which only mothers at high risk are screened. Ventricular size discrepancy is frequently the first hint of impending problems. Certainly, the presence of an intact or restrictive atrial septum should prompt term high-risk delivery in an institution where an urgent postdelivery atrial septectomy or catheter-based septostomy and atrial stenting can be rapidly performed. The use of prenatal screening improves the prenatal condition of the child but may not translate into an improved outcome (at least in cases of transposition of the great arteries or HLHS).28,29 After delivery, the infant should undergo two-dimensional and Doppler echocardiography to define anatomy to an extent sufficient for medical and surgical decision-making. It is important to distinguish HLHS from other diseases that may mimic certain of its features, as their management may differ substantially.
Chest radiography often demonstrates mild cardiomegaly and excessive pulmonary blood flow. A head ultrasound should be obtained in all patients to rule out intracranial hemorrhage and minimize the risks of heparinization. Patients with medical necrotizing enterocolitis should have a course of intravenous antibiotics before first-stage palliation.
Regardless of the anatomic subtype, preoperative stabilization is critical to the ultimate outcome of patients with HLHS. Nearly all patients with suspected HLHS are transported on prostaglandin E1 (PGE1) at a dose of 0.01 to 0.025 μg/kg/min. Two clinically dose-dependent side effects of PGE1 are hypotension and apnea, though they are infrequently clinically significant. Umbilical arterial and umbilical venous lines are used for monitoring and central access in most patients. Most infants can ventilate with a natural airway and indeed will often have more favorable hemodynamics while extubated. Supplemental oxygen should generally be avoided, as it will act as a pulmonary vasodilator, decreasing PVR, increasing Qp:Qs, and thus decreasing systemic perfusion. Inotropic support is required in patients who have had a perinatal insult, but is otherwise rarely necessary. The goal of these maneuvers is to get the patient to the operating room as stable as possible.
There are two primary therapies for HLHS: (1) staged reconstructive surgery leading to a modified Fontan–Kreutzer procedure and (2) heart transplantation. Heart transplantation is discussed in detail in Chapter 89; this chapter focuses on staged reconstructive surgery.
Over the past 25 years, the Norwood procedure has evolved to become the standard approach in nearly all institutions that care for neonates and infants HLHS. There are three primary goals of first-stage palliation: (1) establishment of unrestricted interatrial communication to provide complete mixing and avoidance of pulmonary venous hypertension, (2) establishment of reliable source of pulmonary blood flow, allowing for the development of pulmonary vasculature and for the minimization of the volume load on the single ventricle, and (3) provision of unobstructed outflow from the ventricle to the systemic circulation. Most institutions will offer surgical palliation to nearly all patients with HLHS, very low-birth-weight infants, and those with non-lethal genetic syndromes.
Two perfusion techniques can be used for first-stage palliation: deep hypothermic circulatory arrest (DHCA) and selective antegrade continuous cerebral perfusion. At present, there is no consensus on the superiority of one approach over the other.30
The child is brought to the operating room and ventilated on room air, with care taken to avoid hyperventilation. A full midline sternotomy is performed, and the thymus is removed in its entirety, with care being taken to avoid the phrenic nerves. The pericardium is opened and an obligatory mediastinal inspection is performed to confirm the echocardiographic findings and, in particular, to identify abnormalities of the aortic arch and coronary arteries. The ascending and descending aorta, head vessels, ductus arteriosus, and PAs are mobilized extensively with care taken to preserve the integrity of the recurrent laryngeal nerve. No attempt is made to dissect the systemic veins. Purse-string sutures are placed in the proximal main pulmonary artery (MPA) and generously around the RA appendage, through which heparin is administered. A previously thawed pulmonary homograft hemiartery patch is trimmed in an extended arrowhead shape and set aside.
After the activated clotting time reaches 300 seconds, the patient is cannulated, with the arterial cannula inserted at the base of the MPA and a single venous cannula inserted in the RA (Fig. 76-2). Cardiopulmonary bypass (CPB) is initiated, and snares are brought down around the branch PAs. The patient is cooled to 18°C over 15 min, during which time any remaining dissection is performed. A side-biting clamp is placed on the innominate artery, and a Gore-Tex graft (usually 4.0 mm patients over 3.2 kg) is anastomosed in an end-to-side fashion to the artery; this can be done either while cooling or before commencing cardiopulmonary bypass. The clamp is removed, and flow is assessed. If blood does not flow easily out of the open shunt, the anastomosis should be revised. A large hemoclip is placed to temporarily occlude the shunt. An alternative source of pulmonary blood flow is a 5.0-mm RV–PA shunt described initially by Norwood and repopularized by Sano. Some institutions have reported that this technique provides a smoother postoperative course and it has gained wide popularity.31,32
Figure 76-2
Incisions for hypoplastic left heart syndrome first-stage palliation. Cannulation is performed with an arterial cannula in the base of the main pulmonary artery and a single venous cannula through a generous purse string around the right atrial appendage. Subsequent incisions, as described in the text, are indicated by the dotted lines. The patch for aortic augmentation and completion of the aortopulmonary anastomosis is fashioned from a pulmonary homograft (insert). (Reprinted with permission from Gruber P, Spray T, et al. Hypoplastic left heart syndrome. In: Kaiser LK, Kron IL (eds). Mastery of Cardiothoracic Surgery, 2nd ed. Philadelphia: Lippincott Williams & Wilkins, 2007:938.)
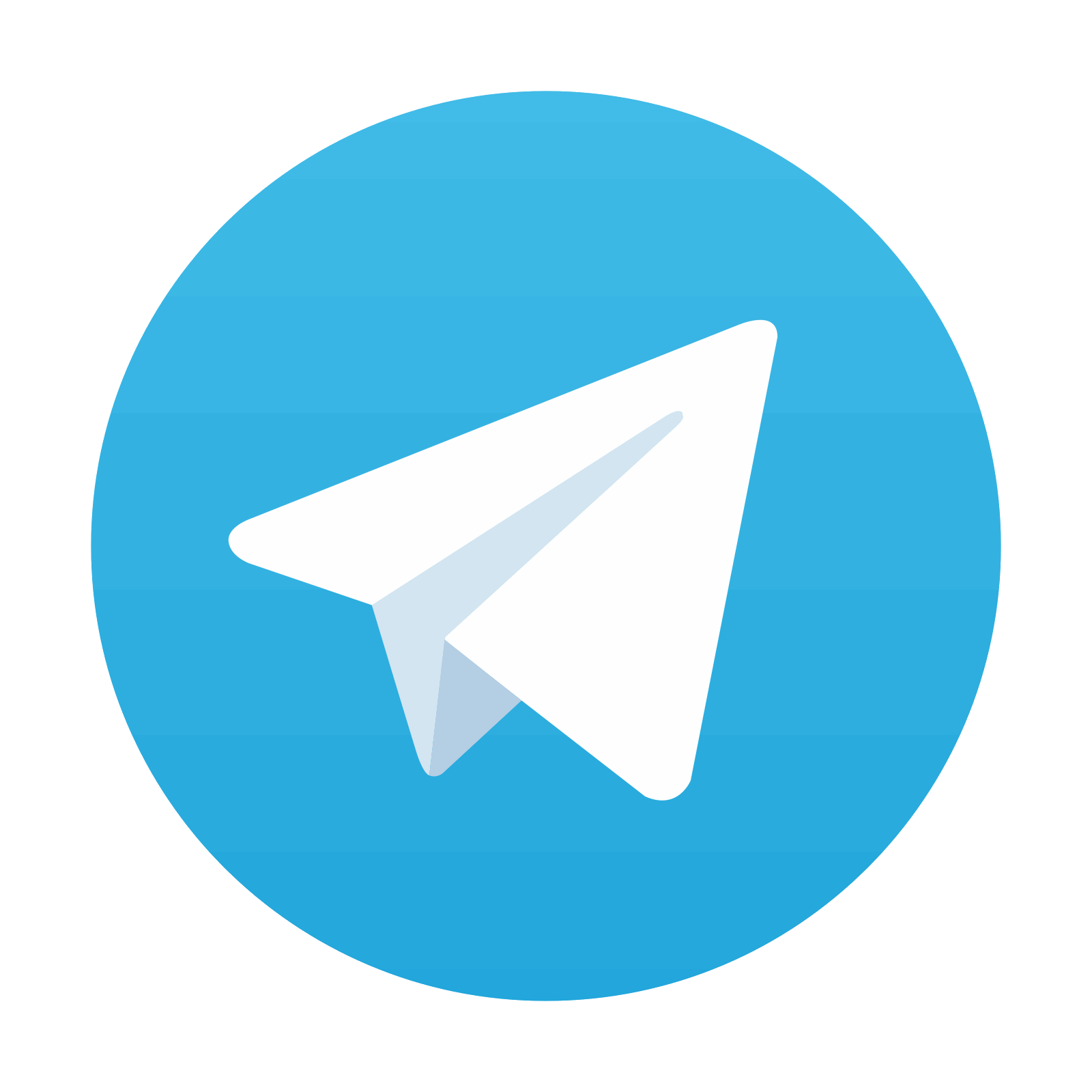
Stay updated, free articles. Join our Telegram channel

Full access? Get Clinical Tree
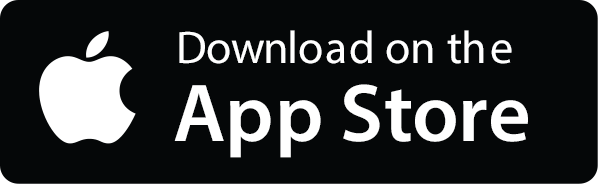
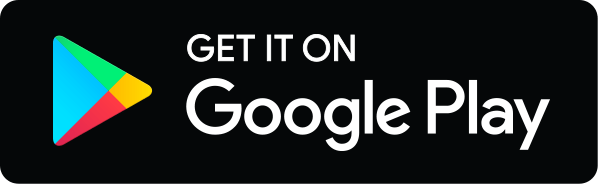
