Hepatorenal syndrome (HRS) is a potentially reversible clinical syndrome that occurs in patients with cirrhosis, ascites and liver failure, as well as in patients with acute liver failure or alcoholic hepatitis. It is characterized by impaired renal function, marked alterations in cardiovascular function and overactivity of the sympathetic nervous (SNS) and renin–angiotensin–aldosterone systems.
The incidence of functional renal failure including HRS in nonazotemic patients with cirrhosis after the onset of ascites is estimated to be 23.6% at 1 year and 42% by 5 years. Older age, higher Child–Pugh score, and higher baseline creatinine are strong predictors for the development functional renal failure including HRS, reflecting that a longer duration of disease, and more severe liver and renal dysfunction are strong risk factors for the development of HRS.
The diagnosis of HRS must be based on excluding other causes of acute kidney injury, because there are no specific tests for the syndrome. The HRS is diagnosed by a serum creatinine higher than 1.5 mg/dL (133 μmol/L) after the exclusion of reversible functional renal failure with volume expansion using albumin at a dose of 1 g/kg body weight (maximum 100 g/d), and withdrawal of diuretic therapy for at least 2 days. The diagnostic criteria of HRS were updated by the International Ascites Club in 2007 ( Box 1 ).
Cirrhosis with ascites.
Serum creatinine >133 μmol/L (1.5 mg/dL).
No improvement of serum creatinine (decrease to a level of ≤133 μmol/L) after ≥2 days with diuretic withdrawal and volume expansion with albumin. The recommended dose of albumin is 1 g/kg of body weight per day up to a maximum of 100 g/d.
Absence of shock.
No current or recent treatment with nephrotoxic drugs.
Absence of parenchymal kidney disease as indicated by proteinuria >500 mg/d, microhematuria (>50 red blood cells per high power field), and/or abnormal renal ultrasonography.
Clinically, there are 2 types of HRS, each with different clinical presentation and different prognostic implications. Type 1 HRS (HRS-1) is a rapidly progressive acute renal failure that occurs in cirrhosis and ascites either spontaneously or in the context of various precipitating factors ( Box 2 ). HRS-1 is diagnosed when the serum creatinine doubles from baseline to a level higher than 2.5 mg/dL (221 μmol/L) in less than 2 weeks. The patient is usually very ill with jaundice and marked coagulopathy. If untreated, median survival is several days. In contrast, type 2 HRS (HRS-2) occurs in patients with cirrhosis and refractory ascites, and the renal function slowly deteriorates over the course of weeks to months as reflected by a chronic, slowly progressive rise in serum creatinine eventually reaching 1.5 mg/dL (133 μmol/L). Because the major clinical problem in patients with HRS-2 is refractory ascites, they are usually less ill with lesser degrees of liver dysfunction. Their prognosis is therefore slightly better than patients with HRS-1 with median survival of several weeks to months.
Spontaneous bacterial peritonitis.
Other bacterial infections.
Intravascular volume depletion: Overly rapid diuresis, excess vomiting, gastrointestinal bleeding.
Large volume paracentesis without adequate intravascular volume replacement.
Nephrotoxic drugs including radiocontrast dye.
Surgical jaundice.
Recent recognition that serum creatinine may underestimate the severity of renal dysfunction has led to the proposal to diagnose renal dysfunction in cirrhosis with lower levels of serum creatinine than traditionally recognized ( Table 1 ). This is because even smaller rises of serum creatinine have been associated with poorer prognosis both in patients with cirrhosis and ascites and in those without underlying liver disease. If accepted, this will allow patients with cirrhosis and renal dysfunction to be treated at an earlier stage of renal impairment, potentially improving their overall prognosis.
Diagnosis | Definition |
---|---|
Acute kidney injury | A rise in serum creatinine of ≥50% from baseline, or a rise of serum creatinine by ≥0.3 mg/dL (≥26.4 μmol/L) in <48 hours. HRS type I is a specific form of acute kidney injury. |
Chronic kidney disease | Glomerular filtration rate of <60 mL/min for >3 mos calculated using the MDRD6 formula. HRS type II is a specific form of chronic kidney disease. |
Acute-on-chronic kidney disease | Rise in serum creatinine of ≥50% from baseline or a rise of serum creatinine by ≥0.3 mg/dL (≥26.4 μmol/L) in <48 hours in a patient with cirrhosis whose glomerular filtration rate is <60 mL/min for >3 mos calculated using the MDRD6 formula. |
Pathogenesis
The pathogenesis of HRS is complex, with several factors contributing to the gradual deterioration in renal function as cirrhosis advances, leading to the development of HRS-2 ( Fig. 1 ). Any acute event that perturbs the systemic and renal hemodynamics can lead to a rapid decline in renal function, precipitating HRS-1. This can occur either in a patient with normal baseline renal function, or superimposed on preexisting HRS-2. The following is a summary of the major contributing factors to the development of HRS in cirrhosis.
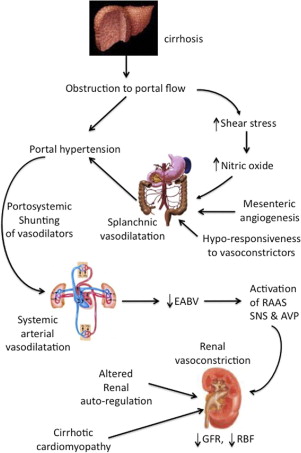
Splanchnic and Systemic Arterial Vasodilatation
The development of cirrhosis is accompanied by the distortion of liver architecture, leading to obstruction to portal flow. As a result, increased shear stress on splanchnic vessel walls lead to increased production of various vasodilators; the most potent is nitric oxide. This, together with development of mesenteric angiogenesis and decreased responsiveness to vasoconstrictors, leads to the development of splanchnic vasodilatation, with a consequent increase in portal venous blood flow. Splanchnic vasodilatation also means that there is pooling of blood volume in the splanchnic circulation. Both the increased portal blood flow and the obstruction to portal flow contribute to the development of portal hypertension. The opening of portosystemic shunts as a result of the portal hypertension means that some vasodilators are channeled from the splanchnic to the systemic circulation, causing systemic vasodilatation. The physiologic response is one of increased cardiac output, together with an increase in total blood volume through renal sodium retention to maintain hemodynamic stability, and a hyperdynamic circulation develops. However, the maldistribution of part of the total blood volume to the splanchnic circulation means that there is effectively a subtraction of volume from the systemic circulation, despite no actual loss of total blood volume, akin to a splanchnic steal syndrome. This is known as a reduction of the effective arterial blood volume (EABV).
Hemodynamic Response to a Reduction in EABV
A reduced EABV results in the compensatory activation of various vasoconstrictor systems, including the SNS, renin–angiotensin–aldosterone system, and arginine vasopressin. In addition, reduced EABV results in decreased renal perfusion pressure, leading to reduced blood flow with consequent reduction in the glomerular filtration rate (GFR). Initially, there is increased production of renal vasodilators such as prostaglandins and kallikrein; however, these soon become exhausted, resulting in an imbalance favoring renal vasoconstriction. This renal hypoperfusion further increases the production of various intrarenal vasoconstrictors, including angiotensin II and endothelin, causing further deterioration of renal hemodynamics and renal function. Endothelin can cause mesangial constriction, further compromising the GFR.
Altered Renal Autoregulation
Renal autoregulation is the process whereby regulatory mechanisms ensure that the kidneys receive a relatively constant blood supply regardless of fluctuations in blood pressure. Below a critical threshold of 65 mmHg, renal blood flow decreases in proportion to renal perfusion pressure that, in turn, depends on mean arterial pressure. When a patient progresses from pre-ascitic cirrhosis to the stages of diuretic-responsive, then diuretic-refractory ascites and eventually HRS, there is a progressive rightward shift of the renal autoregulation curve related to increasing SNS activity. That is, for every given renal perfusion pressure, there is a gradual reduction of renal blood flow as liver disease advances. Therefore, the patient with cirrhosis is poised to develop renal failure simply because of the presence of advanced cirrhosis.
Cardiac Dysfunction in Cirrhosis
The hyperdynamic, high cardiac output state in decompensated cirrhosis means that these patients are encroaching on their cardiac reserve, and further reductions in systemic vascular resistance may not be met with further increases in cardiac output, with consequent fall in blood pressure. Failure to maintain blood pressure further compromises renal perfusion. In advanced cirrhosis, a relative inability to increase cardiac output during stress or systolic incompetence, part of a syndrome known as cirrhotic cardiomyopathy, may be a risk factor for the development of the HRS. For example, cirrhotic patients who develop bacterial infections such as spontaneous bacterial peritonitis have further worsening of their arterial vasodilatation as a result of increased cytokine production from the bacteria. Those who went on to develop the HRS had significantly lower cardiac output at infection resolution when compared with baseline, when compared with those who did not develop the HRS at the time of infection resolution. Recently, cardiac systolic dysfunction in cirrhotics has been found to be associated with increased risk of developing renal dysfunction and poorer survival. This has been confirmed in a further study indicating a correlation between low cardiac output, low mean arterial pressure, and low renal blood flow.
A pathophysiologic basis for using vasoconstrictors in HRS
The rationale for using vasoconstrictors in HRS is to reduce the extent of arterial vasodilatation in cirrhosis, thereby reducing the mismatch between circulatory capacitance and intravascular volume within it, with an overall improved EABV. This attenuates the activation of the various vasoconstrictor systems, leading to an improved renal perfusion pressure, and hence an improved GFR. Currently, there are 3 classes of vasoconstrictors that have been used in the management of HRS.
- •
Vasopressin analogs: Compounds such as ornipressin and vasopressin bind to the V1 receptors of the vascular smooth muscle cells to cause vasoconstriction in both the systemic and in the splanchnic circulations. The latter gives the added beneficial effects of reducing portal inflow, as well as reducing the extent of portal systemic shunting ; terlipressin, another vasopressin analog, has also been shown to dilate intrahepatic vessels, thereby reducing intrahepatic resistance to portal inflow. The overall result is a reduction in portal pressure, which may have a direct effect on improving renal function.
- •
α-Adrenergic receptor agonists: These include norepinephrine (NE) and midodrine. These act by binding to the α-1-adrenergic receptors on the vascular smooth muscle cells, which in turn leads to an increase in the intracellular calcium, thereby causing smooth muscle cell contraction and vasoconstriction.
- •
Octreotide: This is a somatostatin analog. It mediates its vasoconstrictive effects by inhibiting the release of glucagon and other vasodilatory peptides. It has been shown to have a vasoconstrictive effect in both the splanchnic and systemic circulations. Its portal hypotensive effects, however, are very short lived.
To date, most of the studies using vasoconstrictor therapy have concentrated on patients with HRS-1, because they have the most disturbed hemodynamics that can derive the most benefits from vasoconstrictor therapy.
A pathophysiologic basis for using vasoconstrictors in HRS
The rationale for using vasoconstrictors in HRS is to reduce the extent of arterial vasodilatation in cirrhosis, thereby reducing the mismatch between circulatory capacitance and intravascular volume within it, with an overall improved EABV. This attenuates the activation of the various vasoconstrictor systems, leading to an improved renal perfusion pressure, and hence an improved GFR. Currently, there are 3 classes of vasoconstrictors that have been used in the management of HRS.
- •
Vasopressin analogs: Compounds such as ornipressin and vasopressin bind to the V1 receptors of the vascular smooth muscle cells to cause vasoconstriction in both the systemic and in the splanchnic circulations. The latter gives the added beneficial effects of reducing portal inflow, as well as reducing the extent of portal systemic shunting ; terlipressin, another vasopressin analog, has also been shown to dilate intrahepatic vessels, thereby reducing intrahepatic resistance to portal inflow. The overall result is a reduction in portal pressure, which may have a direct effect on improving renal function.
- •
α-Adrenergic receptor agonists: These include norepinephrine (NE) and midodrine. These act by binding to the α-1-adrenergic receptors on the vascular smooth muscle cells, which in turn leads to an increase in the intracellular calcium, thereby causing smooth muscle cell contraction and vasoconstriction.
- •
Octreotide: This is a somatostatin analog. It mediates its vasoconstrictive effects by inhibiting the release of glucagon and other vasodilatory peptides. It has been shown to have a vasoconstrictive effect in both the splanchnic and systemic circulations. Its portal hypotensive effects, however, are very short lived.
To date, most of the studies using vasoconstrictor therapy have concentrated on patients with HRS-1, because they have the most disturbed hemodynamics that can derive the most benefits from vasoconstrictor therapy.
The vasoconstrictors
Vasopressin Analogs
The proof-of-concept studies using a vasopressin analog in the management of HRS-1 employed ornipressin. Complete response defined as either serum creatinine falling to less than 1.5 mg/dL or doubling of creatinine clearance to greater than 40 mL/min was observed in 57% to 75% patients with HRS-1. Median survival was prolonged to several months. However, because of the development of severe adverse events of an ischemic nature, ornipressin is not generally recommended for patients with HRS.
One of the most studied vasopressin analogs in HRS is terlipressin. Because terlipressin is a pro-drug, the active metabolite, lysine-vasopressin, is gradually released over several hours, thereby avoiding many of the ischemic side effects without any compromise of its potency. Its longer half-life also allows for more convenient intermittent intravenous dosing. There is no standardized dosing schedule for terlipressin administration because of the lack of dose-finding studies. Terlipressin is generally started at a dose of 1 mg every 4 to 6 hours and increased to a maximum of 2 mg every 4 to 6 hours if there is no reduction in serum creatinine of at least 25% compared with the baseline value on day 3 of therapy. Treatment is maintained until the serum creatinine has decreased below 1.5 mg/dL (133 μmol/L). Response to therapy is characterized by a slowly progressive reduction in serum creatinine to below 1.5 mg/dL (133 μmol/L), and an increase in mean arterial pressure, urine volume, and serum sodium concentration. Median time to response is 14 days and the response time is usually dependent on the pretreatment serum creatinine level, being shorter in patients with lower baseline serum creatinine levels. Recurrence after withdrawal of therapy is uncommon and retreatment with terlipressin is generally effective. It is important to emphasize that most studies excluded patients with known severe cardiovascular or ischemic conditions or patients with ongoing sepsis. In most studies, terlipressin was given in combination with albumin (1 g/kg of body weight on day 1 followed by 40 g/d) to improve the efficacy of treatment on circulatory function.
Several small studies involving a total of 46 patients with HRS examined the effects of terlipressin, with or without albumin, on systemic hemodynamics and renal function. Terlipressin, given at an initial dose of 0.5 to 1.0 mg every 4 to 6 hours, and titrating upward to 2.0 every 4 to 6 hours significantly improved mean arterial pressure by 13% to 28%. Serum creatinine reduced by at least 50% and the GFR doubled. In the 2 studies that measured plasma renin activity, the levels were reduced by at least 80%, suggesting that terlipressin improved the EABV and reduced the activation of the systemic vasoconstrictor systems. Ischemic side effects were significantly less with terlipressin than with ornipressin.
Two larger, randomized, controlled trials were subsequently published on the use of terlipressin for the treatment of HRS-1. In the first, Sanyal and the Terlipressin Study Group evaluated the safety and efficacy of terlipressin plus albumin versus albumin alone in 112 patients with HRS-1 in a multinational study. Patients in the terlipressin group achieved significant improvement in serum creatinine ( P <.009; Fig. 2 A ), mean day-14 Model of End-stage Liver Disease score (−4.1 vs −1.7; P <.008) and HRS reversal (44% vs 9%; P <.008) compared with the placebo group. However, there was no difference in 6-month overall survival or transplant-free survival. More patients in the terlipressin group experienced serious adverse cardiac events such as nonfatal myocardial infarction, nonsustained supraventricular tachycardia, and arrhythmias (10 vs 4 patients). In the second study, Martín-Llahí and The Terlipressin and Albumin for Hepatorenal Syndrome Investigators compared terlipressin at 1 to 2 mg every 4 hours plus albumin to albumin alone in both HRS-1 and HRS-2 patients (n = 46). Similarly, in this trial, the terlipressin group experienced a significantly higher rate of improved renal function (by 0.7 mg/dL vs no change with placebo; Fig. 2 B ). Survival at 3 months, however, was not different. Once again, there was an increased rate of cardiovascular complications in the terlipressin group including myocardial ischemia, intestinal ischemia, arrhythmias, and volume overload (5 vs 1 patient).
The improvement in renal function after the administration of terlipressin in HRS-1 can be explained by its physiologic actions. Its vasoconstrictive effects on the systemic circulation can lead to an improvement in systemic hemodynamics, associated with an amelioration of the hyperdynamic circulation in cirrhosis. The EABV becomes better filled, as reflected by a significant reduction in activities of the renin–angiotensin–aldosterone system and SNS. This in turn, leads to an improvement in GFR, and renal sodium excretion.
There is preliminary evidence that when terlipressin is given as a continuous infusion rather than as boluses in the treatment of HRS-1, the same efficacy can be achieved with a lower total daily dose and with fewer side effects. Pharmacodynamic studies have shown that continuous infusion of terlipressin provides a more sustained portal pressure-lowering effect than bolus injections, thereby explaining the beneficial effects of a terlipressin infusion over bolus injections.
Although these studies have included a few patients with HRS-2, the data are difficult to abstract to determine the effects of terlipressin on renal function in patients with HRS-2. Studies specifically designed for HRS-2 patients are scant. However, given the fact that the pathophysiology of HRS-2 is similar to that of HRS-1, especially in terms of the splanchnic and systemic hemodynamic changes, there is every reason to believe that terlipressin should also work in patients with HRS-2. In a small study that included 11 patients with HRS-2, terlipressin given at a dose of 1 mg every 4 hours for at least 7 days resulted in a reduction in serum creatinine in 73% of patients, with 88% of the responders achieving a serum creatinine of less than 1.5 mg/dL (133 μmol/L). Seven of the responders then went on to receive a transjugular intrahepatic portosystemic shunt (TIPS), making it difficult to assess whether HRS-2 recurred after completion of terlipressin therapy or not.
α-Adrenergic Receptor Agonists
Midodrine
The most widely used α-adrenergic receptor agonist is midodrine, a systemic vasoconstrictor that has been approved for the treatment of postural hypotension. The acute effects of midodrine on renal function was first assessed in 25 cirrhotic patients with ascites (17 patients without HRS and 8 with HRS-2). In patients without HRS, midodrine was able to improve systemic and renal hemodynamics, increase urinary sodium excretion, associated with a decrease in plasma renin activity, and arginine vasopressin, as well as serum nitrite and nitrate levels. However, in the 8 patients with HRS, midodrine did not have any significant effects on renal function, or urinary sodium excretion.
However, the combination of midodrine and octreotide in patients with HRS-1 seems to have the same beneficial effects on renal function as midodrine alone in patients without HRS. Three studies, totaling 79 patients reported HRS reversal as defined by a serum creatinine of less than 1.5 mg/dL (133 μmol/L) in 49% of patients when the combination was given for a median period of 17 days. Midodrine has been administered orally at an initial dose of 5 to 10 mg 3 times per day, and octreotide either subcutaneously at an initial dose of 100 μg 3 times daily, or as an intravenous infusion at an hourly dose of 25 μg/h after an initial bolus of 25 μg. If there is no increase in MAP of at least 15 mmHg, the dose of midodrine can be increased up to 15 mg 3 times daily and that of subcutaneous octreotide up to 200 μg 3 times daily. Another retrospective study evaluated the use of 7.5 to 15 mg midodrine combined with subcutaneous octreotide 100 to 200 μg 3 times per day and intravenous albumin 50 to 100 g/d for a mean of 8.4 ± 9.6 days in 75 patients with either HRS-1 (n = 49) or HRS-2 (n = 26). The treatment group was compared with a pre-2001 historical control group of 87 patients with either HRS-1 (n = 53) or HRS-2 (n = 34) who did not receive the drug regimen. During a mean follow-up of almost 4 months, there was a significant improvement of GFR in the treatment group compared with the historical controls (48 vs 34 mL/min; P = .03). Median survival was significantly improved in patients who received the combination therapy for both HRS-1 (40 vs 17 days; P = .007) and HRS-2 patients (>12 months vs 22 days; P = .0004). The percentage of patients who underwent transplantation was increased only for patients with HRS-2 (58% vs 25%; P = .04).
One study found that the dose of midodrine was an important determinant in HRS-1 reversal. When midodrine was given at 15 mg 3 times daily, 88% patients had HRS-1 reversal compared with 33% in those receiving <12.5 mg 3 times daily. Adverse events associated with midodrine use are generally mild and self-limiting and these include diarrhea and tingling without cardiovascular complications. Therefore, the combination of midodrine and octreotide is a alternative in the management of HRS, particularly in countries where terlipressin in unavailable.
NE
NE (at 0.5–3 mg/h) is another α-adrenergic receptor agonist that has been administered to patients with HRS because of its potent vasoconstrictive effects on both the venous and arterial vasculature. However, the number of patients treated with NE is small and no randomized, comparative studies with a control group of patients receiving no vasoconstrictor therapy have been performed to evaluate its efficacy.
The initial uncontrolled pilot study using NE showed a 83% reversal of HRS-1 in 12 patients after a median duration of treatment of 7 days. NE also effectively improved serum sodium concentration, creatinine clearance, urine output, and renal sodium excretion. Systemic hemodynamics as indicated by mean arterial pressure, and fullness of the EABV, as indicated by plasma renin activity, and aldosterone levels also improved.
Two recent studies that were part of small, prospective, open-label, randomized studies of NE versus terlipressin found both vasoconstrictors to be equally effective in the treatment of HRS-1 with similar rates of side effects. One study compared the efficacy of NE versus terlipressin for treating HRS in 22 patients (9 with HRS-1 and 13 with HRS-2). Patients received norepinephrine 0.1 to 0.7 μg/kg per minute plus albumin (n = 10) or terlipressin 1 to 2 mg every 4 hours plus albumin (n = 12) for up to 2 weeks. Reversal of HRS occurred in 70% of patients receiving NE versus 83% of patients receiving terlipressin ( P = NS), indicating NE was not inferior to terlipressin in the management of HRS, although it was a small trial. Relapse after treatment discontinuation occurred in 29% of NE responders versus 60% of terlipressin responders ( P = NS). The cost of NE therapy was significantly lower than that of terlipressin (107 vs 1536 Euros; P <.0001). To evaluate the efficacy of NE for treating patients with HRS-1, a randomized pilot study was conducted using NE infusion starting at 0.5 mg/h with stepwise increases of 0.5 mg/h every 4 hours titrated to the mean arterial pressure plus albumin (20 patients) versus intravenous terlipressin 0.5 to 2 mg every 6 hours plus albumin (20 patients). NE significantly increased MAP (mean, 78.3–93.3 mmHg; P <.01), increased urine output (mean, 479–1278 mL/d; P <.01), and decreased serum creatinine from baseline (mean, 3.3–1.0 mg/dL; P <.01; Fig. 3 ). The renal function improvement between patients treated with NE and those treated with terlipressin was similar. The percentage of patients who responded to therapy was not different between the NE and terlipressin groups (50% vs 40%; P = .741), and no difference was noted in cumulative survival between groups (11.6 vs 12.6 days; P = .452).
