© Springer International Publishing Switzerland 2017
Kia Saeian and Reza Shaker (eds.)Liver Disordershttps://doi.org/10.1007/978-3-319-30103-7_3333. Hepatopulmonary Syndrome
(1)
Division of Pulmonary & Critical Care, Medical College of Wisconsin, Milwaukee, WI, USA
(2)
Department of Critical Care Medicine, Aurora St. Luke’s Medical Center, Milwaukee, WI, USA
Keywords
HypoxemiaCirrhosisLiver diseaseIntrapulmonary vascular dilatationsPlatypneaOrthodeoxiaCyanosisHepatopulmonary syndromePatient-Level Questions
- 1.
What is hepatopulmonary syndrome and what causes it?
Hepatopulmonary syndrome is a condition that may be present in patients with liver disease. It is characterized by low levels of oxygen in the body. We think that the diseased liver is responsible for changes in the lungs that cause the low oxygen levels. Oxygen enters the body through transfer from the airspaces in the lung to small blood vessels that are a part of the lung circulation. These small blood vessels in the pulmonary circulation are affected by this condition and become enlarged or dilated and are not able to efficiently participate in receiving oxygen from the lung airspaces leading to low oxygen levels in the body
- 2.
What are the consequences of hepatopulmonary syndrome?
The most important consequence of hepatopulmonary syndrome is the low level of oxygen in the body. This may lead to shortness of breath at rest or with exercise. The shortness of breath may become worse on standing up from a supine or sitting position. Frequently patients may have to wear supplemental oxygen to raise the levels of oxygen in their body and alleviate their shortness of breath. The definitive cure for heptaopulmonary syndrome is liver transplantation; however, complete resolution may take several months after liver transplant.
- 3.
How do I know if I have hepatopulmonary syndrome?
The most commonly used method of diagnosing hepatopulmonary syndrome is contrast-enhanced echocardiography. In this test, tiny air bubbles are injected into a peripheral vein while visualizing the heart via echocardiography at the same time. Normally the tiny injected bubbles pass through the right side of the heart and into pulmonary circulation where they are trapped by the small vessels of the lungs. This is because the bubbles are usually larger than the small lung vessels. However, in hepatopulmonary syndrome, the lung vessels are dilated and allow passage of the air bubbles into the left side of the heart. This is seen on echocardiography.
Historical Perspectives
Observations linking chronic liver disease to pulmonary abnormalities first appeared in the medical literature in 1884 when Fluckiger reported cyanosis and finger clubbing in patients with cirrhosis [1]. This was followed by confirmation of arterial hypoxemia and elegant autopsy studies describing widespread vasodilation of the pre-capillary pulmonary arterioles in 13 patients with cirrhosis [2]. Seminal observations from these histopathological studies included normal lung structures (alveoli and connective tissue) and increase in number of vessels along the alveolar wall caused by pre-capillary arteriolar vasodilation. Hepatopulmonary (HPS) syndrome was recognized as a clinical entity when the intellectual leap of intrapulmonary vasodilation causing arterial hypoxemia was made [3].
Epidemiology and Natural History
There remains a lack of large prospective series defining the epidemiology of HPS. Retrospective series mostly from single centers report prevalence ranges between 5 and 32 % [4]. The wide variance in prevalence is likely secondary to variability in cutoffs used to define gas exchange abnormalities. Although most commonly associated with cirrhosis and portal hypertension, HPS has been described in hepatitis without cirrhosis and portal hypertension as well as in noncirrhotic portal hypertension without underlying chronic liver disease [5–8]. Further neither the presence nor the degree of hypoxemia in HPS correlate well with the severity of liver disease as adjudicated by the Child-Pugh’s or Model for End-Stage Liver Disease (MELD) classification [9]. However, the presence of HPS confers an increased risk of mortality when compared to similar patients who do not have HPS [9, 10]. Moreover, survival is markedly worse if the room air partial pressure of oxygen is less than 50 mmHg at the time of diagnosis. Reasons for demise in patients with HPS are usually related to complications of liver disease and are rarely secondary to arterial hypoxemia. Although, the exact biological pathways that lead to the development of HPS need to be elucidated, a recent study found that polymorphisms in candidate genes involved in the regulation of angiogenesis was associated with a higher risk of HPS [11].
Pathophysiology
The main pathological features of HPS are gross dilatation of the pulmonary pre-capillary and capillary vessels as well as an increase in the absolute number of dilated vessels [2]. Additionally, some pleural and pulmonary arteriovenous communications (true shunts) may also be observed. Reduced tone of the pulmonary vasculature and inhibition of hypoxic pulmonary vasoconstriction are some other findings that characterize HPS [12]. The arterial hypoxemia seen in HPS is secondary to intrapulmonary vascular dilatations (IPVD) resulting in ventilation-perfusion inequality [13, 14]. Blood flow or lung perfusion is increased while ventilation remains preserved. The ventilation perfusion mismatch may be exacerbated by true shunting of deoxygenated blood through pulmonary arteriovenous communications [13, 14]. The severity of arterial hypoxemia is related to the extent of the intrapulmonary mechanisms in play.
Animal Models
A rat common bile duct ligation (CBDL) model has features akin to human HPS [15]. In this model, proliferating cholangiocytes secrete endothelin-1 (ET-1), which binds to upregulated endothelin B receptors [16]. This enhances nitric oxide (NO) production through endothelial nitric oxide synthetase (NOS) [17]. Further an increase in pulmonary monocytes is observed which enhance nitric oxide and carbon monoxide production through inducible NOS and heme-oxygenase-1 respectively [18]. Moreover, an increase in blood levels of vascular endothelial growth factor is observed (VEGF) [19]. These mechanisms in concert are responsible for the development of IPVD and de novo pulmonary angiogenesis resulting in hypoxemia [20]. The anti-angiogenesis factor sorafenib has shown to be associated with improvements in oxygenation in CBDL rats with HPS [21].
Human Disease
Besides autopsy studies demonstrating the presence of vascular dilatations and shunts, the understanding of molecular mechanisms leading to HPS in humans is limited. Several lines of indirect evidence implicate NO and angiogenesis as likely contributors. Exhaled nitric oxide levels in patients with HPS are higher compared to those without HPS and these levels normalize after liver transplantation [22, 23]. Polymorphisms in genes associated with angiogenesis have now been linked to the presence of HPS [11]. Often, hypoxemia post liver transplantation takes several months to resolve suggesting that there is perhaps remodeling of the pulmonary vasculature, lending credence to the theory of angiogenesis. In one study, ET-1 levels in the hepatic vein were higher in patients with HPS and IPVD compared to patients without IPVD and blood ET-1 levels corresponded with the degree of bile duct proliferation in liver biopsy specimens [24]. It is, however, important to understand that a causal relationship between the aforementioned mechanisms and hypoxemia in HPS has yet to be established.
Diagnostic Criteria
Formal accepted diagnostic criteria for HPS comprise of three components—(1) documentation of impaired oxygenation, (2) presence of intrapulmonary vasodilation (IPVD), and (3) evidence of cirrhosis or portal hypertension [25].
Because patients with liver disease hyperventilate and this phenomenon may increase the arterial tension of oxygen, milder forms of HPS may be missed if hypoxemia is used as the sole criterion. It is therefore important to use the sensitive alveolar-arterial (A-a) oxygen gradient to document defects in oxygenation for milder forms. A cut-off value of 15 mmHg for younger patients and 20 mmHg for patients aged 64 or older while breathing ambient air (21 % oxygen) is agreed upon as being diagnostic of impaired oxygenation in the right clinical setting [25].
The severity of the oxygenation defect is associated with survival and is a guide to the timing and risks of liver transplantation. Therefore the technique of alveolar-arterial gradient for the diagnosis of impaired oxygenation in HPS is used to classify patients into the following 4 categories of severity [25]
- (a)
Mild disease—PaO2 > 80 mmHg
- (b)
Moderate disease—PaO2 >60 to <80 mmHg
- (c)
Severe disease—PaO2 >50 to <60 mmHg
- (d)
Very severe disease—PaO2 < 50 mmHg (or <300 mmHg while breathing 100 % oxygen)
Demonstration of IPVD
There are two commonly used tests to demonstrate the presence of IPVD—contrast-enhanced echocardiography (CEE) [26] and nuclear medicine lung perfusion scan using technetium-labeled macro-aggregates of albumin [27].
CEE is the most sensitive test and is more commonly used. Agitated saline which creates microbubbles is infused into a peripheral vein causing opacification of the right atrium and ventricles. Normally as the microbubbles move through the pulmonary circulation they are trapped by the pulmonary capillaries and should not appear on the left side of the heart. Appearance of microbubbles in the left atrium within 3–6 cardiac cycles is indicative of passage through an abnormally dilated pulmonary vascular bed. Earlier appearance of microbubbles on the left side (<3 cardiac cycles) indicates a right to left intra-cardiac shunt. Almost 60 % of patients referred for liver transplantation have a positive CEE, but only half meet the diagnostic criteria for HPS (IPVD with normal A-a gradient). The clinical significance of these subclinical IPVD is currently not known [4, 28].
Using the lung perfusion approach involves injecting macro-aggregates of albumin into the peripheral venous circulation. These macro-aggregates should not pass into systemic circulation as they are trapped by the pulmonary vasculature. Appearance in the systemic circulation as measured by scintigraphy is indicative of either IPVD or a right to left shunt at the cardiac or pulmonary level. A shunt fraction (brain/total body macro-aggregates) of greater than 6 % denotes a positive result. This test is less sensitive than CEE and maybe useful in the determining the degree of contribution of IPVD to hypoxemia in patients with coexisting lung disease and other causes of hypoxemia.
Pulmonary angiography is another modality that may be used but is not generally recommended. It is reserved for patients whose hypoxemia is poorly responsive to 100 % oxygen and may demonstrate true arteriovenous (AV) communications that may be amenable to coil embolization. The appearance of the pulmonary vasculature in HPS with pulmonary angiography has two patterns—Type I HPS characterized by pre-capillary dilatations without AV communications and Type 2 HPS characterized by true localized pulmonary AV communications. Type 2 HPS is poorly responsive to oxygen therapy and consideration should be given to coil embolization in these cases [29, 30].
Clinical Manifestations and Laboratory Testing
In addition to the general manifestations of chronic liver disease such as spider nevi, dyspnea is the most common complaint of patients with HPS, occurring in nearly 70 % patients [31]. However, this complaint is nonspecific and may result from a variety of coexisting conditions. These conditions may be complications of chronic liver disease and portal hypertension such as ascites and hepatic hydrothorax or independent associated entities such as volume overload, muscle wasting, anemia, and intrinsic lung disease. HPS may be concurrent with these pleural and pulmonary complications of advanced liver disease and therefore clinical judgment may be necessary in several cases in the attribution of the degree of hypoxemia to HPS versus one of the concomitant conditions.
Physical examination findings are nonspecific as well and include the presence of digital clubbing, cyanosis , and spider nevi. Two phenomena associated with HPS are orthodeoxia (hypoxemia that worsens in the upright position) and platypnea (increased shortness of breath in the seated versus supine position). These occur because change in position from supine to upright result in redistribution of pulmonary blood flow to lung bases, preferentially perfusing dilated vasculature and worsening ventilation perfusion defects [32]. Although classically described in HPS, both platypnea and orthodeoxia are not sensitive indicators of disease [28].
The single most consistent laboratory abnormality is a decrease in the diffusing capacity for carbon monoxide [28]. However, this is not specific and unlike other gas exchange indexes may not normalize after liver transplantation [33, 34]. The chest radiograph may demonstrate an interstitial reticular pattern in the lower lung zones reflecting the presence of IPVD. Often chest imaging and pulmonary function tests may be representative of other conditions that coexist with HPS and contribute to hypoxemia. In these cases demonstration of IVPD via CEE and judgment is essential to attribute clinical manifestations to HPS [35].
Treatment
Pharmacological Therapies
Patients with significant degrees of hypoxemia at rest or with exercise are universally treated with supplemental oxygen therapy. However, oxygen therapy has never been demonstrated to reliably improve dyspnea or improve quality of life in patients with HPS. Although a variety of pharmacological agents targeting putative biological pathways have been tested in uncontrolled studies, effects have been variable and none have shown to dependably improve oxygenation. These interventions include pentoxifylline [36], mycophenolate mofetil [37], somatostatin [29], methylene blue [38], almitrine [39], cyclo-oxygenase inhibitors [40], antibiotics [41], propranolol [42] and garlic [43, 44].
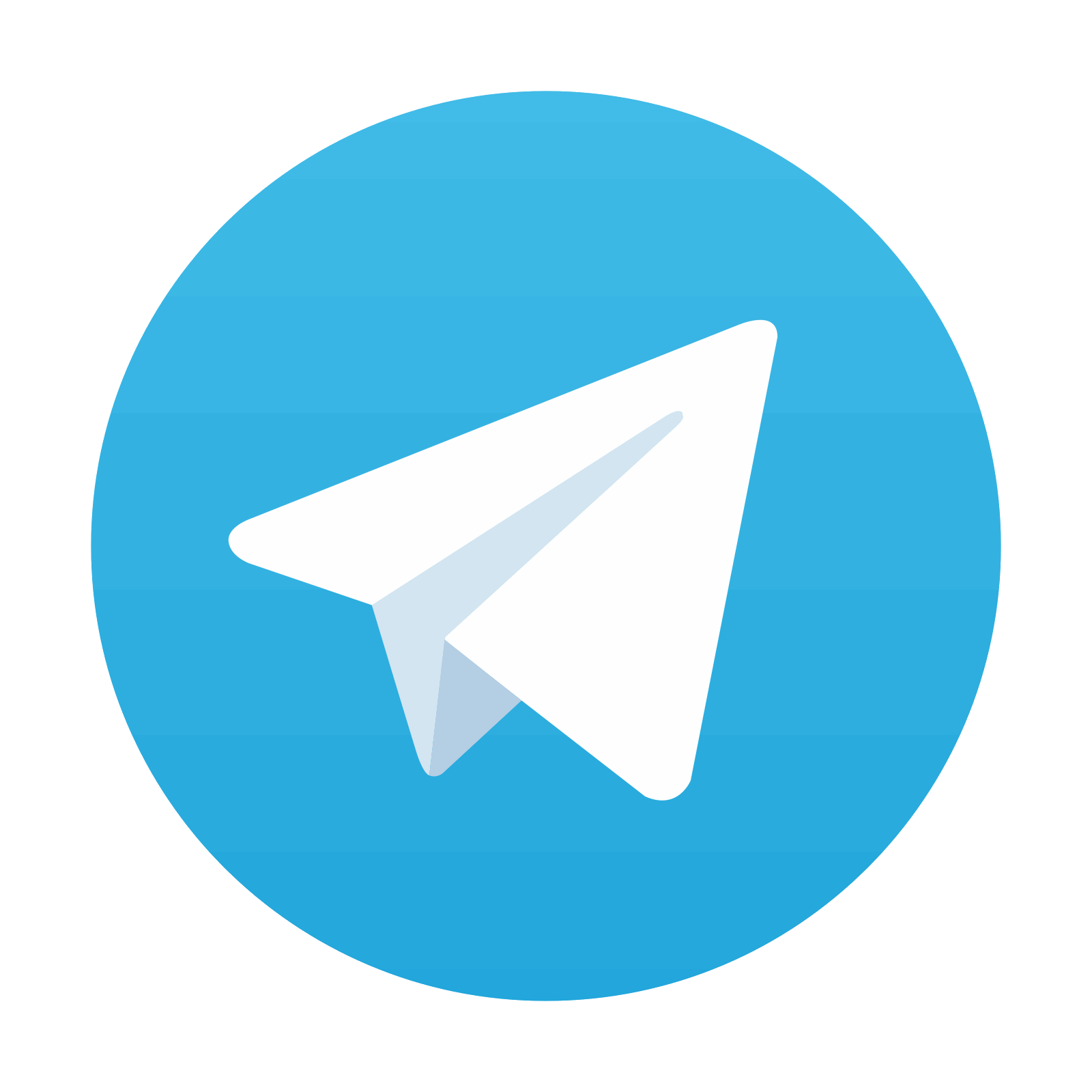
Stay updated, free articles. Join our Telegram channel

Full access? Get Clinical Tree
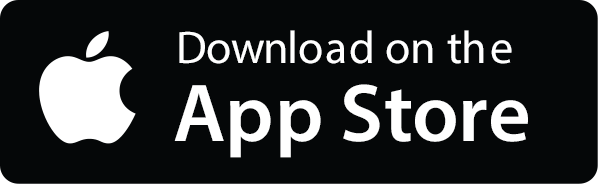
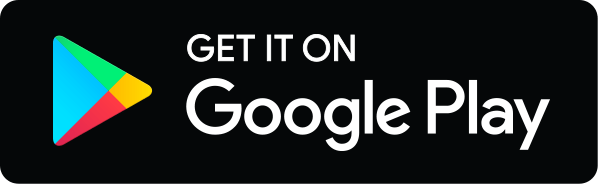