Abstract
Hemodialysis patients are at increased risk of infections, which are common adverse events among this patient population. We review factors contributing to infections among hemodialysis patients and epidemiology of common infections and outbreaks, including bloodstream infections, vascular access infections, and infections caused by bloodborne pathogens. Recommendations for prevention are discussed with emphasis on essential infection control practices for hemodialysis settings.
Keywords
hemodialysis, infections, infection control
Outline
Introduction, 389
Factors Contributing to Infections Among Hemodialysis Patients, 389
Microbial Contamination of Water, 390
Distribution Systems, 391
Hemodialysis Machines, Effluent, and Environmental Surfaces, 392
Hemodialyzer Reuse, 392
High-Flux Dialysis and Bicarbonate Dialysate, 397
Disinfection of Hemodialysis Systems, 397
Dialysis-Associated Pyrogenic Reactions, 398
Disinfection, Sterilization, and Environmental Cleaning in Dialysis Facilities, 399
Bloodstream Infections and Other Infections, 400
Vascular Access Infections, 401
Etiology and Prevention of Bloodstream Infection, 401
Respiratory Infections, 403
Antimicrobial-Resistant Bacteria, 403
Hepatitis C Virus, 404
Epidemiology, 405
Screening and Diagnostic Tests, 405
Prevention of Hepatitis C Virus Transmission, 405
Hepatitis B Virus, 406
Epidemiology, 406
Screening and Diagnostic Tests, 407
Prevention of Hepatitis B Virus Transmission, 407
Hepatitis Delta Virus, 408
Human Immunodeficiency Virus Infection, 408
Other Emerging Infections, 408
Summary of Recommendations and Future Directions, 408
Introduction
Of the patients with end-stage renal disease (ESRD) treated by maintenance dialysis in the United States, approximately 90% are on maintenance hemodialysis and 10% are on peritoneal dialysis. Maintenance hemodialysis patients are at higher risk for infection, because uremia is known to make patients with ESRD more susceptible to infectious agents through defects in cellular immunity, neutrophil function, and complement activation. In addition, because the process requires vascular access for long periods in an environment where multiple patients receive hemodialysis concurrently, repeated opportunities exist for transmission of infectious agents. Patient-to-patient transmission of infectious agents, directly or indirectly through contaminated devices, equipment, supplies, injectable medications, environmental surfaces, or hands of healthcare personnel have all been demonstrated. Furthermore, hemodialysis patients require frequent hospitalizations and surgery, which increases their opportunities for exposure and risk for developing healthcare-associated infections. This chapter describes (1) the major infectious diseases that can be acquired in the dialysis center setting, (2) important epidemiological and environmental microbiological considerations, and (3) infection control strategies.
Factors Contributing to Infections Among Hemodialysis Patients
Technical development and clinical use of hemodialysis delivery systems improved dramatically in the late 1960s and early 1970s. However, a number of microbiological parameters were not accounted for in the design of many hemodialysis machines and their respective water supply systems. There are many situations where certain types of gram-negative water bacteria can persist and actively multiply in hemodialysis water supplies and aqueous environments associated with hemodialysis equipment. This can result in massive numbers of gram-negative bacteria, which can directly or indirectly lead to septicemia or endotoxemia. These bacteria can adhere to surfaces and form biofilms (glycocalyces), which are virtually impossible to eradicate. Control strategies are designed not to eradicate bacteria but to reduce their concentration to relatively low levels and to prevent their regrowth.
Although certain genera of gram-negative water bacteria (e.g., Burkholderia, Flavobacterium, Pseudomonas, Ralstonia, Serratia , Stenotrophomonas maltophilia , and Sphingomonas ) are most commonly encountered, virtually any bacterium that can grow in water can be a problem in a hemodialysis unit. Several species of nontuberculous mycobacteria may also contaminate water treatment systems, including Mycobacterium chelonae, M. abscessus, M. fortuitum, M. gordonae, M. mucogenicum, M. scrofulaceum, M. kansasii, M. avium , and M. intracellulare ; these microorganisms do not contain bacterial endotoxin but are comparatively resistant to chemical germicides.
Gram-negative water bacteria can multiply even in water containing relatively small amounts of organic matter, such as water treated by distillation, softening, deionization, or reverse osmosis, reaching levels of 10 5 to 10 7 microorganisms/mL ; these levels are not associated with visible turbidity. When treated water is mixed with dialysis concentrate, the resulting dialysis fluid is a balanced salt solution and growth medium almost as rich in nutrients as conventional nutrient broth. Gram-negative water bacteria growing in dialysis fluids can reach levels of 10 8 to 10 9 microorganisms/mL, producing visible turbidity.
Bacterial growth in water used for hemodialysis depends on the types of water treatment system used, dialysate distribution systems, dialysis machine type, and method of disinfection ( Table 25.1 ). Each component is discussed separately next.
Factors | Comments |
---|---|
Water Supply (Water Source) | |
Groundwater | Contains endotoxin and bacteria |
Surface water | Contains high levels of endotoxin, bacteria, and other organisms |
Water Treatment at the Dialysis Center | |
None | Not Recommended |
Filtration | |
Prefilter | Particulate filter to protect equipment; does not remove microorganisms |
Absolute filter (depth or membrane) | Removes bacteria but unless changed frequently or disinfected, bacteria will accumulate and grow through the filter; acts as a significant reservoir of bacteria and endotoxin |
Granular activated carbon (GAC) | Removes organics and available chlorine or chloramine; significant reservoir of water bacteria and endotoxin |
Water Treatment Devices | |
Ion exchange (softener, deionization) | Softeners and deionizers remove cations and anions, contaminants from source water; significant reservoir for bacteria and endotoxin |
Reverse osmosis (RO) | Removes bacteria, endotoxin, chemicals, and must be cleaned and disinfected; most systems employed for dialysis applications operate under high pressure |
Ultraviolet (UV) germicidal irradiator | Kills most bacteria, but there is no residual; some UV-resistant bacteria can develop |
Ultrafilter | Removes bacteria and endotoxin; operates on normal line pressure; can be positioned distal to storage tank and deionizer; must be disinfected or changed |
Water and Dialysate Distribution System | |
Distribution pipes | |
Size | Oversized diameters and length decrease fluid flow and increases bacterial reservoir in the form of biofilms for both treated water and central delivery systems (bicarbonate concentrate or bicarbonate dialysate) |
Materials | Pipe materials influence bacterial colonization and biofilm formation, as well as what types of chemical disinfectants can be used |
Construction | Rough joints, dead ends, and unused branches can act as bacterial reservoirs |
Elevation | Outlet taps should be located at highest elevation to prevent loss of disinfectant |
Storage tanks | Generally undesirable because of large surface area and can act as a reservoir for water bacteria; a properly designed tank can minimize this risk |
Dialysis Machines | |
Single pass | Disinfectant should have contact time with all parts of the machine that are in contact with treated water or dialysate |
Recirculating single pass, or recirculating batch | Recirculating pumps and machine design allow for massive contamination levels if not properly disinfected; overnight disinfection recommended |
Microbial Contamination of Water
Water used for the production of dialysis fluid must be treated to remove chemical and microbial contaminants. The Association for the Advancement of Medical Instrumentation (AAMI) published guidelines and recommended practices for the chemical and microbial quality of water used to prepare dialysis fluid and reprocess hemodialyzers ( Table 25.2 ). The Centers for Medicare and Medicaid Services (CMS) has incorporated into their ESRD facility conditions for coverage infection control requirements that dialysis facilities need to follow, including water quality standards. Some components of the water treatment system may allow for amplification of water bacteria. For example, ion exchangers such as water softeners and deionizers do not remove endotoxin or microorganisms and provide many sites for significant bacterial multiplication. Granular activated carbon adsorption media (i.e., carbon filters) are used primarily to remove certain organic compounds and available chlorine (free and combined) from water, but they also significantly increase the level of water bacteria, yeast, fungi, and endotoxins.
Microbial Bioburden | Endotoxin | |||
---|---|---|---|---|
Type of Fluid | Maximum Contaminant Level | Action Level | Maximum Contaminant Level | Action Level |
Water for all purposes | 100 CFU/mL | 50 CFU/mL | 0.25 EU/mL | 0.125 EU/mL |
Conventional dialysate | 100 CFU/mL | 50 CFU/mL | 0.5 EU/mL | 0.25 EU/mL |
Ultrapure dialysate | 1 CFU/10 mL | 0.03 EU/mL | ||
Dialysate for infusion ∗ | This online process shall be validated by the manufacturer to produce fluid that is sterile and nonpyrogenic. |
∗ Compliance with a maximum bacterial level of 10 –6 CFU/mL cannot be demonstrated by culturing, but by processes developed by the machine manufacturers.
A variety of filters are marketed to control bacterial contamination of water and dialysis fluids. Most are inadequate, especially if they are not routinely disinfected or frequently changed. Particulate filters, commonly called prefilters , operate by depth filtration and do not remove bacteria or endotoxin. These filters can become colonized with gram-negative water bacteria, resulting in higher levels of bacteria and endotoxin in the filter effluent. Absolute filters, including membrane types, temporarily remove bacteria from passing water. However, some of these filters tend to clog, and gram-negative water bacteria can “grow through” the filter matrix and colonize downstream surfaces of the filters within a few days. Further, absolute filters do not reduce levels of endotoxin in the effluent water. These filters should be changed regularly in accordance with the manufacturer’s directions and disinfected in the same manner and at the same time as the rest of the water distribution system.
Ultraviolet germicidal irradiation (UVGI) is sometimes used to reduce microbial contamination in water, but the use of UVGI has some special considerations. The lamp should be appropriately sized for the flow rate of water passing through the device, and the energy output should be monitored to ensure effectiveness of the lamp. Manufacturers of the lamp may require routine replacement schedule. Some bacterial populations may develop resistance to UVGI. In recirculating dialysis distribution systems, repeated exposure to UVGI are used to ensure adequate disinfection; however, this approach allows for progressive removal of sensitive microorganisms and selection of UVGI-resistant organisms. In addition, bacterial endotoxins are not affected.
Reverse osmosis (RO) is an effective water treatment modality that is used in more than 97% of US hemodialysis centers. RO possesses the singular advantage of being able to remove a variety of substances, including microorganisms and endotoxins, from supply water based primarily on particle size and adsorption to the membrane. However, low numbers of gram-negative and acid-fast organisms may penetrate the membrane or by other means (leaks around seals), and colonize downstream portions of the water distribution system. Consequently, the RO unit must be disinfected routinely.
We recommend a water treatment system that produces chemically adequate water while avoiding high levels of microbial contamination. The components in a typical water system should include (1) prefilters, (2) a water softener, (3) carbon adsorption tanks (at least two in series), (4) a particulate filter (to protect the reverse osmosis membrane), and (5) an RO unit. If one includes a deionization unit as a polisher (post–reverse osmosis unit) and a storage tank, the final component should be an ultrafilter to remove microorganisms and endotoxin. As the incoming tap water passes through the system components, it becomes more chemically pure, but the level of microbial contamination increases, which is why ultrafiltration and RO are important. Additional components or processes may be included in the pretreatment chain (see Table 25.1 ) depending on the pH, potable water disinfectant, and chemical quality of the incoming municipal water. If the system is adequately disinfected and properly maintained, the microbial content of water should be well within the recommended limits.
Distribution Systems
Water that has passed through the water distribution system (product water) is then distributed to individual dialysis machines where it is combined with dialysate concentrates and to a reprocessing area if a facility reprocesses hemodialyzers. It may also be combined with concentrates at a central location where the resulting dialysis fluid is supplied to the individual machines. Plastic pipe (most often polyvinyl chloride) is then used to distribute water, or dialysis fluids to the dialysis machines. Distribution systems should include the use of a loop-based system and no dead-ended pipes. Outlets to dialysis machines should have a relatively short path with the least amount of fittings and the use of valves with minimal dead space. Voids, dead ends, and large surface areas serve as sites for microbial colonization. Also large diameter pipes decrease fluid velocity and increase the wetted surface area available for microbial colonization. In addition, long pipe runs also increase the available surface area for colonization. Gram-negative water bacteria in fluids remaining in pipes overnight can rapidly multiply and colonize wetted surfaces of the distribution system, producing microbial populations and endotoxin in quantities proportional to the total volume of the surface area. Such colonization results in the formation of protective biofilm, which is difficult to remove and protects the bacteria and other organisms from disinfection. Continuous circulation of water slows down this process.
Disinfection of the water or dialysate distribution system should be performed on a regular basis so that the microbial quality of the fluids is within the acceptable standards range. The frequency of disinfection should be validated by each facility and should be performed after any changes or modifications to the system. AAMI standards and recommended practices are community consensus standards, and do not specify a schedule for disinfection other than to suggest that routine disinfection be conducted. In many instances, microbiological monitoring can be used to determine the frequency of testing of disinfection of the distribution system. In some circumstances, repeat disinfection of the system cannot adequately control microbial growth because of established biofilm and replacement of the system is the only option.
To prevent disinfectant from draining from pipes by gravity before adequate contact time, distribution systems should be designed with all taps at equal elevation and at the highest point of the system. Furthermore, the system should be free of rough joints and dead-end pipes. Fluid trapped in such stagnant areas can serve as reservoirs for bacteria and fungi that later contaminate the rest of the distribution system.
Storage tanks greatly increase the volume of fluid and surface area of the distribution system. If used, these should be designed with a conical bottom so that water exits the storage tank at its lowest point (and allows the tank to be drained), be fitted with a tight-sealing lid, be equipped with a spray head, and possess an air vent containing a bacteriological filter. If used, the storage tanks should be routinely cleaned, disinfected, and drained. To remove biofilm, use of strong oxidizers may aid in stripping biofilm from surfaces; however, physical scrubbing of the inner surfaces of the tank may be necessary. When using a storage tank, an ultrafilter should be incorporated before water is pumped into the distribution system.
Hemodialysis Machines, Effluent, and Environmental Surfaces
In the 1970s, most dialysis machines were of the recirculating or recirculating single-pass type; their design contributed to relatively high levels of gram-negative bacterial contamination in dialysis fluid. Virtually all dialysis machines in the United States now are single-pass machines (i.e., the dialysate flows through the machine once). Single-pass machines tend to respond to adequate cleaning and disinfection procedures and, in general, have lower levels of bacterial contamination than do recirculating machines. Levels of contamination in single-pass machines depend primarily on the microbiological quality of the incoming water and the method of machine disinfection. Earlier dialysis machines had a port (waste-handling option) that allowed disposal of the extracorporeal circuit priming fluids. If one-way check valves in the waste-handling option are not maintained, checked for competency, or disinfected as recommended, it allows backflow from the effluent dialysate path into and contamination of the port and the attached bloodline. This led to outbreaks of infections among hemodialysis patients. The waste-handling option is much less commonly used now.
The external surfaces of dialysis machines and components are also likely sources for contamination. These include frequently touched surfaces (e.g., the control panel, dialysis chairs, keyboard, shared charting computers), attached priming buckets used during the priming of the dialyzers, blood tubing draped or clipped to waste containers, or other equipment brought into the station. For example, among nine outbreaks of bacteremia, fungemia, and pyrogenic reactions not related to dialyzer reuse investigated by the Centers for Disease Control and Prevention (CDC), inadequate disinfection of the water distribution system or dialysis machines was implicated in seven ( Table 25.3 ). Surface contamination has been described as a potential contributor to transmission of bloodborne pathogens in the context of other poor practices. A novel source of transmission has been identified: dialysis wall boxes, which contain several connections that allow the dialysis machine to hook up to the water supply and drain effluent. A large outbreak of bloodstream infections caused by Serratia marcescens, Pseudomonas aeruginosa, Enterobacter cloacae , and other gram-negative bacteria was identified and wall boxes were determined to be the source. More work is needed to understand the role of wall boxes and other surfaces and related infection control aspects in transmission of pathogens.
Description | Cause(s) of Outbreak | Corrective Measure(s) Recommended | Reference |
---|---|---|---|
Bacteremia, Fungemia, or Pyrogenic Reactions Not Related to Dialyzer Reuse | |||
Pyrogenic reactions in 49 patients | Untreated city water contained high levels of endotoxin | Install a reverse osmosis system | |
Pyrogenic reactions in 45 patients | Inadequate disinfection of the fluid distribution system | Increase disinfection frequency and contact time | |
Pyrogenic reactions in 14 patients; 2 cases of bacteremia; 1 death | Reverse osmosis water storage tank contaminated with bacteria | Remove or properly maintain and disinfect the storage tank | |
Pyrogenic reactions in 6 patients; 7 cases of bacteremia | Inadequate disinfection of water distribution system and dialysis machines; improper microbial assay procedure | Use correct microbial assay procedures; disinfect water treatment system and dialysis machines following manufacturer’s recommended procedures | |
Bacteremia in 35 patients with central venous catheters (CVCs) | CVCs used as facilities’ primary vascular access; median duration of infected catheters was 311 days; improper aseptic techniques | Uses CVCs when only absolutely necessary for vascular access; use appropriate aseptic technique when inserting and performing routine catheter care | |
Three pyrogenic reactions and 10 cases of bacteremia in patients treated on machines with a port for disposal of dialyzer priming fluid (waste handling option [WHO] port) | Incompetent check valves allowing backflow of fluid from the waste side of the machine into attached blood tubing; bacterial contamination of the WHO | Routine disinfection and maintenance of the dialysis machine including the WHO; check competency of WHO before patient treatment | |
Bacteremia in 10 patients treated on machines with WHO port | Incompetent backflow to allow backflow from dialysate effluent side of the machine in the WHO port and attached bloodlines | Routine maintenance, disinfection, and check for check valve competence of the WHO port | |
Outbreak of pyrogenic reactions and gram-negative bacteremia in 11 patients | Water distribution system and machines were not routinely disinfected according to manufacturer’s recommendations Water and dialysate samples were cultured using a calibrated loop and blood agar plates—results always indicated no growth | Disinfect machines according to manufacturer’s recommendations; include reverse osmosis water distribution system in the weekly disinfection schedule; microbiological assay should be performed via membrane filtration or spread plate using Trypticase soy agar | |
Phialemonium curvatum access infections in four dialysis patients; two of these patients died of systemic disease | Observations at the facility noted some irregularities in site preparation for needle insertion All affected patients had synthetic grafts One environmental sample was positive for P. curvatum (condensate pan of HVAC serving the unit) | Review infection control practices clean and disinfect HVAC system where water accumulated; perform surveillance on all patients | |
P. curvatum bloodstream infection (BSI) in two patients | Water system and dialysis machines with WHO ports not routinely maintained; water system contained dead legs and laboratory used wrong assays | Conduct routine maintenance and disinfection of machines and WHO ports; redesign water system to eliminate dead legs; have a routine schedule for disinfection of the water system | |
Outbreak of gram-negative BSI in 58 patients | Poor infection control practices, contamination from dialysis wall boxes | Improve infection control practices, cleaning and disinfection of wall boxes | |
Bacteremia/Pyrogenic Reactions Related to Dialyzer Reprocessing | |||
Mycobacterial infections in 27 patients | Inadequate concentration of dialyzer disinfectant | Increase formaldehyde concentration used to disinfect dialyzers to 4% | |
Mycobacterial infections in five high-flux dialysis patients; two deaths | Inadequate concentration of dialyzer disinfectant and inadequate disinfection of water treatment system | Use higher concentration of peracetic acid for reprocessing dialyzers and follow manufacturer’s labeled recommendations; increase frequency of disinfecting the water treatment system | |
Bacteremia in six patients | Inadequate concentration of dialyzer disinfectant; water used to reprocess dialyzers did not meet AAMI standards | Use AAMI-quality water; ensure proper germicide concentration in the dialyzer | CDC unpublished data |
Bacteremia and pyrogenic reactions in six patients | Dialyzer disinfectant diluted to improper concentration | Use disinfectant at the manufacturer’s recommended dilution and verify concentration | |
Bacteremia and pyrogenic reactions in six patients | Inadequate mixing of dialyzer disinfectant | Thoroughly mix disinfectant and verify proper concentration | |
Bacteremia in 33 patients at 2 dialysis centers | Dialyzer disinfectant created holes in the dialyzer membrane | Change disinfectant (product was withdrawn from the market by the manufacturer) | |
Bacteremia in six patients; all blood isolates had similar plasmid profiles | Dialyzers were contaminated during removal and cleaning of headers with gauze; staff not routinely changing gloves; dialyzers not reprocessed for several hours after disassembly and cleaning | Do not use gauze or similar material to remove clots from header; change gloves frequently; process dialyzers after rinsing and cleaning | |
Pyrogenic reactions in three high-flux dialysis patients | Dialyzer reprocessed with two disinfectants; water for reuse did not meet AAMI standards | Do not disinfect dialyzers with multiple germicides; more frequent disinfection of water treatment system and conduct routine environmental monitoring of water for reuse | |
Pyrogenic reactions in 14 high-flux dialysis patients; 1 death | Dialyzers rinsed with city (tap) water containing high levels of endotoxin; water used to reprocess dialyzers did not meet AAMI standards | Do not rinse or reprocess dialyzers with tap water; use AAMI-quality water for rinsing and preparing dialyzer disinfectant | |
Pyrogenic reactions in 18 patients | Dialyzers rinsed with city (tap) water containing high levels of endotoxin; water used to reprocess dialyzers did not meet AAMI standards | Do not rinse or reprocess dialyzers with tap water; use AAMI-quality water for rinsing and preparing dialyzer disinfectant | |
Pyrogenic reactions in 22 patients | Water for reuse did not meet AAMI standards; improper microbiological technique was used on samples collected for monthly monitoring | Use the recommended assay procedure for water analysis of water and dialysate; disinfect water distribution system | |
Bacteremia and candidemia among patients in seven dialysis units (in Minnesota and California) | Dialyzers were not reprocessed in a timely manner; some dialyzer refrigerated for extended periods before reprocessing; company made changes to header cleaning protocol | Reprocess dialyzers as soon as possible; follow joint CDC and dialyzer reprocessing equipment and disinfectant manufacturer guidance for cleaning and disinfecting headers of dialyzer | CDC unpublished Data |
Outbreak of gram-negative BSI, including Burkholderia cepacia and Stenotrophomonas maltophilia in 17 patients | B. cepacia was isolated from header cleaning machine matched patient isolates Contamination likely was due to incomplete disinfection during reprocessing | Reuse was stopped | |
Transmission of Viral Agents | |||
26 patients seroconverted to HBsAg+ during a 10-month period | Leakage of coil dialyzer membranes and use of recirculating bath dialysis machines | Separation of HBsAg+ patients and equipment from all other patients | |
19 patients and 1 staff member seroconverted to HBsAg+ during a 14- month period | No specific cause determined; false-positive HBsAg results caused some susceptible patients to be dialyzed with infected patients | Laboratory confirmation of HBsAg+ results; strict adherence to glove use and use of separate equipment for HBsAg+ patients | |
24 patients and 6 staff seroconverted to HBsAg+ during a 10-month period | Staff not wearing gloves; surfaces not properly disinfected; improper handling of needles/sharps resulting in many staff needlestick injuries | Separation of HBsAg+ patients and equipment from susceptible patients; proper precautions by staff (e.g., gloves; handling of needles and sharps) | |
13 patients and 1 staff member seroconverted to HBsAg+ during a 1-month period | Extrinsic contamination of intravenous medication being prepared adjacent to an area where blood samples were handled | Separate medication preparation area from area where blood processing for diagnostic tests is performed | |
Eight patients seroconverted to HBsAg+ during a 5-month period | Extrinsic contamination of multidose medication vial shared by HBsAg+ and HBsAg-susceptible patients | No sharing of supplies, equipment, and medications between patients | (CDC, unpublished data) |
Seven patients seroconverted to HBsAg+ during a 3-month period | Same staff caring for HBsAg+ and HBsAg-susceptible patients | Separation of HBsAg+ patients from other patients; same staff should not care for HBsAg+ and HBsAg– patients | |
Eight patients seroconverted to HBsAg+ during a 1-month period | Not consistently using external pressure transducer protectors; same staff members cared for both HBsAg+ patients and susceptible patients | Use external pressure transducer protectors and replace after each use; same staff members should not care for HBV-infected and -susceptible patients on the same shift | |
14 patients seroconverted to HBsAg+ during a 6-week period | Failure to review results of admission and monthly HBsAg testing; inconsistent handwashing and use of gloves; adjacent clean and contaminated areas; <20% of patients vaccinated | Proper infection control precautions for dialysis facilities; routine review of serological testing; hepatitis B vaccination of all patients | |
Seven patients on the same shift seroconverted to HBsAg+ during a 2-month period | Same staff members cared for HBsAg+ and HBsAg– patients on the same shift; common medication and supply carts were moved between stations, and multidose vials were shared | Dedicated staff for HBsAg+ patients; no sharing of equipment or supplies between any patients; centralized medication and supply areas; hepatitis B vaccination of all patients | |
Four patients seroconverted HBsAg+ during a 3-month period | Transmission appeared to occur during hospitalization at an acute care facility | Hepatitis B vaccination of all patients | |
11 patients seroconverted to HBsAg+ during a 3-month period | Staff, equipment, and supplies were shared between HBsAg+ and HBs– patients; no patients were vaccinated | Dedicated staff for HBsAg+; no sharing of medication or supplies between any patients; hepatitis B vaccination of all patients | |
Two patients converted to HBsAg+ during a 4-month period | Transmission appeared to occur during hospitalization at an acute care facility; Same staff cared for HBsAg+ and HBs– patients; no patients vaccinated | Hepatitis B vaccination of all patients; dedicate staff for the care of HBsAg+ patients; no sharing of supplies or medication between patients | |
One patient converted to HBsAg+ | Transmission from a patient with history of resolved HBV infection, but the infection reactivated as a result of immunosuppression; multiple infection control breaches observed | Awareness of the reactivation/reserve seroconversion situation | |
36 patients with liver enzyme elevations consistent with non-A, non-B hepatitis | Environmental contamination with blood | Use proper precautions (e.g., gloving of staff; environmental cleaning); monthly liver function tests (e.g., ALT) | |
35 patients developed elevated liver enzymes consistent with non-A, non-B hepatitis during a 22-month period; 82% of probable cases were anti-HCV+ | Inconsistent use of infection control precautions, especially hand washing | Strict compliance to aseptic technique and dialysis center precautions | |
HCV infection developed in 7 out of 40 (17.5%) HCV-susceptible patients; shift specific attack rates of 29%–36% | Multidose vials left on top of machine and used by multiple patients; routine cleaning and disinfection of surfaces and equipment between patients not routinely done; arterial line for draining prime draped into a bucket that was not routinely cleaned or disinfected between patients | Strict compliance with infection control precautions for all dialysis patients; routine HCV testing | |
HCV infection developed in 5 out of 61 (8%) HCV-susceptible patients | Sharing of equipment and supplies between chronically infected and susceptible patients; gloves not routinely used; clean and contaminated areas not separated | Strict compliance with infection control precautions for all dialysis patients; CDC does not recommend separation of equipment/supplies between HCV-infected and -susceptible patients | |
HCV infection developed in 3 out of 23 (13%) HCV- susceptible patients | Supply carts moved between stations and contained both clean and blood-contaminated items; medications prepared in the same area used for disposal of used injection equipment | Strict compliance with infection control precautions for all dialysis patients | |
HCV infection developed in 7 out of 52 (13%) HCV-susceptible patients; shift-specific attack rates 4%–21% | Medication cart moved between stations and contained both clean and blood-contaminated items; single-dose medication vials used for multiple patients; cleaning and disinfection of surfaces and equipment between patients not routinely done | Strict compliance with infection control precautions for all dialysis patients | |
HCV infection developed in 9 out of 119 (7.6%) patients; attack rate 10% | Cleaning and disinfection of surfaces and equipment between patients not routinely done; gloves not routinely used; medications not stored in separate clean area | Strict compliance with infection control precautions for all dialysis patients; perform routine HCV testing | |
HCV infections developed in 6 out of 66 (9%) patients | Clean and contaminated areas not well delineated; clean supplies accessed with contaminated gloves; medication preparation in proximity to blood specimen processing; reuse of single-dose vial | Strict compliance with infection control precautions for all dialysis patients | CDC unpublished data |
HCV infections developed in 8 out of 149 (5.4%) patients; attack rate 8.6% | Multidose heparin vials taken to individual dialysis stations; poor hand hygiene and glove use; poor cleaning and disinfection practices | Strict compliance with infection control precautions for all dialysis patients | |
HCV infections developed in 18 patients; attack rate 16.7% | Poor hand hygiene and glove use; poor cleaning and disinfection practices; blood stains found on machine surfaces after cleaning | Strict compliance with infection control precautions for all dialysis patients | |
HCV infections developed in 16 patients at 9 facilities between 2013 and 2015 | Multiple infection control breaches identified | Strict compliance with infection control precautions for all dialysis patients |
Hemodialyzer Reuse
Reuse of disposable hollow-fiber dialyzers in the United States increased between 1976 and 1982, from 18% to 43% of facilities reporting reuse; the highest percentage was 82% in 1997. By 2002 the percentage of facilities reporting reusing dialyzers had declined to 63%. Recent data from the CDC’s National Healthcare Safety Network indicated that only 1.8% of facilities reported reuse in 2017 (CDC, unpublished data). This decline coincides with decisions made by several large dialysis organizations to discontinue the practice of reuse and to only use single-use dialyzers. Although dialyzer reuse is still common in developing countries, it has become less popular in developed countries and some have plans to phase out this practice. After a series of outbreaks of bacterial infections associated with reuse and reprocessing of dialyzers, CDC recommended that single use of dialyzers be the preferred practice and stated that it should be used whenever possible.
In 1986, AAMI standards for reprocessing hemodialyzers were adopted by the United States Public Health Service and was incorporated into regulation by CMS. In the United States, dialyzer reuse has not been associated with the transmission of bloodborne pathogens such as hepatitis B (HBV), hepatitis C (HCV), or human immunodeficiency virus (HIV). However, the reprocessing of dialyzers has been associated with pyrogenic reactions and bacterial infections. These adverse events may be the result of the use of incorrect concentrations of chemical germicides, the failure to maintain appropriate water quality, breaks in reprocessing procedures, or practical challenges to achieving complete disinfection of reused dialyzers. Manual reprocessing of dialyzers, which is allowed in the United States, does not include testing for membrane integrity, such as a pressure-leak test, may fail to detect membrane defects, and relies on disinfection processes that are particularly difficult to standardize.
Dialyzer reprocessing can be performed in myriad ways with few quality control checks. Procedures used to reprocess hemodialyzers generally constitute high-level disinfection rather than sterilization. Several liquid chemical germicides have been used for high-level disinfection of dialyzers. There are commercially available chemical germicides specifically formulated for this purpose (e.g., peroxyacetic acid, chlorine-based, and glutaraldehyde-based products that are approved by the US Food and Drug Administration [FDA] as sterilants or high-level disinfectants for reprocessing hemodialyzers). During the period between 1983 and 2002, the percentage of centers using formaldehyde for reprocessing dialyzers decreased from 94% to 20%, whereas the percentage using peroxyacetic acid increased from 5% to 72%. Only a minority of facilities (4%) reported used either glutaraldehyde or heat disinfection.
Using a suboptimal disinfectant may lead to outbreaks of infection, such as nontuberculous mycobacteria. An outbreak of systemic mycobacterial infections in five hemodialysis patients, resulting in two deaths, occurred when high-flux dialyzers were contaminated with Mycobacterium abscessus during manual reprocessing and disinfected with a commercial disinfectant prepared at a concentration that did not ensure complete inactivation of mycobacteria. These and other outbreaks of infections in dialysis patients emphasize the need to reconsider the safety and necessity of dialyzer reuse.
Outbreaks of pyrogenic reactions (defined as fever or chills in a patient who was afebrile and had no signs or symptoms of an infection before the start of the dialysis treatment session) have often resulted from reprocessing hemodialyzers with water that did not meet AAMI standards (see Table 25.3 ). In most instances the water used to rinse dialyzers or to prepare the dialyzer disinfectants exceeded the allowable AAMI microbial or endotoxin standards, because the water distribution system was not disinfected frequently, the disinfectant was improperly prepared, or routine microbial assays were improperly performed. Several outbreaks associated with dialyzer reuse have been reported. Breaches in disinfection of dialyzer components (such as an O-ring) and contamination caused by poor infection control practices during reprocessing steps have been identified as major contributors to those outbreaks. In at least one outbreak, no major breaches in reprocessing were identified. Rather, it was determined that dialyzers are difficult to reprocess safely and completely under typical conditions. This is due to poorly trained staff (often in low-paying jobs), variability in procedures, and few quality control standards.
As described in the most recent investigation of a reuse associated outbreak that resulted in 17 cases, “In practice, reuse and reprocessing of dialyzers poses an increased risk for infection to patients.” In this investigation, each additional use of a dialyzer was associated with higher odds of bloodstream infection. In the era of affordable single-use dialyzers, dialysis providers have discontinued reuse in the interest of patient safety. For facilities or regions where reuse and reprocessing continues to be performed, improved standardization of processes and rigorous quality assurance programs are needed.
High-Flux Dialysis and Bicarbonate Dialysate
High-flux dialysis uses dialyzer membranes and hydraulic permeability that are 5 to 10 times greater than conventional dialyzer membranes. There has been concern that bacteria or more likely endotoxin in the dialysate may penetrate these highly permeable membranes.
High-flux membranes require the use of bicarbonate rather than acetate dialysate. Bicarbonate dialysate must be prepared from two concentrates, an acid concentrate (acetic acid or citric acid) with a pH of 2.8 that is not conducive to microbial growth and a bicarbonate concentrate with a relatively neutral pH and a salt molarity of 1.2 M. Because the bicarbonate concentrate will support rapid growth, its use can increase microbial and endotoxin concentrations in the dialysate and theoretically may contribute to an increase in pyrogenic reactions, especially when used during high-flux dialysis.
Some of the concern appeared justified by results of surveillance data during the 1990s showing a significant association between use of high-flux dialysis and reporting of pyrogenic reactions among patients during dialysis. However, a prospective study of pyrogenic reactions in patients receiving more than 27,000 conventional, high-efficiency, or high-flux dialysis with bicarbonate dialysate containing high concentrations of bacteria and endotoxin found no association between pyrogenic reactions and the type of dialysis treatment. Although there seems to be conflicting data on the relationship between high-flux dialysis and pyrogenic reactions, centers providing high-flux dialysis should ensure that dialysate meets AAMI microbial standards (see Table 25.2 ).
Disinfection of Hemodialysis Systems
Routine disinfection of isolated components of the dialysis system often produces inadequate results. Consequently, the total dialysis system (water treatment system, distribution system, and dialysis machine) should be included in the disinfection procedure.
Disinfection of dialysis systems usually employs sodium hypochlorite solutions, hydrogen peroxide solutions, commercially available peracetic acid disinfectants, ozone, and, in some systems, hot water pasteurization. Sodium hypochlorite solutions are convenient and effective in most parts of the dialysis system when used at the manufacturer’s recommended concentrations. Also, the test for residual available chlorine to confirm adequate rinsing is simple and sensitive. However, because chlorine is corrosive, it is usually rinsed from the system after a relatively short dwell time of 20 to 30 minutes. The rinse water invariably contains organisms that can multiply to significant levels if the system is permitted to stand overnight. Therefore disinfection with chlorine-based disinfectants are best used before the start of the first patient treatment session rather than at the end of the day. However, for models of machines that most dialysis facilities are using, options for disinfection include heat at the end of the day and use of other disinfectants with longer contact time that also require overnight dwell. There is no need to disinfect the fluid pathway between patients.
Aqueous formaldehyde, peroxyacetic acid, hydrogen peroxide, or glutaraldehyde solutions can produce good disinfection results. These products are not as corrosive as hypochlorite solutions and can be allowed to dwell in the system for long periods of time when the system is not in operation. However, formaldehyde, which has good penetrating power, is considered an environmental hazard and potential carcinogen and has irritating qualities that may be objectionable to staff. The US Environmental Protection Agency has also limited the amount of formaldehyde that can be discharged into the wastewater stream, which has drastically reduced the use of this chemical in the dialysis community as a disinfectant. Peroxyacetic acid and glutaraldehyde are commercially available and are designed for use with dialysis machines when used according to the manufacturers labeled instructions. Glutaraldehyde use is also limited because it is considered a sensitizer and may pose a risk to healthcare workers.
Some dialysis systems (both water treatment and distribution systems, some hemodialysis machines) use hot-water disinfection (pasteurization) for control of microbial contamination. In this type of system water heated to >80°C (176°F) is passed through the water distribution system and hemodialysis machine or just the hemodialysis machine at the end of the day. These systems are excellent for controlling microbial contamination. However, it should be noted that heat disinfection of the hemodialysis machine would not control microbial contamination of the waste lines and effluent drains. Additional processes may be needed to disinfect waste lines, drains, and wall boxes.
Monitoring of Water and Dialysis Fluid
Microbiological and endotoxin standards for water and dialysis fluids (see Table 25.2 ) were originally based on the results of culture assays performed during outbreak investigations. There is increasing evidence that the microbial quality of hemodialysis fluids plays a role in the chronic inflammatory response syndrome, affects anemia management, accelerates loss of residual renal function, and affects serum albumin levels in dialysis patients. Increasing data suggest that use of ultrapure water and dialysate would benefit maintenance hemodialysis patients and potentially save costs. A large cohort study from Japan found a lower all-cause mortality in facilities using ultrapure water. However, there have been no randomized controlled studies to evaluate and confirm these studies, so regulatory agencies have not yet mandated these higher water standards.
Water samples for routine testing should be collected from a source as close as possible to where water enters the dialysate proportioning unit. In most cases this is the tap (not from that hose connecting the tap to the dialysis machine) at the dialysis station ( Fig. 25.1 ). Water samples should be collected at least monthly from several locations within the dialysis unit, including samples at different dialysis stations. Samples should also be collected using a similar approach after any modifications or maintenance have been made to the water treatment system water distribution system. Dialysate samples should be collected during or at the end of the dialysis treatment from a source close to where the dialysis fluid either enters or leaves the dialyzer ( Fig. 25.2 ). Dialysate samples should be collected at least monthly from a representative number of dialysis machines. Samples of water and dialysate should also be collected when a pyrogenic reaction is suspected. If centers reprocess hemodialyzers for reuse, water used to prepare disinfectant and rinse dialyzers should also be assayed monthly. The maximum contaminant levels for water are 100 CFU/mL and 0.25 EU/mL (see Table 25.2 ). Methods for microbiological and endotoxin testing are available elsewhere.


In an outbreak investigation, the assay methods may need to be both qualitative and quantitative; also detection of nontuberculous mycobacteria and in some cases fungi in water or dialysate may be desirable. In such instances, plates should be incubated for 5 to 14 days at both 36°C and 28° to 30°C. Laboratories should be notified of special testing requests outside of routine water testing, such as if the facilities would like to look for specific pathogens.
Dialysis-Associated Pyrogenic Reactions
Gram-negative bacterial contamination of dialysis water or components of the dialysis system (water, dialysate, water used for reprocessing) can cause pyrogenic reactions. A pyrogenic reaction is defined as objective chills (visible rigors) or fever (oral temperature ≥ 37.8°C [100°F]) or both in a patient who was afebrile (oral temperature up to 37°C [98.6°F]) and had no signs or symptoms of an infection before the start of the dialysis treatment session. Depending on the type of dialysis system and the level of contamination, fever and chills may start 1 to 5 hours after dialysis has been initiated. Other symptoms may include hypotension, headache, myalgia, nausea, and vomiting. Pyrogenic reactions can occur without bacteria; because presenting signs and symptoms cannot differentiate bacteremia from pyrogenic reactions, blood cultures are necessary.
During 1990–2002 an annual average of 20% to 24% of the hemodialysis centers in the United States reported at least one pyrogenic reaction in the absence of septicemia in their patients undergoing maintenance hemodialysis. Pyrogenic reactions can result from passage of bacterial endotoxin (lipopolysaccharide) or other substances in the dialysate across the dialyzer membrane or from the transmembrane stimulation of cytokine production in the patient’s blood by endotoxin in the dialysate. In other instances, endotoxin can enter directly into the bloodstream with fluids that are contaminated with gram-negative bacteria. The signs and symptoms of pyrogenic reactions without bacteremia generally abate within a few hours after the dialysis has been stopped. If gram-negative sepsis is associated, fever and chills may persist and hypotension is more refractory to therapy.
When a patient develops a pyrogenic reaction (i.e., onset of fever or chills) while being dialyzed, the following steps are recommended: (1) careful physical examination of the patient to identify signs and symptoms and evaluate other possible causes of chills and fever (e.g., pneumonia, vascular access site infection); (2) blood cultures, other diagnostic tests (e.g., chest radiograph), and other cultures as clinically indicated; (3) collection of dialysate from the dialyzer (i.e., postdialyzer effluent sample) for quantitative and qualitative microbiological culture; and (4) recording of the incident in a log or other permanent record. In addition, empiric antibiotic treatment should be administered to the patient. Determining the cause of such episodes is important because they may be the first indication of a remediable problem that can affect a potentially large number of patients.
The higher the level of bacteria and endotoxin in dialysis fluid, the higher the probability that the bacteria or their products will pass through the dialyzer membrane to produce bacteremia or stimulate cytokine production. In an outbreak of febrile reactions among patients undergoing hemodialysis, the attack rates were directly proportional to the level of microbial contamination in the dialysis fluid. Prospective studies also reported a lower pyrogenic reaction rate among patients when they underwent dialysis with dialysis fluid from which most bacteria had been removed by filtration, compared with patients who underwent dialysis fluid that was highly contaminated (mean 19,000 CFU/mL).
Disinfection, Sterilization, and Environmental Cleaning in Dialysis Facilities
Good cleaning, disinfection, and sterilization procedures are important components of the infection control program in the hemodialysis center. The procedures do not differ from those recommended for other healthcare settings, but the high potential for blood contamination makes the hemodialysis setting unique. In addition, the need for routine aseptic access of the patient’s vascular system makes the hemodialysis unit more akin to a surgical suite than to a standard hospital room. Medical items are categorized as critical (e.g., needles and catheters), which are introduced directly into the bloodstream or normally sterile areas of the body; semicritical (e.g., fiberoptic endoscopes), which come in contact with intact mucous membranes; and noncritical (e.g., blood pressure cuffs), which touch only intact skin.
Cleaning and housekeeping in the dialysis center have two goals: to remove soil and waste on a regular basis, thereby preventing the accumulation of potentially infectious material, and to maintain an environment that is conducive to good patient care. Crowding of patients and patient stations, as well as overtaxing of staff members, may increase the likelihood of microbial transmission. Adequate cleaning may be difficult if there are multiple wires, tubes, and hoses in a small area. There should be enough space to move completely around each patient’s dialysis station without interfering with the neighboring stations. According to the Facility Guidelines Institute, each dialysis station should be at least 80 square feet and allow at least 4 feet distance between stations to avoid contamination. However, most of dialysis facilities do not have space to meet that guideline. To avoid contamination, cleaning should only start when patients have left the station and staff should not allow new patients into chairs until cleaning and disinfection is complete. Creating unit-wide patient-free intervals between treatment shifts is likely to improve the adequacy of station cleaning and disinfection between patients.
Where space is limited, elimination of unneeded items, orderly arrangement of required items and removal of excess lengths of tubes, hoses, and wires from the floor can improve accessibility for cleaning. Because of the special requirements for cleaning in the dialysis center, staff should be specially trained in this task.
After each patient treatment, frequently touched environmental surfaces, including external surfaces of the dialysis machine, should be properly disinfected; some surfaces may also require precleaning (with a detergent) before disinfection. A study in the Netherlands and an investigation of a large HCV outbreak in the United States where the investigators used chemiluminescent agents to detect nonvisible blood contamination have demonstrated the importance of environmental cleaning. Antiseptics, such as formulations with povidone-iodine, hexachlorophene, or chlorhexidine, should not be used for surface disinfection because these are formulated for use on skin and are not designed for use on hard surfaces. Given the role of environmental surfaces of components adjacent to the machine (e.g., wall boxes) in transmission of pathogens, as illustrated in recent outbreak, attention should be paid to cleaning and disinfection of those surfaces as well.
Bloodstream Infections and Other Infections
The annual adjusted mortality rate among hemodialysis patients is 169 per thousand patient-years at risk. Infection is the second leading cause of mortality in this patient population, accounting for 8% of all deaths. In a number of published studies that have evaluated bacterial infections in outpatient hemodialysis, bacteremia occurred in 0.6% to 1.7% of patients per month and vascular access infections (VAIs; with or without bacteremia) in 1.3% to 7.2% of patients per month. A review of four studies published during 2002 estimated that 1.8% of hemodialysis patients have vascular access associated bacteremia each month, amounting to 50,000 episodes nationally per year. In a study of 27 French hemodialysis centers, 28% of 230 infections in hemodialysis patients involved the vascular access, whereas 25% involved the lung, 23% the urinary tract, 9% the skin and soft tissues, and 15% other or unknown sites.
Because of the importance of bacterial infections in hemodialysis patients, the CDC initiated a voluntary ongoing surveillance system in the United States called the Dialysis Surveillance Network (DSN) in 1999. At the time, only bacterial infections associated with hospital admission or intravenous antimicrobial receipt were counted; as a result, this system likely only detected more severe infections. During 1999–2001, 109 dialysis centers reported data. Rates per 100 patient-months were 3.2 for all VAIs (including access infections both with and without bacteremia), 1.8 for vascular access associated bacteremia, 1.3 for wound infection not related to the vascular access, 0.8 for pneumonia, and 0.3 for urinary tract infection. Among patients with fistulas or grafts, wounds were the most common site for infection. Among patients with hemodialysis catheters, infections of the vascular access site were the most common site for infection. The surveillance project expanded and evolved into National Healthcare Safety Network (NHSN), of which Dialysis Event Surveillance is a component ( https://www.cdc.gov/nhsn/dialysis/index.html ).The NHSN is an Internet-based surveillance system that enables facilities to report healthcare-associated infection data to the CDC. VAIs in dialysis patients and related events are reported to NHSN’s Dialysis Event Surveillance. Outpatient hemodialysis facilities in the United States eligible to participate in the surveillance are instructed to follow a standard protocol, by which all outpatients who receive hemodialysis at the facility are monitored for three NHSN-defined dialysis events. The three types of dialysis events (positive blood culture; intravenous antimicrobial start; and pus, redness, or increased swelling at the vascular access site) are reported using a standard data collection form. During 2007–2011, 193 facilities reported to NHSN; the rate of bloodstream infection (BSI) and access-related BSI was 1.27 and 0.88 per 100 patient-months, respectively. Data reported to NHSN have been used by CMS as part of the ESRD Quality Incentive Program since 2012; as a result, almost all outpatient hemodialysis facilities now report to NHSN. In 2014 more than 6000 facilities now reported 160,971 events, including 29,516 BSIs and 22,576 access-related BSIs; the rate of BSI was 0.64 per 100 patient-months. The rate of BSI was much higher among patients with a central venous catheter (2.16 per 100 patient-months) compared with other vascular access types.
Vascular Access Infections
Access site infections are particularly important because they can cause disseminated bacteremia or loss of the vascular access. Local signs of VAI include erythema, warmth, induration, swelling, tenderness, breakdown of skin, loculated fluid, or purulent exudates. Based on data from DSN collected during 1995–2005, the overall VAI rate was 3.1 per 100 patient-months and varied from 0.6 for fistulas to 10.1 for temporary catheters. In the 2014 NHSN surveillance data report, the VAI rate was 1.21 per 100 patient-months. The access-related BSI rate was 0.49 per 100 patient-months, which varied by access type: 0.16 for fistulas, 0.27 for grafts, and 1.83 for central venous catheters (tunneled and nontunneled).
VAIs are caused (in descending order of frequency) by Staphylococcus aureus (32% to 53% of cases), coagulase negative staphylococci (20% to 32% of cases), gram-negative bacilli (10% to 18%), other gram-positive cocci (including enterococci; 10% to 12%), and fungi (<1%). Among BSIs, S. aureus remained the most commonly reported pathogen in 2014 NHSN data (31% of BSI and 32% of access-related BSI), and 40% of cases of S. aureus were resistant to methicillin.
The primary risk factor for vascular access–related infection is access type, with catheters having highest risk for infection; grafts intermediate; and native arteriovenous (AV) fistulas the lowest. Other potential risk factors for VAI include (1) location of the access in the lower extremity; (2) recent vascular access surgery; (3) trauma, hematoma, dermatitis, or scratching over the access site; (4) poor patient hygiene; (5) poor needle insertion technique; (6) older age; (7) diabetes; (8) immunosuppression; (9) iron overload; (10) intravenous drug use; and (11) chronic inflammatory state.
Based on the relative risk for both infectious and noninfectious complications, native AV fistulas are considered the preferred vascular access type; a goal of no more than 10% of patients maintained with permanent catheter–based hemodialysis treatment is recommended. To minimize infectious complications, patients should be referred early for creation of an arteriovenous access, thereby decreasing the time dialyzed through a temporary catheter. During the period between 1995 and 2002, the percentage of patients dialyzed through fistulas increased from 22% to 33%, with most of the increase occurring after 1999. Data from Dialysis Outcomes and Practice Patterns Study indicated that from August 2010 to August 2013, AV fistula use increased from 63% to 68%, whereas catheter use declined from 19% to 15%. However, the majority of incident patients still initiated dialysis with a catheter. The US Renal Data System (USRDS) annual data report for 2016 indicated that whereas 18.8% of prevalent hemodialysis patients used a catheter, 80.3% of incident patients started dialysis with a catheter.
Etiology and Prevention of Bloodstream Infection
Bacterial pathogens causing infection can either be exogenous (i.e., acquired from contaminated dialysis fluids or equipment) or endogenous (i.e., caused by invasion of bacteria present in or on the patient). Catheter-related infections are most often caused by bacteria from the patient’s skin colonizing the outside of the catheter or from direct contact (e.g., touch contamination by healthcare personnel) with the catheter hub, leading to contamination of the inner surface of the catheter. Surveillance data indicate that S. aureus and other coagulase-negative staphylococci were the most common pathogens for BSI and access-related BSI. Endogenous sources may also be more likely causes of VAI among fistula and graft patients. Contaminated infusates and hematogenous spread are thought to be less common causes of BSI in this patient population, regardless of vascular access type.
Exogenous pathogens have caused numerous outbreaks, most of which resulted from inadequate dialyzer reprocessing procedures (e.g., contaminated water or inadequate disinfectant concentration) or inadequate disinfection and maintenance of the water treatment and distribution system. During 1995–2006, five outbreaks were traced to contamination of the waste handling option on one type of dialysis machine. Recommendations to prevent such outbreaks have been published elsewhere.
Contaminated medication vials are also a source of bacterial infection for patients. In 1999, an outbreak of Serratia liquefaciens bloodstream infections and pyrogenic reactions among hemodialysis patients was traced to contamination of vials of erythropoietin. These vials, which were intended for single use, were contaminated by repeated puncture to obtain additional doses and by pooling of residual medication into a common vial.
Recommendations for preventing VAIs have been developed by the CDC and the Healthcare Infection Control Practices Advisory Committee and the National Kidney Foundation. The CDC has developed a recommended “Approach to BSI Prevention in Dialysis Facilities” that includes core interventions to prevent BSI among hemodialysis patients ( Table 25.4 ). Facilities that implemented this set of interventions were able to reduce their access-related BSI rates and sustained these lowered rates for at least 4 years. The core interventions include (1) BSI surveillance using NHSN and feedback to clinical staff; (2) hand hygiene observations with feedback to staff; (3) catheter/vascular access care observations to ensure clinical staff adherence to aseptic technique and good infection control practices (with staff feedback); (4) development of staff infection prevention skills, demonstrated through competency assessments; (5) patient education and engagement in infection control processes; (6) decrease catheter prevalence; (7) catheter hub disinfection; and (8) bacitracin zinc/polymyxin B sulfate (Polysporin) triple ointment or povidone-iodine ointment applied to catheter exit sites. The CDC has also developed tools, protocol, and guidance to assist in the implementation of the interventions ( https://www.cdc.gov/dialysis/prevention-tools/index.html ).
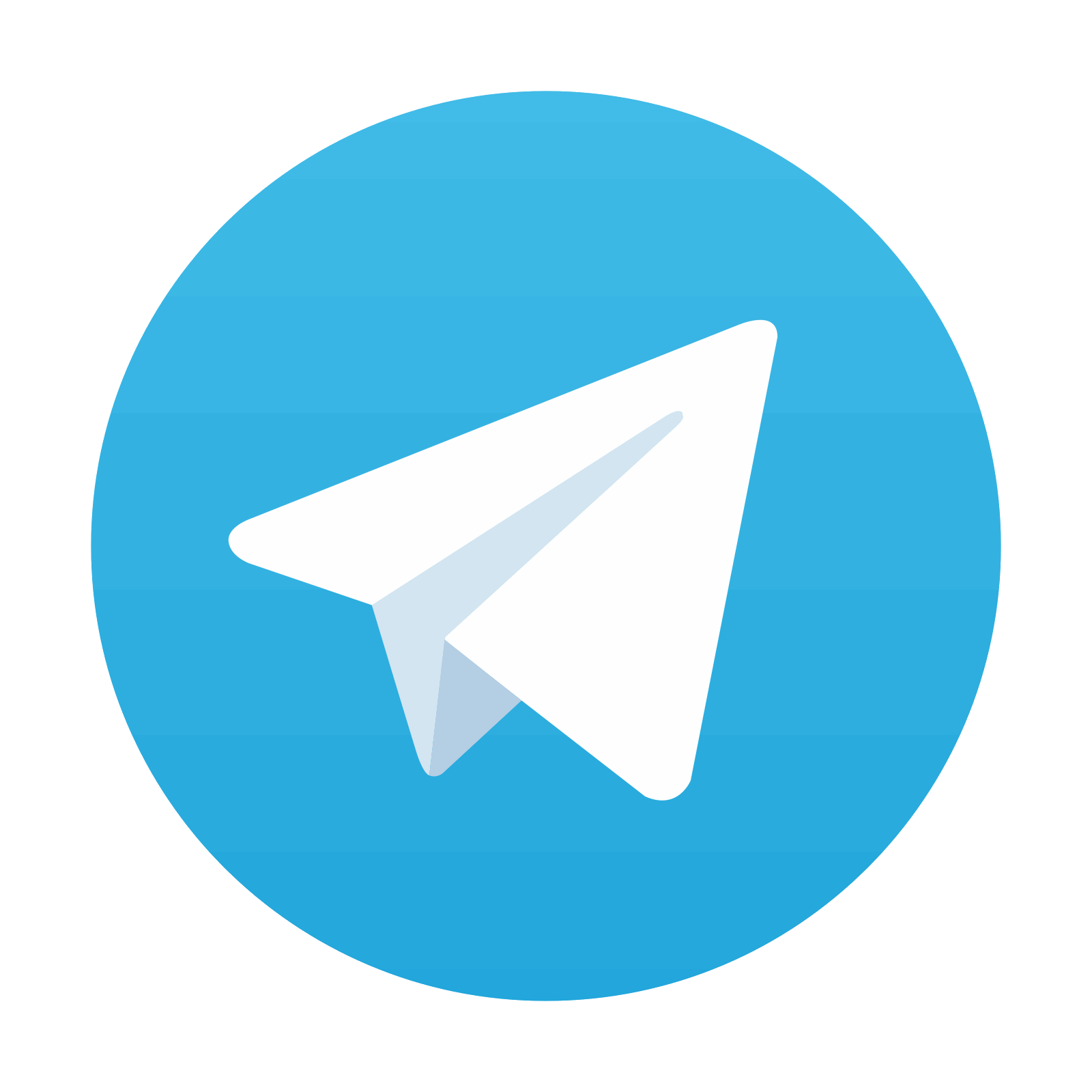
Stay updated, free articles. Join our Telegram channel

Full access? Get Clinical Tree
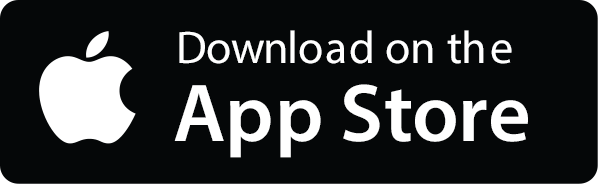
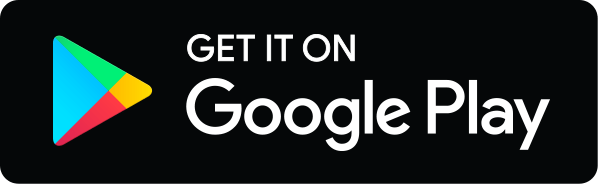
