Pathogenesis
FHF is not a disease but a syndrome, with a severity proportional to the degree of hepatic necrosis and the degree of the systemic response to this injury. FHF causes profound physiological derangement, characterized by encephalopathy, vasoparesis [9], and coagulopathy. As the syndrome progresses, cerebral edema [10] and renal failure are prominent and there is impaired immunity, with increased susceptibility to infection and subsequent multiple organ failure [11].
The rate of progression of FHF can be unpredictable in the hyperacute group. The syndrome typically evolves over several days, but deep coma can occur within hours. The mainstay of treatment in FHF is supportive while the decision to proceed to hepatic transplantation is being considered.
The cause of death in FHF can be split into two main groups; those with cerebral edema who die of brain ischemia or brain stem herniation, and those who succumb to sepsis and multiple organ failure. In a recent study of FHF patients listed for transplantation the major cause of death was multiple organ failure, with only 5% of patients succumbing to death directly related to intracranial hypertension [12]. This is at variance with previous surveys of cause of death in ALF, which showed a much higher incidence of cerebral deaths [13]. The reasons for this change are not clear, but better general ICU care and specifically the use of osmotherapy are likely to have been responsible for this change.
Intensive care management versus ward management
There have been no controlled clinical trials comparing intensive care with ward management, but considering the almost 100% mortality before the adoption of modern intensive care units, it seems likely that intensive care management reduces mortality [14]. Patients with grade III and IV encephalopathy should be intubated, ventilated and managed within an intensive care unit. The use of high-dependency areas for patients with liver failure and lower levels of coma is to be encouraged [15].
Management in a liver unit
Survival rates for FHF have increased markedly since the introduction of liver transplantation as a therapeutic modality for patients predicted to have a low rate of survival with medical management alone. Survival rates for FHF with medical therapy alone in cases that progress to grade III or IV encephalopathy are poor, varying between 10 and 40% depending on etiology. With the introduction of orthotopic liver transplantation (OLT) as a therapeutic option for patients with FHF, survival rates have increased to 80% at one year [16], and although not subjected to a randomized controlled trial it stands to reason, therefore, that the optimal management of patients with FHF should occur in liver units with ready access to a liver transplant program (see Figure 41.2).
FHF is a rare syndrome which can evolve rapidly; it is therefore crucial that patients predicted to have a severe course are identified early to allow safe and timely transfer to a liver unit. Criteria have therefore been developed to help advise peripheral hospitals when patients with FHF should be transferred to a liver unit (see Box 41.1) [17]. These criteria are based on clinical judgment and have not been subjected to a controlled clinical trial.
BOX 41.1 Transfer criteria
INR > 3.0
Prothrombin time in seconds greater than the time in hours
since the overdose (for paracetamol poisoning)
Any evidence of encephalopathy
Hypotension following fluid resuscitation
Evidence of a metabolic acidosis
Hyperlactemia
Bilirubin > 300μmol/L
Renal failure
General supportive management
Fluid resuscitation and circulatory management
Patients with FHF develop marked hemodynamic changes. Vasodilatation can be profound and is invariably accompanied by a compensatory increase in cardiac output [18]. This distributive shock, with relative hypovolemia, causes hypotension despite the increased cardiac output. Prognostic criteria such as acidosis, serum lactate and renal function should therefore only be assessed following adequate resuscitation, as there can be marked improvement in these following fluids.
The choice of resuscitation fluid in FHF is not clear. As yet there have been no randomized studies performed to specifically address this question. Colloid solutions provide greater volume expansion for a given volume transfused compared to crystalloids and were traditionally favored for resuscitation in critically ill patients until the publication of a meta-analysis of 30 randomized controlled trials in 1998, which suggested that albumin may increase mortality [19]. This publication led to a change in practice in many centers, although the methodology of the review was heavily criticized. A large randomized controlled clinical trial has since been performed in 7000 patients, comparing 4% human albumin solution and normal saline for the resuscitation of critically ill patients. This study found no difference in the primary endpoint of mortality between albumin and saline, but in subgroup analysis there was an increase in mortality in brain-injured patients receiving albumin, which needs to be subjected to further study [20]. A1a Despite the apparent safety of albumin, it is clear that its use has declined as a resuscitation fluid, with only 7% of units in a large European survey using albumin for resuscitation [21]. In practice, most patients will receive a mixture of colloid and crystalloid for resuscitation and no clear evidence-based recommendation can be given as to which crystalloid or colloid is appropriate in FHF patients. Balanced salt solutions are associated with less risk of hyperchloremia, may have an advantage over saline and are recommended for patients undergoing surgery. Recent data suggests that starch-based colloids may increase the incidence of renal failure and need for renal replacement therapy, especially with increasing doses and it would seem logical to limit the use of this colloid [22].
The hemodynamic changes associated with FHF are fairly predictable. As stated above, profound systemic vasodilatation is followed by a compensatory increase in cardiac output. Blood pressure is often low despite aggressive fluid resuscitation. Endpoints in resuscitation are difficult to define and so the use of some sort of monitor of both cardiac preload and cardiac output can be used to observe response to interventions. There is little evidence to suggest any technique is superior to any other and so local experience should dictate which techniques are used. The use of static measures of preload and therefore adequacy of resuscitation, such as central venous pressure (CVP) and pulmonary artery occlusion pressure (PAOP) can be unreliable and are better supplemented by dynamic measurements, which are better at predicting the response to volume resuscitation in the critically ill. Measurement of stroke volume variation (SVV), pulse pressure variation (PPV) and even the cardiac output response to passive leg raising have all been found to be useful in predicting the response to transfused volume [23–25].
Despite 20 years of clinical trials, the role of the pulmonary artery catheter (PAC) in the management of the critically ill patient is still not settled and remains controversial. In 1996 Connors found in a retrospective case control study that the use of PAC in the initial management of critically ill patients during the first 24 hours appeared to increase mortality and costs [26]. Since that study, two randomized trials examining the clinical utility of PAC have been published. The first study (PAC-Man) reported the outcome of critically ill patients randomized to management with a PAC or no PAC/other cardiac output monitor. There were no differences in hospital mortality between the groups and the conclusion of the study was that PAC did not affect survival in critically ill patients. However, in the PAC group there was a 10% incidence of non-fatal complications [27]. A1d In a post hoc analysis of the same study, no difference could be found between PAC and other forms of cardiac output monitoring: suggesting that this form of invasive hemodynamic monitoring was either ineffective or that the information produced was not being interpreted correctly [28]. The use of PAC within a rigid treatment algorithim was examined in a recent randomized trial in patients with acute lung injury. In this randomized study of 1000 patients, the role of PAC compared to a central venous catheter within a fluid liberal or conservative management strategy was assessed. No overall mortality difference was seen in any of the groups and in particular no benefit of PAC insertion was seen [29]. A1c Taken together, data from these trials suggest that the PAC has no role in the routine management of critically ill patients and the decision to insert a PAC must be made on a case-by-case basis.
During the early 1970s, it was first suggested that during critical illness a pathological supply-dependency line is seen (see Figure 41.2) [30]. This was proposed because of markedly increased resting oxygen consumption noted in critical illness associated with systemic inflammation. Oxygen delivery increases to meet the demand and it was noted that survivors had higher oxygen transport parameters than non-survivors did. It was also noted that if oxygen delivery was increased, by fluid resuscitation and/ or inotropic drugs, oxygen consumption increased as well, suggesting that covert tissue hypoxia exists and that this may be the cause of multiple organ failure.
Work by Bihari and Gimson in the 1980s suggested the presence of a pathological supply-dependency for oxygen in patients with FHF. The patients with FHF who failed to survive had both a lower baseline VO2 than survivors and greater increases in VO2 following infusion of epoproster-nol, suggesting a greater oxygen debt [18]. However, since then the whole premise of this argument, that there exists a pathological supply dependency in critical illness, has been questioned because of the inevitable increase in calculated oxygen consumption when delivery is increased due to mathematical coupling [31]. Furthermore, it is clear that not all patients with critical illness have pathological oxygen supply dependency. Early studies evaluating the use of strategies to increase oxygen delivery to supranor-mal levels in an attempt to decrease organ failure did not succeed and in some reports were actually harmful [32]. From other studies done earlier in the course of septic shock, however, it appears that the early (i.e. less than six hours) institution of a protocol to increase oxygen delivery reduces mortality [33]. The interpretation of the above data with relevance to FHF must be cautious. However, given the similarities between FHF and septic shock it would seem reasonable to assume that early vigorous volume resuscitation is appropriate, but routine targeting of supranormal oxygen delivery should be avoided.
Adrenaline and noradrenaline are effective agents and are frequently employed to improve MAP in FHF, commencing at 0.1 μg/kg/min. Both of these agents have been shown to improve MAP, significantly the addition of epo-prosternol (a microcirculatory vasodilator) to noradrenaline increases oxygen delivery while maintaining MAP [34]. Epinephrine, like other beta agonists has deleterious effects on intermediary metabolism if used over a period of time. These effects include hyperlactemia and hypergly-cemia. This is related partly to the effect of epinephrine on glycolysis within skeletal muscles [35]. Norepinephrine does not have these effects and so is recommended on current evidence.
Blood pressure is important in maintaining flow to essential organs. What pressure is acceptable in FHF is unknown. However, cerebral autoregulation is disturbed in FHF, making cerebral blood flow directly proportional to cerebral perfusion pressure [36]. This implies that hypotension will result in cerebral ischemia, which may be a factor precipitating brain swelling in FHF [37], and that hypertension will result in cerebral hyperemia and increased ICP. Although, as discussed later, targeting a specific blood pressure to maintain cerebral perfusion in the face of cerebral edema is often futile and probably unnecessary [38]. B4
Alternatives to norepinephrine or epinephrine to maintain blood pressure, such as terlipressin or vasopressin, have not been systematically evaluated in FHF. Terlipressin is widely used in patients with chronic liver disease and hepatorenal syndrome and has been used in cathecho-lamine refractory septic shock [39–41]. Whilst it is evident that terlipressin is effective at reducing the need for cathe-cholamines in septic shock states its use in patients with FHF may be compromised by its effects on cerebral blood flow and intracranial pressure. Shawcross et al. showed in a small study of six FHF patients with grade 4 encepha-lopathy, that a single dose of terlipressin (0.25 mg) caused a significant increase in cerebral blood flow (CBF) from a median of 69 (range 48–83) ml/100g/min to 81 (range 62–97) ml/100g/min (p = 0.016) accompanied by a significant increase in intracranial pressure (ICP) from a median of 15mmHg (13–18) to 20mmHg (16–23), which was sustained for three hours post terlipressin administration. There was no significant change in systemic hemodynam-incs to explain these findings and the authors hypothesized that terlipressin affected CBF via an interaction with cerebral V2 receptors [42]. In a separate study, the effect of terlipressin (1mg bolus) was examined in ten patients with FHF. The effects on intracranial pressure, cerebral blood flow and cerebral perfusion pressure were compared to norepinephrine. Surprisingly, given the findings of the previous study, the authors found that a 1 mg dose of terlipressin did not increase ICP or CBF despite increasing cerebral perfusion pressure (CPP) through increases in mean arterial pressure. B4 When the effect of increasing the rate of norepinephrine infusion was studied, there were significant increases in both CBF and ICP. The findings of this study show that terlipressin can increase CPP without increasing CBF, suggesting that terlipressin restored cerebral blood flow autoregulation through an unknown mechanism [43]. The reason for these disparate findings is not clear and highlights the need for further studies in this area.
Mechanical ventilation
Intubation of the trachea and mechanical ventilation is indicated for several reasons in FHF but not usually for hypoxemia [18]. As patients progress from grade II to grade III encephalopathy, decreasing consciousness can lead to airway compromise with the risk of aspiration. Grade III encephalopathy is often characterized by agitation and aggressive behaviour. Sedation in these patients is required to allow appropriate monitoring and treatment, but requires intubation and ventilation. In patients at risk of intracranial hypertension it is common practice to target a partial pressure of CO2 of between 4 and 5 kPA; this minimizes the risk of increased cerebral blood flow due to increases in CO2. Controlled trials have shown there to be no benefit from routine hyperventilation in FHF in terms of prevention of intracranial hypertension [44]. A1d
As opposed to other causes of systemic inflammatory response syndrome, the lungs are relatively spared early in the course of FHF. However, a proportion of patients progress to multiple organ dysfunction in which lung disease is prominent [45].
The normal lung can tolerate “conventional” ventilation with physiological tidal volumes and low levels of positive end expiratory pressure (PEEP) for extended periods without apparent harm. The situation is different for damaged lungs and particularly so in patients with acute respiratory distress syndrome (ARDS). There is increasing evidence that mechanical ventilation in the setting of ARDS can increase lung injury and negatively impinge on outcome. Ventilator induced lung injury (VILI) encompasses a wide spectrum of damage, consisting of conventional barotrauma, pneumothorax, pneumomediastinum and alveolar damage, and increasing pulmonary edema [46]. Recent controlled clinical trials have shown improvements in mortality with a protective ventilatory strategy [47, 48]. A1d A recent consensus conference has recommended certain steps to minimize damage to the lungs during mechanical ventilation [49]. A1c
- Minimize the inspired oxygen level and take aggressive steps to do this if the inspired fraction is greater than 0.65.
- Recruit alveoli by increasing PEEP. The amount of PEEP necessary to prevent cyclic opening and closure of alveoli is approximately 10–15 cmH2O.
- Minimize high airway pressures. Transalveolar pressures should not exceed 25–30 cmH2O. This corre sponds to an end inspiratory static (plateau) pressure of 30–40 cmH2O.
- Prevent atelectasis by employing larger breaths periodi cally to re-expand collapsed units during tidal ventilation with small tidal volumes.
Patients who spontaneously recover from FHF or who are transplanted may need mechanical ventilation for a prolonged period of time and often require tracheostomy to facilitate weaning from the ventilator. A recent prospective study has shown that the percutaneous approach is safe in patients with liver disease, even with refractory coagulopathy and should be the method of choice [50]. B4
Sedation and paralysis
Patients with acute hepatic failure requiring mechanical ventilation are deeply encephalopathic. The need for sedation varies between patients and should be tailored individually, the primary aim being relief from pain and tolerance of the ICU environment and interventions. Mechanical ventilation is usually tolerated with minimum amounts of opiate and little if any hypnotic agent. The effect of sedation should be continuously evaluated to ensure that aims are achieved and over sedation avoided, although it should be noted that scoring systems, for example the six-point Ramsay scale have not been validated in FHF and are difficult to interpret in the setting of hepatic encephalopathy [51]. Deep sedation is unnecessary and will only add to cardiovascular depression and prolong recovery in patients with impaired liver function. There are no randomized data to support the use of one sedative agent over another in the routine management of FHF, but Propofol may have some advantage over benzodiazeopines as it has been used to reduce ICP in brain-injured patients and may reduce cerebral blood flow through the reduction in cerebral metabolic rate for oxygen [52, 53]. These considerations, however, have to be contrasted with the need to prevent surges in intracranial pressure during routine nursing care; supplemental sedation or small doses of non-depolarizing neuromuscular blocker may be useful during suctioning of the patient’s trachea.
The issue of paralysis in FHF should be considered. It had been common practice to paralyze all ventilated patients with FHF whilst at risk from cerebral edema. However, there have not been any controlled clinical trials comparing paralysis or not in FHF, and, for that matter, in any other branch of intensive care medicine. A recent retrospective review of 1030 patients with acute traumatic brain injury showed that ICU stay and infectious complications were higher in the group who received routine paralysis [54]. B4 Anecdotal reports have also suggested an association between long-term paralysis and a necrotizing myopathy, in patients with asthma, that may prolong ICU stay and impinge adversely on outcome [55]. Thus, there is no indication for routine paralysis in FHF. B4
Nutrition in FHF
There are no randomized trials concerning nutrition in FHF, but in common with other critical illnesses FHF is characterized by increased energy requirements and given that the majority of patients will be sedated and ventilated, this energy must come from supplemental feeding [56]. Initially, the majority of FHF patients will be fed nasogas-trically with standard enteral formulae as per international guidelines [57]. A1 c The enteral route, if available, is always superior to the parenteral route, assuming that adequate feed can be given. There is no difference between gastric and post-duodenal feeding in terms of adequacy of feeding or infectious complications and therefore, if tolerated, nasogastric feeding is appropriate [58, 59]. In patients who fail gastric feeding and have residual feed volumes, consideration can be given to prokinetic agents, such as meto-clopramide or erythromycin. These drugs are highly effective at stimulating gastric motility and reducing nasogastric aspirates, but the effect can be short-lived and placement of feeding tubes beyond the pylorus is often required [60]. Feeding should be instituted within 24 hours of admission to the intensive care unit and in the initial phases of the illness should be no more than 20–25 kcal/Kg BW/day increasing to 25–30 kcal/Kg BW/day in the recovery phase [57]. A1c Despite a theoretical risk of worsening encephalopathy in FHF, due to the administration of protein, there is no data to support routine protein restriction. In practice, most patients will tolerate protein intake of 1–1.5g/kg/day without complication. Renal failure is a common complication of FHF and the majority of patients will require renal replacement therapy (RRT) during the course of the illness. RRT can cause reductions in important trace elements and vitamins such as copper, selenium, thia-mine and vitamin E, and therefore consideration should be given to trace element and vitamin supplementation during FHF, especially if complicated by renal failure requiring RRT [61]. Some vitamins such as vitamin C and vitamin E have important antioxidant properties and supplementation with these vitamins has been studied in general critical care populations with some benefits [62, 63]. The use of immuno nutrition (feeds supplemented by compounds such as arginine, glutamine and fish oil) has not been studied in FHF. Individual trials have shown benefit in some subsets of critical care patients and glutamine supplementation is considered a standard of care in parenter-ally fed patients [64]. B4 Further studies are needed in this area, particularly in relation to FHF.
Hypoglycemia is a feature of advanced liver failure due to lack of gluconeogenesis from the failing liver. Whilst it is not uncommon for FHF patients to require administration of glucose containing solutions to maintain blood glucose levels, many patients will need insulin to control elevated blood glucose levels. In 2001, Van den Berghe reported a reduction in mortality in surgical ICU patients treated with intensive insulin therapy to maintain blood glucose levels between 4.4 and 6.1 mmol/l [65]. This approach has not been evaluated in FHF patients and there is concern that such tight glycemic control may predispose to adverse events related to hypoglycemia. A larger study has recently been published concerning blood glucose levels in critically ill patients. This study actually showed a significantly increased mortality in patients with tight glucose control compared to a more liberal regimen [66]. A1c
Stress ulcer prophylaxis
Many small randomized controlled clinical trials over the past 20 years have looked at the prevention of stress ulcera-tion. While the incidence of stress ulcer has fallen over this period, the cause of this decline is unclear. It is probably the result of both improved resuscitation and the widespread use of stress ulcer prophylaxis. The most recent large study estimates the incidence of clinically important stress ulcer bleeding to be about 3% in general ICU patients [67].
Stress ulceration is probably the result of ischemic injury to the gastric mucosa, and adequate resuscitation is the single most important factor in its prevention. Apart from good general ICU care, there have been two broad approaches to reducing the incidence of stress ulceration: decreasing the acidity of the stomach with the use of antacids, H2-blockers, or proton pump inhibitors; and the use of sucralfate, a cytoprotective agent. The role of acid suppression in encouraging an increase in bacterial overgrowth and the ensuing micro-aspiration of colonized pha-ryngeal fluid, thus promoting the development of hospital-acquired pneumonia, has led to the comparison of the ulcer, pneumonia and mortality rates between the two methods.
Recently, several meta-analyses have attempted to resolve the uncertainty regarding efficacy on one hand and adverse effects of the drugs on the other [68, 69]. After combining their efforts, the two main groups of investigators published a meta-analysis which included all relevant published and unpublished randomized clinical trials [70]. The meta-analysis demonstrated similar efficacy for H2-blockers and sucralfate for the outcome of reduction in stress ulceration bleeding, but an increase in the incidence of pneumonia and an excess mortality in the H2-blocker group. A1a A more recent trial conducted by some of the same authors suggests a significantly higher rate of stress ulceration with sucralfate compared to ranitidine, without any difference in pneumonia or mortality rates [70]. A1d
The aim of stress ulcer prophylaxis is to increase the pH of the gastric lumen and therefore reduce acid related damage. Therefore, it would seem logical that proton pump inhibitors would be more efficacious in preventing bleeding. In randomized controlled trials proton pump inhibitors appear to cause more durable increases in gastric pH, but evidence that they are more effective in preventing clinically significant GI bleeding than H2 receptor antagonists is lacking, although adverse events were similar between groups [71, 72]. A1c
FHF was excluded in most of the trials comparing sucralfate to pH-altering drugs and was not included in the meta-analysis. It is therefore difficult to make firm conclusions. Patients with FHF tend to fall into the high-risk group by virtue of both being ventilated and having a coagulopathy. The balance of evidence suggests that pH-altering drugs such as H2-blockers or proton pump inhibitors provide the best defense against stress ulceration, but that this may be offset by an increased incidence of pneumonia. Current guidelines on the management of septic shock advise the use of either H2 RA or proton pump inhibitors for the prevention of bleeding and it would appear reasonable to extrapolate this guidance to patients with FHF [73]. B4
Infection, prophylactic antibiotics and selective decontamination of the digestive tract
Patients with FHF are prone to infection. Early studies of FHF patients reported an incidence of bacterially proven infection in 80% of patients and fungal infection in 30% [11]. Initial analysis of isolates from infected FHF patients showed that the most frequently cultured organism was coagulase negative staphylococcal species [74]. However, it is clear from more recent studies that the epidemiology of infection in FHF patients has changed. In a recent retrospective review of over 200 patients with FHF Karvellas et al. have reported that whilst 34% of patients will at some point develop bacteremia during ICU admission, this occurred later in the course of the illness (median time to bacteremia ten days) than in the original studies. Moreover, the most frequently cultured organisms were Gram-negative organisms such as E. Faecium, Klebsiella spp. and Vancomycin resistant enterococcus [75]. The underlying reasons for this change in epidemiology are not clear, but the widespread use of prophylactic antibiotics in patients with advanced liver failure must have an impact on the timing of the development of infection and the prevalence of resistant strains such as Acinetobacter spp.
The predisposition to infection displayed by FHF patients is multifactorial. These patients often have multiple vascular access devices and may undergo prolonged ventilation and renal replacement therapy, all of which are well-known risk factors for the development of bacteremias in the critically ill [75, 76]. In addition to risk factors related to interventions, patients with FHF also appear to have important immunological defects which predispose to infection and have a prognostic importance. Neutrophils from patients with FHF have been shown to be deficient in killing and phagocytic ability; defects which are reversed in vitro by the administration of G-CSF [77, 78]. Recently, Antoniades et al. have characterized the function of mono-cytes from patients with acetaminophen induced liver failure. Monocytes are important orchestrators of the immune response, (and) have a critical role in protection from infection and show marked deactivation in patients with FHF from acetaminophen [79]. In particular, the density of surface HLA-DR on monocytes was shown to have prognostic ability in reference to acetaminophen induced FHF patients [80].
Inflammation induced by infection also appears to impact upon the severity of hepatic encephalopathy. The presence of components of the systmemic inflammatory response syndrome (SIRS) appears to predict the severity of HE. The mechanism for this interaction is incompletely understood but it appears to be due to increased cerebral blood flow [81].
Because of the high incidence of infection and the underlying immunological defects in FHF, the use of prophylactic antimicrobial agents has been investigated. Both parenteral antibiotics and the use of selective decontamination of the digestive tract (SDD), in combination and individually, have been studied.
Intravenous antibiotics, if given prophylactically will reduce the incidence of infection in patients with FHF to approximately 20% [82, 83]. B4 However, the use of prophylactic antibiotics has not been shown to improve outcome or reduce the length of stay in patients with FHF [83]. The role of SDD is less clear and has not been evaluated in controlled trials compared with placebo or intravenous antibiotics alone in FHF. Rolando reported that SDD used in combination with intravenous antibiotics provided no additional benefit [82–84]. B4
The most recent systematic review of randomized controlled trials of antibiotic prophylaxis in intensive care units was published in the Cochrane database of systematic reviews [85]. This systematic review evaluated 32RCTs, which included 5639 unselected general ICU patients. Selected groups, for example patients with FHF, were excluded from the review. Pooled estimates of the 16RCTs testing the effect of the SDD and systemic antibiotic combination indicate a significant reduction of both respiratory tract infections (OR 0.35; 95% CI: 0.29–0.41) and total mortality (OR 0.80; 95% CI: 0.68–0.93) (Figure 41.3). A1a The number needed to treat to prevent one infection is five, and the NNT to prevent one death is 23. When the data on the effect of SDD alone compared to control were pooled from the 16 available trials, a marked reduction in respiratory tract infections was demonstrated (OR 0.56; 95% CI: 0.46–0.68) but no corresponding effect on overall mortality (OR 1.01; 95% CI: 0.84-1.22) was found (Figure 41.4).
Although prophylactic intravenous antibiotics have been shown to reduce the number of proven infections in FHF, improvements in outcome have not been demonstrated. SDD on its own has not been shown to reduce infection or improve outcome in FHF. B4 There is also a risk of promoting the emergence of multiply resistant organisms within intensive care units by the blanket use of broad spectrum antimicrobials. SDD selects for an increase in Gram-positive organisms, especially methicillin resistant staphylococcus (MRSA) and vancomycin resistant enterococcus (VRE). In a large single center randomized trial the use of SDD was not associated with the development of resistant strains such as VRE and MRSA. However, a follow-up study suggested that SDD promoted the development of extended spectrum beta-lactamase producing strains [86, 87]. Future research should be aimed at determining the cost-effectiveness of SDD, with inclusion of estimates of the effects of the emergence of resistant microorganisms. However, for the individual patient the evidence in favor of the use of prophylactic antimicrobials is compelling.
Figure 41.3 (a) and (b) Antibiotics for preventing respiratory tract infections (source: (Cochrane Review). In: The Cochrane Library, issue 2, 1999. Oxford: Update Software).
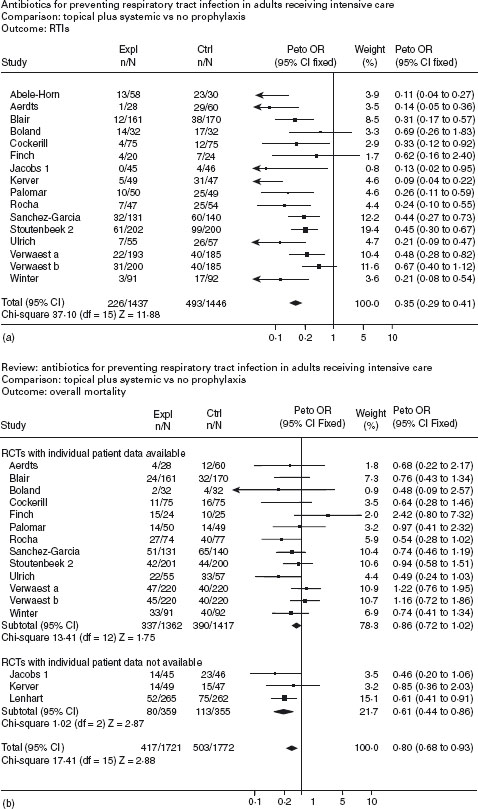
Figure 41.4 (a) and (b) Antibiotics for preventing respiratory tract infections (source: Cochrane Review) The Cochrane Library, issue 2, 1999. Oxford: Update Software).
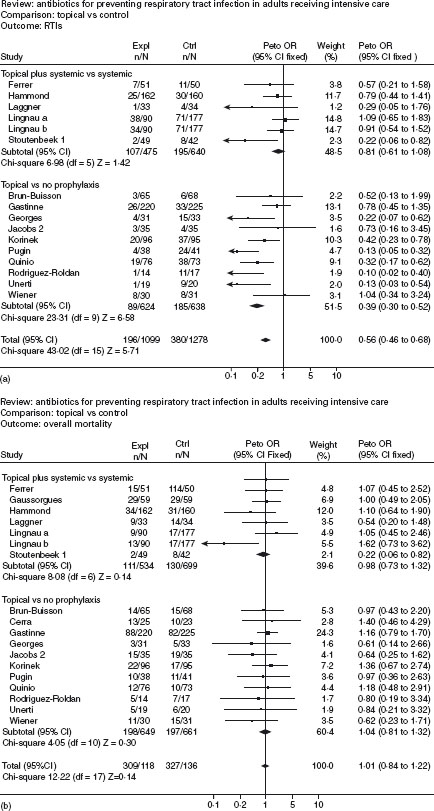
Management of cerebral edema
Hepatic encephalopathy (HE) is always present in FHF and although the pathogenesis has not been fully elucidated, considerable evidence points to a central role for ammonia as a major factor. The ammonia hypothesis is supported by extensive animal data and clinical studies correlating the level of ammonia with onset and severity of intracranial hypertension (ICH) [88–90]. Recent studies have suggested a role for infection and inflammation in the pathogenesis of HE, with evidence that the molecular mediators of infection and inflammation may act synergistically with ammonia to produce HE [81, 91–93].
Infection is a common precipitant of HE in both chronic liver disease and FHF. Large studies have demonstrated the role of the systemic inflammatory response syndrome (SIRS) and infection in the progression and severity of HE and other complications of liver failure [75, 92–94]. ALF is an inflammatory state and increased levels of the pro-inflammatory cytokines TNF-α, IL-6 and IL-1 β can be demonstrated, especially in patients with raised ICP. The generation of these pro-inflammatory cytokines may be due to activation of the immune system by infection and also release from the necrotic liver [91].
The generation of cerebral edema in HE is dependent on blood ammonia, with entry into the brain increased in ALF, and serum levels > 150–200 μmol/l found to predict intracranial hypertension [89, 90]. Ammonia is detoxified in the brain by formation of glutamine in astrocytes from amida-tion of glutamate. Glutamine accumulation in the astrocyte causes osmotic swelling, and cellular dysfunction through induction of oxidative stress and mitochondrial permeability transition [95, 96]. Indeed inhibition of glutamine syn-thetase ameliorates brain edema and improves the survival in animal models of ALF [97]. Astrocytic swelling thus contributes to the elevation of ICP in addition to the other component of intracranial hypertension in FHF, which is increased cerebral blood flow (CBF), although this has not been consistently demonstrated. Vascular autoregulation within the brain is defective in patients with ALF, with uncoupling of blood flow and cerebral metabolic rate [36, 98]. Cerebral hyperemia has been shown to be contributory to an increase in ICP in animal models based on porto-caval anastomosis and ammonia infusions [99], and an increase in cerebral blood flow has been shown in some human studies in ALF [100], but not in others [37, 101]. Indeed, studies have shown a wide variation in cerebral blood flow in patients with ALF and an increase in cerebral lactate production has been shown, indicating possible ischemia that may induce cerebral swelling [37]. Recent work has shown that in patients with elevated ICP, CBF was increased and associated with the presence of SIRS criteria, markers of inflammation (C-reactive protein, white cell count) and elevated levels of the pro-inflammatory cytokines TNF-α and IL-6. Moreover, patients who developed surges in ICP also had associated increases in CBF and inflammatory markers [102]. The blood brain barrier is thought to remain intact in ALF and thus it seems unlikely that circulating cytokines can have a direct effect on cerebral function, although a recent small series has shown net efflux of inflammatory cytokines from the brain, suggesting that the blood brain barrier may be impaired in some way as has been shown in experimental animals [91, 103].
The above studies lead to the proposal of a “two hit” hypothesis, whereby the initial event in the precipitation of HE is raised ammonia levels consequent upon liver failure, which causes astrocyte swelling and cellular dysfunction, setting the stage for the worsening of ICP via increased cerebral blood flow in the setting of a concurrent inflammatory state.
Intracranial pressure monitoring
The use of intracranial pressure (ICP) monitors in FHF has not been subjected to a randomized controlled trial. As with any monitor used in critical illness, finding a positive outcome related to their use is difficult. At best, studies have suggested they may help with the management of patients with raised ICP. One study using historical controls suggested greater interventions associated with their use, and assuming the interventions were appropriate, this may be of benefit. The duration of survival from the onset of grade IV encephalopathy was significantly greater in the ICP monitored group (median 60 vs 10 hours, p < 0.01), although overall survival was unchanged [104]. Blei et al. performed a postal survey of complications in 262 patients from liver transplant centers across the USA [105]. Epidural transducers were the most commonly used devices and had the lowest complication rate (3.8%); subdural bolts and parenchymal monitors (fiberoptic pressure transducers in direct contact with brain parenchyma and intraventricular catheters) were associated with complication rates of 20% and 22% respectively. Fatal hemorrhage occurred in 1% of patients undergoing epidural ICP monitoring, whereas subdural and intraparenchymal devices had fatal hemorrhage rates of 5% and 4% respectively. They concluded that epidural transducers were the safest form of monitoring even if not the most accurate. A similar survey of 25 centers contributing to the US Acute Liver Failure Study Group was recently performed by Vaquero et al. [106]. In this study, only 27.7% of patients with grade III or IV encepha-lopathy had an ICP monitor placed. Patients with ICP monitoring were more likely to be younger and be listed for liver transplantation. There was considerable heterogeneity amongst centers regarding the frequency of use and the type of monitor used. In this study subdural monitors were used most commonly. Similar to the earlier study by Keays et al. the use of ICP monitoring was associated with more interventions such as mannitol, barbiturate and vaso-pressor administration. There was no difference in survival or neurological outcome between the two groups, although only patients who underwent transplantation were compared. Complications of insertion were seen in 10% of patients, all of these events being related to intracranial bleeding. In three patients intracranial bleeding was clinically insignificant, but in two patients bleeding may have contributed to mortality. Whilst the complication rate appears to be lower than the previous study by Blei et al.
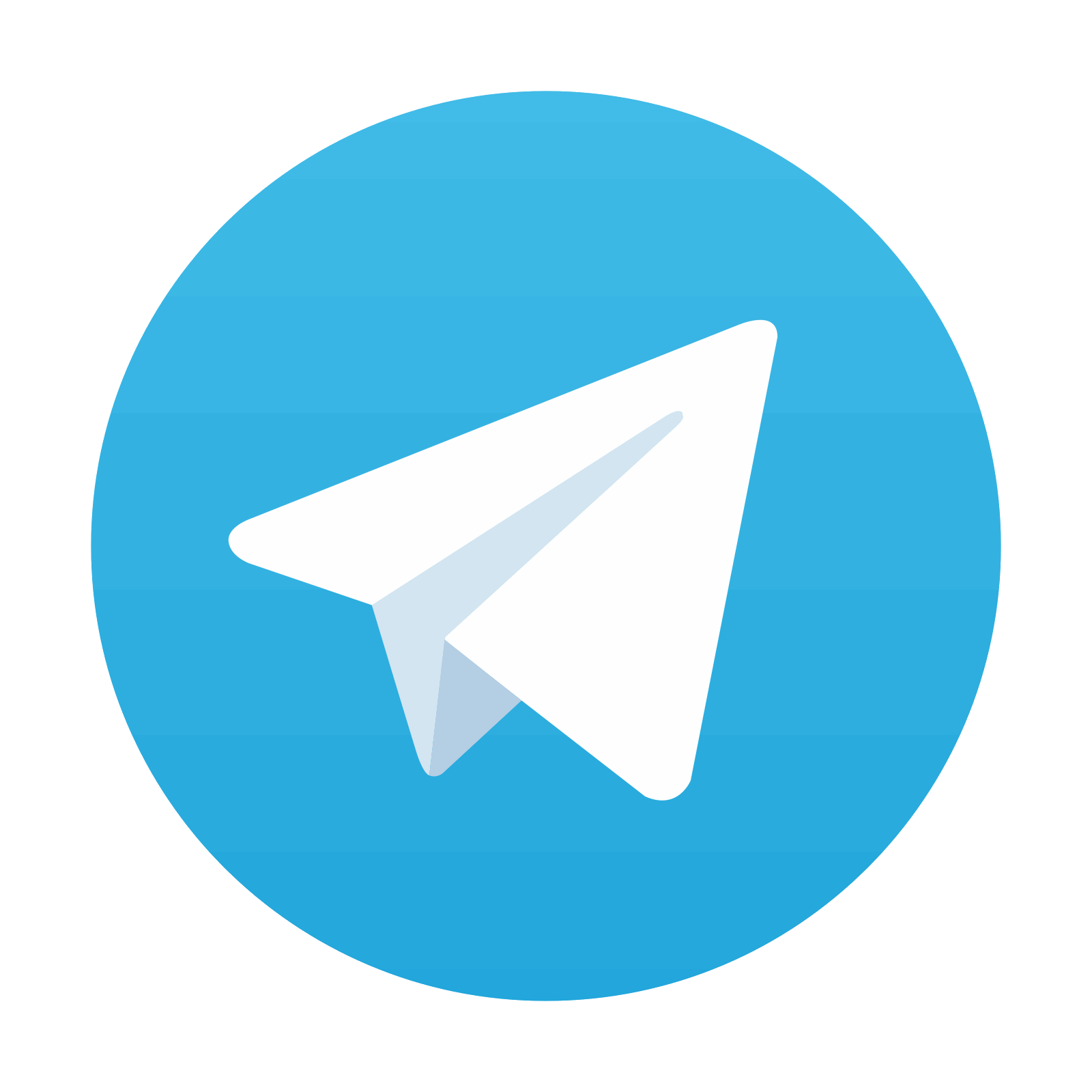
Stay updated, free articles. Join our Telegram channel

Full access? Get Clinical Tree
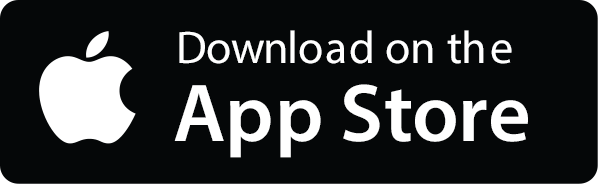
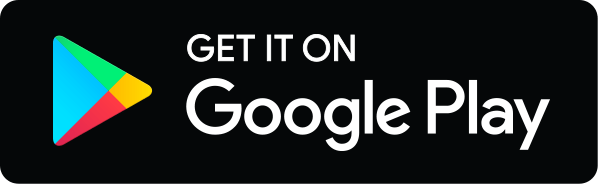