From the earliest days, advances in extracorporeal membrane oxygenation (ECMO) have been linked to advances in cardiopulmonary bypass (CPB) and repair of congenital cardiac malformations. Since C. Walton Lillihei1 first employed the technique of controlled cross-circulation using the patient’s parent, efforts to perfect cardiopulmonary support have transitioned from the operating room to the intensive care unit. In the intervening years, every facet of CPB has been studied and refined. Improvements in these techniques have afforded the surgeon the opportunity to tackle the most complex anatomic anomalies in even the smallest babies. Likewise, advances in extracorporeal support (ECLS) methods have led clinicians to expand their clinical indications and implementation in the ICU. However, despite the fact that the notion and use of extracorporeal circulation originated in the cardiac surgical suite, heart disease was, until the last decade or so, usually a contraindication for ECMO. Early experience in adults was discouraging,2 with better success reported in the care of newborns with respiratory failure.3 Extension to include older infants and children with respiratory failure followed.4
Pediatric cardiac ECMO, as it has come to be known, was first reported by Soeter and colleagues in 1973. He described the successful application of ECMO in a 4-year-old girl following repair of tetralogy of Fallot, who was weaned from support in 48 hours and discharged home 11 days later.5 By the late 1980s and early 1990s, cardiac ECMO gained traction in the pediatric cardiac community, as many large centers reported favorable outcomes with postoperative support. With experience, refinement, and successes, cardiac ECMO has made the transition from “rescue” therapy, to “therapeutic” or even “preventive” management. Coincident with advances in pharmacologic and mechanical ventilatory support strategies for respiratory failure, the number of ECMO runs for the cardiac population has steadily increased as a proportion of the Extracorporeal Life Support Organization (ELSO) Registry (Fig. 87-1). In this chapter, we take a comprehensive look at cardiac ECMO, with attention to the different types (venoarterial or venovenous), their relative indications, and their respective advantages, disadvantages, and outcomes. In addition, we discuss the myriad equipment options and also review the physiology of ECMO and principles of clinical application. Last, we consider new opportunities and indications for mechanical circulatory support.
In simplest terms, venoarterial ECMO (VA-ECMO) consists of taking deoxygenated blood from the venous circulation, oxygenating it, and then pumping it back into the arterial circulation. How this is accomplished depends on individual and institutional experience and preference. The obvious benefit is that the patient’s entire cardiorespiratory system is bypassed, thereby ensuring adequate end-organ oxygenation despite respiratory and circulatory collapse. Ideally, the brain, kidneys, and other organs thereby maintain their essential functions in a circumstance that would otherwise almost surely lead to multi-organ failure and possibly death. Importantly, this support system likely creates an environment that is more conducive to functional improvement. There are ample data to support this assertion with regard to the lungs, and advances in the understanding of the pathogenesis of cardiac failure suggest that the same is likely true for the heart.7
Broad indications for VA-ECMO include preoperative stabilization, postcardiotomy support, bridge-to-transplant, cardiopulmonary arrest, myocarditis and cardiomyopathy, pulmonary hypertension, intractable arrhythmias, and occasionally, respiratory failure refractory to venovenous extracorporeal circulatiry support.
Among neonates and pediatric patients, congenital heart disease is by far the most common indication for VA-ECMO, accounting for 72 percent of all cases, and 86 percent of neonatal (age <31 days) cases.6 In neonates with congenital cardiac malformations, indications for VA-ECMO can arise soon after birth. The infant with obstructed total anomalous pulmonary venous drainage is the classic example of a newborn that can present in extremis and require emergent institution of VA-ECMO. Ideally, with VA-ECMO support, the baby’s clinical condition can be optimized prior to surgical correction. Similarly, some neonates with congenital cardiomyopathy (i.e., dysrhythmias) will benefit from support while diagnostic studies and therapeutic interventions, such as radiofrequency ablation, are pursued. Without ECMO, these babies might die before a diagnosis is established and therapies implemented.
Postoperative instability is the most common indication for VA-ECMO in the cardiac setting. Whether due to prolonged CPB or circulatory arrest times, complex surgical repair and manipulation of the heart, exaggerated inflammatory response to CPB, or inadequate myocardial protection, the availability of VA-ECMO is often lifesaving as a bridge to recovery. Of course it is critical that residual structural lesions be ruled out as the cause for compromise; thus a thorough assessment (i.e., transesophageal echocardiogram) is imperative before initiating VA-ECMO. Standard postoperative ECMO must be distinguished from rapid or resuscitative ECMO (eCPR), a topic covered later in the chapter.
In 1987, Kanter and colleagues were the first to report some success with ECMO in patients who had undergone cardiotomy, with 6 of 13 surviving to discharge.8 Since then, the literature is replete with institutional reports of ECMO use in patients with structural cardiac anomalies. Morris and coworkers at the Children’s Hospital of Philadelphia (CHOP) reported their experience with 137 patients on cardiac ECMO9 between 1995 and 2001. Diagnoses encompassed the spectrum of congenital cardiac malformations, including single ventricle with intracardiac mixing and single ventricle with separate circulations, as well as children with structurally normal hearts. Overall survival to discharge was 39 percent. Two-thirds of the patients had undergone cardiac surgery prior to ECMO, with 40 percent of those surviving to discharge. In that subset, risk factors for mortality included age less than 1 month, male gender, duration of mechanical ventilation before initiation of ECMO, and the development of renal or hepatic dysfunction on ECMO. Failure to separate from CPB was not found to be a predictor of death, which means that in those cases ECMO was literally life-saving. In addition, despite historical bias against placing patients with single ventricle physiology on ECMO, this factor did not prove to be significantly associated with death in this series. No factor analyzed was predictive of mortality in the subset of patients who had not undergone surgery and in whom survival to discharge was 35 percent. Overall, considering the fact that many of the survivors likely would have died without mechanical support, the authors concluded that, “it is imprudent to impose strict indications or contraindications to ECMO support.”
Sivarajan and colleagues reported another large study of 116 patients supported with cardiac ECMO from Royal Children’s Hospital in Melbourne. They included data from patients undergoing conventional ECMO as well as patients cannulated during eCPR. Survival to hospital discharge improved from 33 percent in the earlier era (1990–2001), to 48 percent in the recent era (2002–2006).10 Another relatively large series from the Great Ormond Street Hospital in London reported on 81 cardiac patients managed with ECMO between 1992 and 2000.11 More than half of these patients were placed on ECMO in the OR following multiple unsuccessful attempts at separation from CPB. Overall survival to discharge was 49 percent. Their report includes only patients with two-ventricle anatomy. Patients placed on ECMO in the surgical suite had a 64 percent survival to discharge, as compared to 29 percent in those patients cannulated in the ICU. They found, in agreement with many previous studies, that renal failure was a significant risk factor for mortality. Also, as with virtually all other reports of pediatric cardiac ECMO, their data support the contention that a residual cardiac lesion bodes poorly for outcome and is a contraindication for initiation of support. In our experience, however, there are situations where a patient can be supported for a period of hours or even days, subsequently return to the operating room or the interventional cardiology suite for correction of residual lesions, and then be weaned from ECMO; so even this contraindication is a relative one.
VA-ECMO can also be utilized for postoperative support following heart transplantation, either for the treatment of pulmonary hypertension or early graft dysfunction. Galantowicz and Stolar12 reported 40 percent salvage rate among children requiring mechanical support following transplantation. Rarely, acute rejection episodes occurring remotely that are marked by severe myocardial dysfunction can be managed with VA-ECMO until the initiation of antirejection therapy.13
VA-ECMO can also be indicated in neonates and pediatric patients with cardiac failure waiting for transplantation, or more rarely, as an intermediate step prior to implantation of a ventricular assist device14. A recent series by Imamura et al.15 at Arkansas Children’s Hospital reported 43 percent mortality among 21 patients supported with ECMO as a bridge-to-transplant. The ELSO registry reported similar data, with 51 percent mortality for pediatric patients supported with ECMO prior to transplantation.16
Data from a large single-institution study from CHOP had comparatively better outcomes. These authors described 31 patients supported with ECMO as a bridge to cardiac transplantation.17 No difference in long-term survival was found between heart transplant recipients who were supported with ECMO prior to transplant versus those who were not. Of the 31 patients, 6 had sufficient recovery of function to be weaned from ECMO and discharged home (“bridged to recovery”), while 12 of the original 31 ultimately underwent cardiac transplantation. Overall survival to discharge was 48 percent (15 of 31).
The CHOP experience does not support the enforcement of an arbitrary cutoff for duration of support, as they have had several patients survive after more than 250 h on ECMO, including four who were successfully transplanted after 500 h of support and one who survived 1126 h before transplant. Furthermore, as others have reported, pre-ECMO cardiac arrest was not associated with poorer outcome.18–20
For patients requiring ECMO after cardiac transplantation, Kirshbom and coworkers17 reported 22 percent survival to discharge in those patients requiring support soon after transplantation as compared to the three patients who required support in the late period; two of the latter survived to discharge. They concluded that ECMO can be an effective tool for management of the peritransplant patient.
Morales and colleagues reported the initial multicenter experience with the Berlin Heart EXCOR ventricular assist device in 73 patients from 17 institutions.14 VA-ECMO was used as a bridge to EXCOR in 22 patients (33 percent). Though the overall mortality for the cohort was high at 24 percent, patients supported with ECMO prior to device implantation were not an increased risk for death. ECMO was therefore used successfully as a bridge-to-bridge in these patients. ECMO could also be applied as a “bridge-to-decision” in neonates in whom the decision to proceed with EXCOR implantation or heart transplantation is unclear for social, neurologic, infectious, or other reason. This is similar to the paradigm for application of short-term mechanical circulatory assist devices in older patients and adults.
eCPR refers to the application of ECMO as an immediate life-sustaining therapy in the setting of cardiopulmonary arrest. Most centers consider activation of an eCPR protocol after 10 min of resuscitation, or after two rounds of “code” drugs have been administered without success.21 John Gibbon, the pioneer of CPB, speculated in 1939 on the ability of a heart–lung machine to temporarily take over the function of the heart and lungs during a period of deterioration and provide a time interval for the recovery of organ function, leading to potential survival.22 eCPR has evolved from early reports of use following myocardial infarction, pulmonary embolism, and other emergency conditions.23–26
The acceptance of eCPR as a viable therapeutic intervention has been largely based on the study by Duncan and colleagues,27 demonstrating that patients cannulated for eCPR had equivalent survival to patients cannulated for conventional cardiac ECMO. Its current form is the result of a slow iterative approach in the design and development of both the equipment and management principles over the past 20 years.
Though specific components may differ among institutions, successful application of eCPR generally requires the coordinated 24-hour involvement of surgical, nursing, perfusion, and ICU staff, as well as a modified ECMO circuit that can be primed and deployed rapidly (ideally within 15 min). A state-of-the-art rapid deployment circuit ideally would include a preassembled completely heparin-coated (Carmeda® Bio-Active Surface) surface, a centrifugal blood pump, a new-generation hollow-fiber oxygenator such as the Quadrox® or Minimax® oxygenator (approximately 250 mL priming volume), a Bio-Probe flow probe, and a BioTrend Oxygen Saturation and Hematocrit monitor. The entire circuit can be assembled and primed in under 5 minutes.28 eCPR usually necessitates institution of VA-ECMO, with cannulation sites individualized to anatomy and postoperative status, and often patients are actively cooled for 48 hours to 34°C to lower metabolic demands and provide central nervous system (CNS) protection. Based on current literature, it is unclear whether the duration of conventional CPR should limit the application of ECMO. A recent study from Kane et al. showed no difference in survival based on conventional CPR duration.21 Further, there are data demonstrating survival even after prolonged (>95 minutes) CPR.29,30 On the other hand, however, a study by Sivajaran et al.10 showed a clear survival disadvantage in patients undergoing ≥30 minutes of conventional CPR. It is also likely that other risk factors such as high lactate levels or low arterial pH, which have more consistently been uncovered, could be surrogates for prolonged conventional CPR duration. Neurologic status is intuitively influenced by prolonged CPR, and further studies will be required to elucidate optimam timing and prognostic factors implicit in these outcomes.
Despite the controversies regarding the specific criteria and development of eCPR protocols, there is clear evidence that eCPR is a useful and important strategy to salvage patients who would otherwise die. The study by Kane et al.21 from Boston Children’s Hospital reported their outcomes in 172 patients with cardiac disease undergoing eCPR between 1995 and 2008. They reported a laudable 51 percent survival to discharge, which was independent of anatomy, CPR duration, and location of cardiac arrest. Importantly, 75 percent of the survivors had either no or mild neurologic impairment as measured by pediatric overall performance category (POPC) and pediatric cerebral performance category (PCPC) scores <2. Data from another large series at the Hospital for Sick Children in Toronto,30 which included prolonged conventional CPR duration of up to 95 minutes, also demonstrated the efficacy of eCPR, with 30 percent survival. These authors concluded that the lack of consistent poor prognostic factors in eCPR should prompt aggressive application of resuscitative ECMO in all patients without obvious contraindications. Institution of an eCPR protocol, as mentioned previously, requires a tremendous amount of resources, personnel, as well as a dedicated iterative approach to refining deployment. An additional caveat is that centers offering eCPR should possess both transplant and longer-term mechanical circulatory assistance capabilities as an important (up to 11 percent) of eCPR patients will eventually require one of these exit strategies.21,30
Defining which patients are appropriate candidates for cardiac ECMO support is complicated, and is evolving as the utilization of cardiac ECMO increases. Specific conditions including incurable malignancy, advanced multisystem organ failure, extreme prematurity, and severe neurologic or CNS damage constitute absolute contraindications for the application of mechanical support.31
Based on univariate and multiple logistic regression analysis of retrospectively collected data in 218 patients, Trittenwein and colleagues proposed inclusion criteria related to lactate and central venous oxygen saturations.32 We believe that such criteria are too narrow and not universally applicable. As mentioned above, establishment of rigid inclusion and/or exclusion criteria is problematic; each case requires thoughtful consideration on an individual and institutional basis.
Timing of initiation of ECMO is critically important. Low-cardiac-output syndrome (LCOS)33,34 is common to most scenarios in which cardiac ECMO is considered. Clinically, LCOS manifests as poor peripheral perfusion, oliguria, and lactic acidosis despite adequate intravascular volume and often elevated filling pressures. Mixed venous oxygen saturations are low, secondary to decreased oxygen delivery. After correcting for anemia, hypoxia, or obstructive cardiac disease, traditional therapy for this type of cardiogenic shock focuses on maximizing pharmacologic support. Usual agents act through a variety of mechanisms, but most cause an increase in biventricular afterload and heart rate. The net effect is to increase myocardial work and oxygen demand in a setting where myocardial oxygen supply is already compromised. This can exacerbate injury and delay or even prevent myocardial recovery. With ECMO, unloading the heart and decreasing its work creates an environment that is more favorable for repair and recovery.35 In effect, earlier institution of mechanical support helps to ensure adequate perfusion of the heart, brain, kidneys, and other vital organs while minimizing ongoing myocardial insult and enhancing recovery. Most recent reports have documented better outcomes in those patients placed on ECMO in the OR as compared to those cannulated in the ICU.11,18,36 Along these lines, Shen et al. reported 87 percent hospital survival in 23 patients who were routinely converted directly from CPB to VAD support in the OR after undergoing the Norwood procedure.37 Although many of these patients would likely have survived without circulatory support, the authors assert that some patients might have otherwise developed acute heart failure and therefore benefited from early implementation of mechanical cardiac assistance, especially in the setting of hypoxemia that is associated with all Norwood patients. Research has demonstrated that in the setting of hypoxemia, the brain is extremely vulnerable to diminished cerebral oxygen delivery that can accompany a fall in systemic cardiac output.38
Experience with mechanical support of nonsurgical heart failure also merits consideration. In their series, Morris and associates report 35 percent survival in the nonsurgical population, which included patients with cardiomyopathy and myocarditis.9 Marx and coworkers have reported 67 percent survival in patients supported with ECMO for acute myocarditis and 62 percent survival in patients with cardiomyopathy. Duncan and colleagues report 83 percent survival in 12 pediatric patients with myocarditis who were placed on ECMO.34 Of their overall population (12 ECMO, 3 VAD), 47 percent recovered with return of native ventricular function, and 33 percent survived following successful ECMO bridge to cardiac transplantation.
Survival is not the only marker of a successful ECMO run. Long-term sequelae can be considerable and devastating and should bear considerable weight in any discussion of indications and outcomes. Assessment of neurologic insult directly related to cardiac ECMO is difficult due to the many confounding variables. Cyanotic heart disease alone is, as an example, associated with neurodevelopmental abnormalities.39 –42 Similarly, behavioral and cognitive impairment are well-documented in both adults and children following CPB, as are gross and fine motor deficits.43–45 In addition, follow-up in neonatal respiratory ECMO survivors has revealed neurobehavioral and functional deficiencies.46–54
Acute abnormalities have been described in cardiac ECMO patients at the University of Michigan, as 14 percent had seizures and 7 percent had radiographic evidence of injury to the CNS.18 Hamrick and colleagues report significant long-term impairment in their 11-year experience with infants supported by ECMO following cardiac surgery.55 Of their 53 patients, 7 of the 14 survivors available for long-term follow-up (13 percent overall) were neurologically completely intact.
Golej and Trittenwein have looked critically at the issue of neurologic impairment associated with cardiac ECMO.56 They advocate for early recognition and rehabilitation in this at-risk population. As with most advances in CPB and operating room management, monitoring devices and strategies are crossing over into the ICU (bi-spectral index monitoring and near-infrared spectroscopy). However, pre-ECMO intracranial imaging is not as universally possible in cardiac patients as it is in neonates with respiratory failure. In that population, evidence of preexisting intracranial hemorrhage is a contraindication for ECMO. In cardiac ECMO patients, serial cranial ultrasounds are routinely obtained after return to the ICU, but even these studies lack adequate capability to detect anything less than gross hemorrhage or infarct.55 Refinements in the ECMO circuit and devices have allowed for relative minimization of anticoagulation needs (discussed later), but the risks of hemorrhage and embolic events remain real and may be even more significant on a microvascular level than is currently appreciated.
Placing a patient on ECMO for cardiac support requires a cohesive team of surgeons, perfusionists, ECMO specialists, nurses, and cardiac intensivists. Though conceptually simple, ECMO cannulation is fraught with potential technical complications that are magnified by critical patient condition and need for expeditious completion. Experience and vigilance therefore are critical to ensuring optimal outcomes.
The cannulation strategy for ECMO must be based on the type of support to be provided (VA-ECMO vs VV-ECMO), the age and weight of the patients, and underlying structural or functional cardiac abnormalities. When providing complete cardiopulmonary support (VA-ECMO), the arterial cannula is generally introduced into the ascending aorta, right common carotid artery, or either femoral artery. Other cannulation sites occasionally may be used depending on unique anatomic considerations. The importance of proper cannula selection and quality of the cannulation to successful ECMO support cannot be overemphasized. The right common carotid artery is generally the ideal site for peripheral arterial cannulation in neonates and infants because of the vessel’s diameter and the fact that relatively direct access to the right atrium makes the right internal jugular vein the optimal site of venous cannulation. The optimal cannula size must take into consideration the size of the carotid artery and the projected amount of blood flow through the cannula. Cannulation with an oversized cannula should be avoided to prevent injury to the carotid endothelium or complete and catastrophic vascular disruption. The cervical arterial cannula is inserted into the common carotid under direct vision using a limited neck incision. The cannula is advanced so that the tip lies at the base of the innominate artery. It is important to avoid advancing the cannula too far into the aorta to prevent partial occlusion of the aortic arch, injury to the facing aortic wall, or retrograde orientation of the cannula into the ascending aorta. The latter puts unnecessary mechanical stress of the aortic valve that may lead to injury to the delicate valve leaflets or valvular insufficiency. When cannulating the carotid artery, the surgeons should consider the potential for carotid reconstruction once ECMO support is discontinued. Although prior neck cannulation does not preclude subsequent use of the carotid artery, occlusion of either carotid artery is an obvious contraindication to cervical arterial cannulation. Carotid artery reconstruction at the time of decannulation can be performed if cannulation techniques are employed that are conducive for this. Several reports have demonstrated very good short- and long-term patency following post-ECMO arterial reconstruction.57,58 Carotid reconstruction is not performed by all centers and may not always be possible. There are no data demonstrating an advantage with respect to long-term neurologic function in patients with as opposed to without carotid reconstruction. Long-term neurologic outcome may be more related to the condition of the patient at the time of initiation of ECMO, as well as to other factors related to their underlying condition (such as hypoxemia and adequacy of cerebral blood flow). Despite the lack of conclusive data, carotid reconstruction has advocates and is generally recommended if feasible. Direct cannulation of the ascending aorta is generally performed in patients who require emergent circulatory support in the immediate postoperative period. This approach enables the clinician to evaluate the mediastinum for evidence of tamponade and perform direct cardiac massage when needed. The principles of aortic cannulation used during cardiac surgery are applicable to ECMO patients. The site of cannulation should be above the sinotubular junction to avoid injury or distortion of the aortic valve, which may lead to valvular insufficiency. The cannula is secured with a purse-string suture and flexible tourniquet. It is important to direct the tip of the cannula away from the aortic valve while avoiding injury to the back wall of the aorta. Oversizing the cannula or advancing the cannula too far into the aorta may cause a mechanical obstruction to antegrade flow through the aortic arch and can contribute to difficulties in weaning ECMO support before decannulation. Femoral artery cannulation is useful in larger patients and in patients with compromised carotid blood flow. Either femoral artery may be cannulated under direct vision or using percutaneous Seldinger technique. The cannula is advanced so that the tip lies in the descending aorta. We advocate direct suture repair of the femoral artery at time of decannulation to limit subsequent limb morbidity related to vascular insufficiency. In some patients, it may be safer to sew a side graft onto the femoral artery and place the cannula into this side graft. This may be especially useful in patients with small femoral arteries in whom placement of an adequate size cannula would otherwise be hazardous. For example, a 5-mm side graft is large enough to allow insertion of a 14-mm cannula and an 8-mm side graft would easily accommodate a 22-mm cannula. This technique can be extremely valuable in selected cases and greatly reduces the risk of dissection of the femoral or iliac vessels during cannula insertion.
Venous cannulation is generally performed through the right internal jugular vein, either femoral vein, or the right atrial appendage. However, as with arterial cannulation, certain anatomic considerations may require the clinician to adopt unique venous cannulation strategies, such as is seen in patients who require support following superior cavopulmonary (Glenn) anastomosis.
The technique for VA-ECMO jugular venous cannulation involves placing a standard venous cannula into the internal jugular vein, and advancing the tip to the cavoatrial junction. Although this may be performed using the percutaneous Seldinger technique, we prefer to perform venous cannulation under direct visualization to prevent unnecessary injury to the vascular structures in the neck and chest. We routinely perform jugular vein reconstruction at time of decannulation, though, admittedly, short- and long-term patency rates are poor. Alternatively, femoral venous cannulation may be performed through either femoral vein. We prefer the percutaneous Seldinger technique of cannulation when possible. The femoral venous cannula is advanced until the tip lies within the right atrium. Placement must be confirmed by chest radiography. Cannulation involving both femoral vessels in one leg or either femoral vessel in each leg is possible. Femoral vein reconstruction is generally not necessary after removing a venous cannula. The technique for right atrial cannulation is similar to that used in the operating room for CPB, utilizing a broad-based purse-string situated mostly on the right atrial appendage. A variety of cannulae may be used. Care must be taken to avoid injury to the sinoatrial node or the tricuspid valve during cannulation.
Aortic valve insufficiency and increased systemic arterial pressure may contribute to left ventricular distention, particularly in the setting of poor ventricular function. The subsequent increase in wall tension and myocardial oxygen demand will ultimately impede myocardial recovery. Improper carotid and aortic cannulation can exacerbate this process, by generating a flow jet that is directed toward the aortic valve. When necessary, decompression of the left ventricle may be accomplished in several ways. In the setting of a closed chest, left ventricular decompression may be accomplished by creating, or augmenting, an interatrial communication (Fig. 87-2). When present, a patent foramen ovale can be enlarged at the bedside by balloon septostomy under echocardiographic guidance. Alternatively, blade or balloon septostomy may be performed in the catheterization laboratory, even in the absence of a preexisting interatrial communication. Recently, Cheung et al.59 have described an alternative method of left heart decompression, utilizing a percutaneous technique for inserting a vent into the left ventricle that is connected to the venous arm of the ECMO circuit. More options are available for decompressing the left heart in cases of an open chest. Since many of these patients are placed on ECMO in the operating room, a cannula can be placed directly into the left atrium and connected to the venous return of the ECMO circuit. This cannula can be placed through the left atrial appendage or in the space between the two right-sided pulmonary veins. Either site is easily accessible and readily visualized in an ICU setting if reexploration for any reason becomes necessary. Alternatively, a surgical atrial level communication by atrial septectomy can be performed, precluding the need for additional cannulae. Irrespective of the technique used, we believe it is important that left ventricular decompression is achieved soon after initiating ECMO support in patients with severely depressed left ventricular function. In addition to decreasing the likelihood of recovery of ventricular function, left ventricular distension may lead to increased left atrial pressure, causing fulminant pulmonary edema to rapidly ensue. In addition, the relative amount of coronary blood flow derived from the ECMO circuit (aortic or carotid cannula) or the lungs (through antegrade flow through the aortic valve) is dependent upon the amount of cardiac support provided by the ECMO circuit. At lower levels of support, the blood delivered to the coronary arteries is predominately derived from the left ventricle, whereas all coronary blood flow is derived from the ECMO circuit during periods of total cardiopulmonary support and the absence of left ventricular ejection.60 Even at high ECMO flow rates, the coronaries preferentially receive blood supplied by the left ventricle blood. Effective left-sided decompression ensures that adequately oxygenated blood derived from the ECMO circuit will preferentially perfuse the coronaries in the setting of severely depressed lung function. This is an important consideration when weaning ECMO support. Moderate levels of mechanical ventilation must be continued during partial ECMO support and lung function must be adequate to wean ECMO support. Left ventricle decompression can be a source of air being introduced into the ECMO circuit and ECMO technicians need to be aware of this possibility. The decision to utilize left ventricular decompression is one that is dependent on the circumstances of the underlying pathology as well as the experience of the center. Excellent results can be achieved in centers where left ventricular decompression is used sparingly, if at all. In fact, left ventricle decompression in the setting of severe aortic insufficiency may simply set up a “recirculation” circuit and in these cases, ECMO may be contraindicated in lieu of aortic valve replacement or repair.
Unlike the neonate with isolated respiratory failure, in whom ECMO flows and sweep gases are adjusted to maintain adequate oxygenation and ventilation, the infant or child on cardiac ECMO has cardiac insufficiency, often coupled with respiratory insufficiency. The degree to which gas exchange is dependent on ECMO will obviously affect the management strategy. For the patient with isolated cardiac failure, ECMO flows are adjusted to ensure adequate end-organ blood flow. Clinical parameters, such as urine output and toe temperature, should be regularly scrutinized and ECMO flows adjusted to maintain adequate perfusion. Laboratory values, including arterial lactate and mixed venous oxygen saturations, are also important and should be followed serially. In general, flows of 100 to 150 mL/kg/min should be adequate to maintain homeostasis. Some circumstances, as in the case of an infant in whom the pulmonary and systemic circulations are in parallel (single ventricle with aortopulmonary shunt), may require considerably higher flows. It has been and continues to be our practice to keep aortopulmonary shunts open and utilize higher flows. Because of the non-pulsatile nature of generally available circuits, arterial waveform tracings may provide an indication of the degree of native cardiac contribution to systemic output. Lack of discernible pulse pressure indicates that the ECMO circuit is responsible for all the effective systemic blood flow. This circumstance can occur when the heart is completely bypassed, when there is “cardiac stun,” or in the setting of cardiac failure. It is important to remember that coronary perfusion results from antegrade ejection of blood out the aortic valve, and it is only in the setting of nearly complete lack of cardiac output that coronary blood flow becomes dependent on the oxygen-rich blood returning via the arterial ECMO cannula. Bearing that in mind, it is important to ensure that whatever blood is being ejected out the left ventricle is well saturated, so that the heart does not suffer ongoing ischemia. Ventilator Fio2 should thus be maintained around 40 percent.
Serial echocardiograms can be helpful in gauging improvement in cardiac function, particularly in the setting of right ventricular failure where ventricular geometry and pressures can be assessed. Levels of brain natriuretic peptide (BNP) and cardiac troponin I can be followed serially as well. It is often necessary to reduce ECMO flows to allow cardiac filling in order to make a determination. During periods of flow reduction, systemic blood pressure and perfusion are monitored using the clinical parameters described above. Increases in arterial pulse pressure and perfusion indicate improving cardiac function and should encourage further weaning of ECMO flows. When flows have been sufficiently reduced, echocardiography is favorable, and perfusion is maintained on reasonable levels of inotropes, a trial off ECMO is warranted.
For the patient with combined cardiorespiratory failure, estimation of support needs and weaning preparedness are more complicated. In addition to ECMO flow rate, the sweep gas Fio2 and flow rate are important determinants of respiratory gas exchange. In many instances, restoration of adequate circulation with initiation of ECMO leads to rapid improvement in respiratory mechanics and gas exchange as interstitial and alveolar lung water is cleared. Likewise, restoration of normal arterial oxygen tensions and pH can precipitate significant improvement in cardiac function. In addition to the clinical and laboratory parameters previously discussed, regular monitoring of arterial blood gases is vital. Weaning involves gradual decreases in Fio2, sweep flow rate, and ECMO flow rate coupled with corresponding increases in mechanical ventilatory support. Once the patient can maintain physiologic values on reasonable ventilator settings and inotropes, a trial off ECMO is justified.
One consideration for cardiac ECMO is how to handle systemic-to-pulmonary artery shunts, either in single-ventricle patients or in those with inadequate pulmonary blood flow. Our practice is to leave all shunts open, especially considering that the vast majority of our patients are peripherally cannulated through a cervical approach. Currently, we would support a patient with a modified Blalock-Taussig shunt on ECMO would be hypoxemia or evidence of a hyperactive pulmonary vascular bed. In both cases, we would employ venovenous ECMO (VV-ECMO) support with a single cannula placed in the internal jugular vein. In this case, the shunt is not affected by the presence of the ECMO cannula. In the case of a palliated HLHS patient, we again would favor VV-ECMO for low saturations. If a patient were in a state of low cardiac output after Norwood repair and required mechanical cardiac assist, we would use VA-ECMO via neck cannulation. However, we prefer the “Sano shunt” (a valveless conduit from the right ventricle to the pulmonary artery), which would not be affected by an arterial neck cannulation.
Indications for VV-ECMO in the cardiac population may seem limited. In fact, as experience grows, more clinical applications become apparent. VV-ECMO has several potential advantages over VA-ECMO. First, in many instances only a single venous cannula is required and, obviously, no large-caliber arterial vessels are disrupted and potentially sacrificed. Second, physiologic pulsatile flow is maintained. Third, the pulmonary vascular bed is perfused with oxygen-rich blood. And last, the myocardium is perfused with oxygen-rich blood. The last two features deserve particular consideration as they apply to the cardiac population.
Pulmonary hypertension is a common comorbidity in the pediatric cardiac population.61 Elevated pulmonary vascular resistance (PVR) and right ventricular dysfunction often lead to significant morbidity and even mortality in the perioperative period. In addition, infants with unrepaired cyanotic heart disease are at particular risk when they acquire common viral respiratory illnesses.61–64 We have found VV-ECMO to be the ideal mechanical support mode in these settings to improve oxygenation, correct of respiratory acidosis, and reduce PVR. Clinically, the result is increased pulmonary blood flow, decreased right ventricular strain, better left-sided filling, enhanced myocardial oxygenation, and improved cardiac output. We consistently note rapid decreases in inotropic requirements following the institution of VV-ECMO.
Imamura and associates at Arkansas Children’s Hospital report a similar experience in 10 patients placed on VV-ECMO for support in the setting of acute viral pneumonia and another 8 patients with inadequate pulmonary blood flow.61 In each of these two groups, VV-ECMO served as a bridge to recovery or surgical intervention. Survival to discharge was 95 percent.
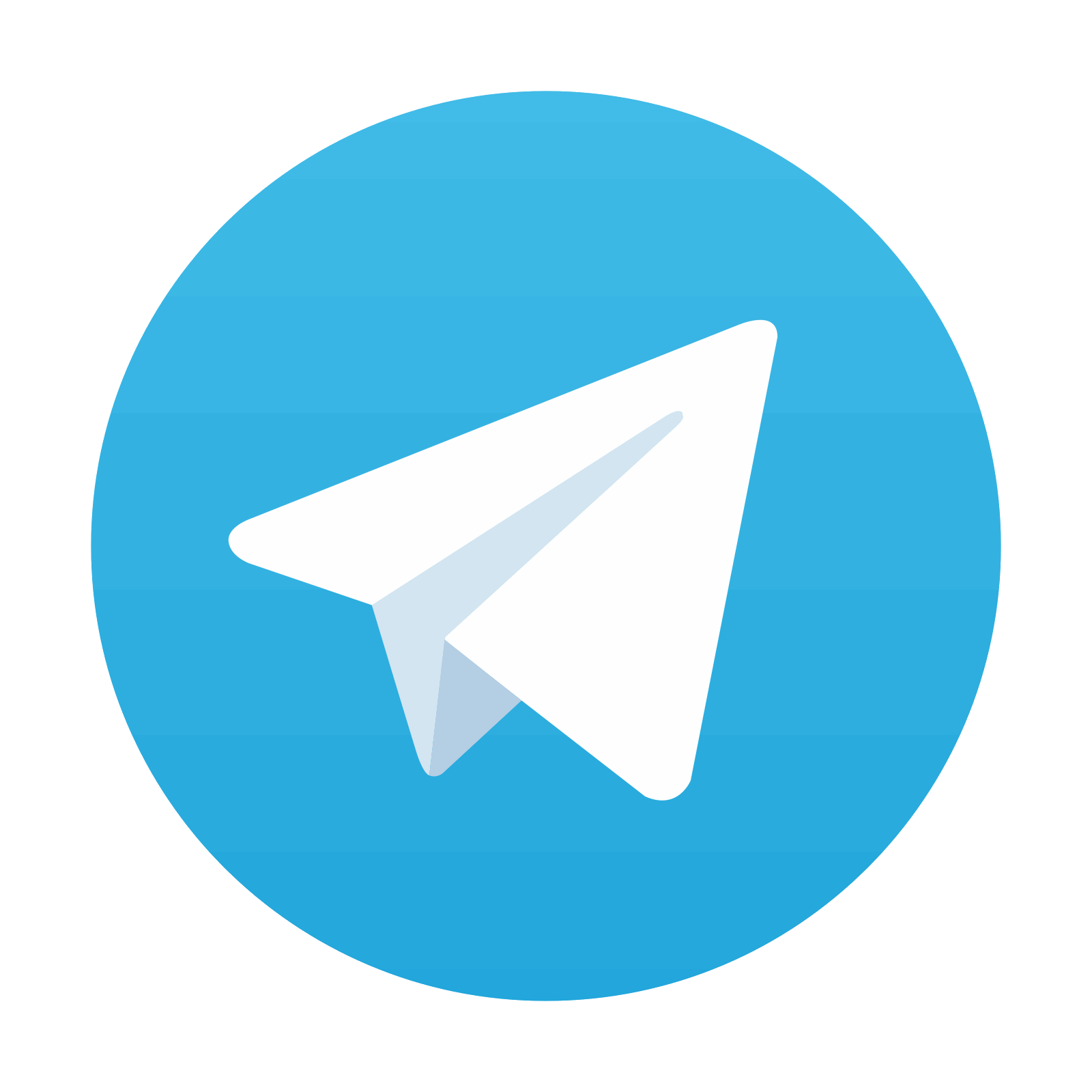
Stay updated, free articles. Join our Telegram channel

Full access? Get Clinical Tree
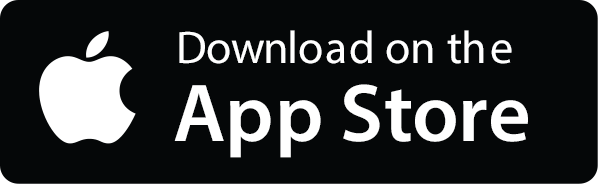
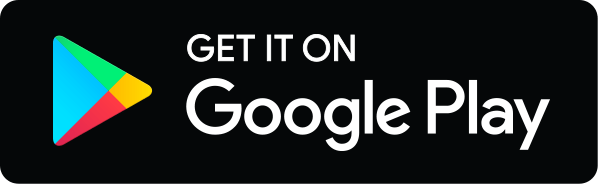