Outline
Introduction 401
Alternative Methods 403
Adult Stem Cells 403
Multipotent Stem Cells from Extraembryonic Tissues 404
Reprogramming of Somatic Cells: Induced Pluripotent Stem Cells 405
Altered Nuclear Transfer 405
Single Blastomere Biopsy 405
Organismically Dead Embryos 405
The goal of regenerative medicine is to rebuild or repair damaged or diseased structures and restore their function. Human embryonic stem cells (hESCs) hold great promise for use as a tool in regenerative medicine. This chapter discusses ethical issues surrounding hESC research and gives an overview of alternative sources of pluripotent stem cells and alternative approaches for hESC derivation.
Introduction
Regenerative medicine entails a broad range of methodologies that utilize cells or tissues as therapeutic tools to rebuild or repair damaged or diseased structures and restore their function. The source of therapeutic cells can be autologous, allogenic or xenogenic. If allogenic, then these cells can be harvested without harm or risk of harm or cooperation with harm to another human being or harvested with corresponding harm. Ethical issues abound in experimental medicine but those that arise uniquely in regenerative medicine reflect this latter circumstance, wherein one human life is used as an object to satisfy the needs or desires of another.
The developmental potential of embryonic cells and fetal cells and tissues has attracted the compelling interest of investigators ( Fig. 25.1 ). The interest of the political establishment and the media are similarly engaged in part because the ethics of regenerative medicine are so frequently intertwined with the ethics of abortion. The ethics of human embryonic stem cells (hESCs) and of abortion both hinge on the respect, or the absence of respect, accorded to the human organism at a prenatal stage of development. In hESC research, which historically required destruction of a live embryo to make a new cell line , the biological fact that a new human life appears at fertilization is ever at risk of subordination to a utilitarian calculus. In this calculus, utility to another determines which life will or will not be accorded respect and rights consistent with human dignity and, if so, then to what extent. The ferocity of the abortion debate, together with the contradictions anticipated if the respect mandated to a human embryo by an investigator were to exceed that accorded to a human fetus by its erstwhile mother, helps to drive the stem cell debate.

Another driver of the debate is the issue of scientific freedom of inquiry. Researchers militate for unrestricted access to tools for basic investigation and therapeutic intervention and the perspectives of this lobby are largely sacrosanct. However, the public frequently funds or is asked to fund such research and the broader society is divided on the question of medical progress if achieved at the cost of destroying healthy human embryos. Even more germane than its role as the source of research funding, the public is the presumptive recipient of the therapies that are the rationale for much of the publicly funded research. Some have suggested that the monolithic prerogatives of investigators must yield to those of the divided, and consequently more circumspect, body politic.
The opposition of many patients to aspects of regenerative medicine as currently practiced is not only to a therapy that requires the destruction of an embryo or fetus for its immediate implementation, but also to a therapy that entailed such destruction, perhaps years before, in the creation of the therapeutic cell lines . The issue of “cooperation” with morally suspect practices complicates the use of organs from condemned prisoners, tissues from electively aborted fetuses and human embryonic stem cell lines derived through the destruction of healthy human embryos ( Table 25.1 ) .
Common ground | Requiring cooperation | Generally objectionable | |
---|---|---|---|
Embryonic | Dead embryo created for reproductive purposes that died despite best efforts | Surplus embryo from in vitro fertilization for reproductive purposes | Embryo created for destructive harvesting |
Fetal | Spontaneous abortion | Therapeutic abortion | Abortion after conception for the purpose of abortion and harvesting |
Adult | Deceased adult | Prisoner after legal execution | Execution of political prisoner for the purpose of harvesting |
The division of opinion on the destructive use of prenatal human beings cannot be solved by splitting the difference. The contest, framed in its most positive terms, pits the imperative to respect human dignity and treat human beings as subjects and never as objects against the duty to devise and implement methods to relieve human suffering. This division is not economic and thus cannot be solved by splitting the difference. The difficulties with persuasion and the unseemliness of coercion have argued in favor of a search for a common ground. Alternative approaches that spare human life at the earliest stage of development and yet achieve the objectives of scientific inquiry have sparked considerable interest ( Fig. 25.2 ). These alternative approaches were reviewed in a 2005 White Paper from the US President’s Council on Bioethics. Considerable progress has been made in this area and in the following sections the current status of the alternative embryo-sparing approaches is reviewed.

Alternative Methods
Adult Stem Cells
Adult stem cells (ASCs) represent ethically acceptable source of stem cells and are eligible for federal funding in the USA and elsewhere ( Fig. 25.2 ). ASCs reside within different tissues as tissue-specific progenitors that are employed during tissue self-renewal and/or differentiation. When transplanted into the host, ASCs exhibit lower tumorigenic potential than hESCs. ASCs play an important role in the lifelong maintenance of tissue homeostasis . Any homeostatic disturbance can disrupt a dynamic balance between self-renewal and differentiation which can lead to excessive differentiation, resulting in the exhaustion of the stem cell pool or overproduction resulting in tumorigenesis .
Bone marrow represents a typical adult stem cell source, containing different cell populations that have the potential to migrate and transdifferentiate into cells of diverse phenotypes . The plasticity of bone marrow-derived stem cells has been broadly studied. Two major subsets of bone marrow cells include hematopoietic stem cells (HSCs) and mesenchymal stem cells (MSCs). HSCs are commonly identified by the expression of CD34 + cell surface antigen; HSCs have been studied extensively and used clinically for bone marrow transplantation in a variety of hematological disorders .
MSCs are a population of cells present in adult tissues including the bone marrow and adipose tissues . They have been shown to differentiate into multiple tissues including neuronal, adipose, muscle, liver, lungs, spleen and gut. MSCs derived from adipose tissue are particularly attractive because they are easily accessed and not in short supply.
In summary, ASCs represent an ethically acceptable source of stem cells, albeit currently of limited therapeutic use except for HSCs. The major reasons are the challenges involved in isolating scarce ASCs, their restricted differentiation potential, and difficult and often unsuccessful ex vivo expansion . If these drawbacks were successfully resolved, ASCs could become an important source of tissue-specific stem cells for regenerative therapies.
Multipotent Stem Cells from Extraembryonic Tissues
Umbilical Cord as Stem Cell Source
The umbilical cord is another non-controversial source of stem cells ( Fig. 25.1 ). Stem cells are isolated from two compartments: umbilical cord blood (UCB) and umbilical cord matrix, i.e. Wharton’s jelly . UCB contains stem cells which have been successfully used for transplants in the treatment of leukemia . They are useful clinically, owing to their naïve immune status . UCB stem cells give rise to cardiac, neural, hepatic and dermal tissues in vitro, in addition to hematopoietic lineage . The isolation of UCB stem cells is non-invasive; they are easily collected and banked. However, the main drawback is the limited number of cells available from a single unit, estimated to represent only 5% of the optimal dose required for adult hematopoietic transplantation .
Besides UCB stem cells, mesenchymal stromal cells can be isolated from the Wharton’s jelly of the umbilical cord . Mesenchymal stromal cells can be expanded more efficiently ex vivo than UCB stem cells and were shown to differentiate into bone, cartilage, muscle, fat and neural tissue under suitable conditions . Thus, they are intensively studied for clinical and tissue engineering applications.
Currently, numerous facilities exist that offer long-term storage of UCB stem cells in the hope of future applications of these cells for broad therapeutic purposes . However, at this time the only realistic therapeutic application is for the treatment of leukemias and lymphomas. Physicians should have this information in mind when advising parents wishing to store the cord blood or the umbilical cord of their yet to be born child.
Placenta, Amnion and Amniotic Fluid as Stem Cell Source
The placenta and amnion are additional extraembryonic sources of multipotent stem cells. Fetal placenta has four distinct regions: amniotic epithelial, amniotic mesenchymal, chorionic mesenchymal and chorionic trophoblastic . From these regions, the following cell populations are isolated: human amniotic epithelial cells (hAECs), human amniotic mesenchymal stromal cells (hAMSCs), human chorionic mesenchymal stromal cells (hCMSCs) and human chorionic trophoblastic cells (hCTCs) . Placenta is readily available upon birth and represents an ethically non-controversial source of stem cells ( Fig. 25.1 ). However, various placenta-derived cells display variable differentiation potential and self-renewal has not been clearly demonstrated . Therefore, further investigations are required to determine the full potential of these various placental stem cells in order to predict their eventual applicability in therapy.
Amniotic fluid-derived stem cells are isolated from the amniotic fluid, which is routinely isolated during amniocentesis for prenatal diagnosis ( Fig. 25.1 ) . The origin of amniotic fluid-derived stem cells is still under investigation, although current theories suggest that their source is either urinary and pulmonary secretions from the fetus or potentially ultrafiltrate from the plasma of the mother entering through the placenta. Thus, it is not certain whether these cells are an intermediate between adult and embryonic stem cells. Nevertheless, these cells express some markers of pluripotency (such as POU5F1 ), display high proliferative capacity, differentiate into multiple lineages and do not form tumors in vivo . Amniotic fluid-derived stem cells are phenotypically most similar to hAMSCs. The major drawback is their isolation during amniocentesis with the well-known risk of miscarriage due to the procedure. While this risk is accepted when amniocentesis is indicated for the purpose of prenatal genetic diagnosis it would not be acceptable exclusively for the purpose of stem cell isolation .
While all of these extraembryonic tissues represent non-controversial sources of stem cells that could be valuable for specific therapeutic purposes, it is beyond reasonable speculation whether they could replace or substitute for hESCs and their derivatives in vivo given that the full potential of therapeutic application of hESCs is unknown at this time .
Reprogramming of Somatic Cells: Induced Pluripotent Stem Cells
Somatic cells can be reprogrammed into induced pluripotent stem (iPS) cells ( Fig. 25.2 ) by the addition of several genes, as initially reported by Yamanaka’s and Thomson’s laboratories . Yamanaka and colleagues generated human iPS cells through retrovirus-mediated transfection of the genes POU5F1 , SOX2 , KLF4 and c-MYC , while Thomson and colleagues generated iPS cells without the oncogene c-MYC , inducing the pluripotent state by retroviral transfection of POU5F1 , SOX2 and NANOG . It was demonstrated that iPS cells are similar to hESCs by morphology, proliferative capacity and pluripotency. Since these initial reports on iPS cell derivation, a variety of different methods has been reported for reprogramming (see Chapter 13 ) .
Reprogramming is an exciting approach as it does not require the use of human oocytes and/or embryos. Induced pluripotent stem cells opened new possibilities particularly for interrogating human disease. Derivation of patient-specific iPS cells will recapitulate disease pathology and this type of research will give important insight into the pathogenesis of many degenerative diseases. Also, iPS cells are an extensive source of histocompatible pluripotent stem cells, which makes them particularly appealing for clinical use. However, there are several important concerns to be resolved: the low efficiency of reprogramming, tumor formation, and developmental equivalence between iPS cells and hESCs. Two recent reports show that reprogrammed cells fail to differentiate or do not differentiate as efficiently as hESCs . These findings clearly demonstrate that iPS cells are distinct population of pluripotent stem cells and indicate the imperfections in the reprogramming process. Further research is needed to diagnose and overcome these deficiencies of iPS cells prior to their use in regenerative medicine.
Altered Nuclear Transfer
Altered nuclear transfer (ANT) is a theoretical method for derivation of pluripotent stem cells that would avoid embryo destruction . It is based on somatic cell nuclear transfer (SCNT), with a modified nucleus that would lead to an embryo-like structure incapable of implantation ( Fig. 25.2 ). It has been shown in mice that embryos with a mutation in the Cdx2 gene die at the blastocyst stage since trophectoderm fails to form, while the ability to derive hESCs from the inner cell mass was unchanged . It remains unknown whether CDX2 is the optimal target in human development and whether such embryos could be used to derive stem cells . It is also unclear whether these implantation-disabled structures could be considered as biological artifacts and not as defective embryos . Finally, the requirement for a large supply of human oocytes, if ANT should prove successful, implicates further ethical challenges .
Single Blastomere Biopsy
Lanza and co-workers developed single blastomere biopsy (SBB) for the purpose of deriving hESCs in a manner that would avoid embryo destruction . This approach uses a technique that was originally developed for preimplantation genetic diagnosis (PGD) , the difference being the derivation of hESCs from a single removed blastomere rather than genetic testing . A biopsied embryo can continue developing and reach the blastocyst stage and beyond, which was demonstrated with more than a decade of experience with PGD . This method has been used successfully to derive hESC lines . Although this approach bypasses the ethical issue of embryo destruction it brings the risk associated with embryo biopsy which is accepted as the part of PGD procedure, but it would be considered unjustified in the absence of a clinical indication . In addition, US regulations forbid research on an embryo that imposes greater than minimal risk, unless the research is for the direct benefit of the fetus . To date, none of the hESC lines derived by SBB has been approved for National Institutes of Health (NIH) funding .
Organismically Dead Embryos
Embryos engendered by in vitro fertilization (IVF) procedures frequently fail to develop properly and are classified as non-viable . Many non-viable embryos have undergone an irreversible arrest of normal development. Aneuploidy is widespread but mosaicism is common with normal cells distributed among the abnormal. Even a few- celled embryo is an organism with cell-cell communication essential for normal growth and development. Normal cells deprived of integration fail to develop and die. Irreversibly arrested, non-viable embryos satisfy the universal concept of death for multicellular life: an irreversible loss of integrated organismic function. We proposed that hESCs could be derived from cells harvested from human embryos that have died, despite best efforts, during the course of IVF for reproductive purposes . This approach, wherein live cells are harvested from dead embryos is analagous to the harvesting of essential organs from deceased donors and the established ethics of essential organ donation could be applied to the derivation of new hESC lines . The use of brain death as a criterion for diagnosing the death of a developed human informs the use of irreversible arrest of normal development in diagnosing the death of a developing human. The utility of a crisp diagnosis of death in the developed human avoids the inappropriate application of scarce medical resources and is the foundation of essential organ transplantation. Similar benefits would accrue to the diagnosis of death in the developing human.
The natural history studies that provided the foundation for diagnostic criteria for brain death inspired similar studies of non-viable embryos. Observation of non-viable embryos that arrest and then decompose could provide a stage of development and a duration of arrest that define a time beyond which, having failed to develop, no development is possible. In a retrospective study of non-viable embryos, we determined that many of the non-viable embryos rejected for clinical use were organismically dead: the failure of normal cell division for 48 h was irreversible and, despite the possible presence of individual living cells, indicated an irreversible loss of integrated organismic function . A follow-up prospective study examined the progression of non-viable embryos in extended culture . The analysis showed that developmental arrest observed in some human embryos by embryonic day 6 (ED6) following IVF cannot be reversed by extended culture in conditions suitable for preimplantation embryos, as no morphological changes indicative of normal developmental progression were seen and no unequivocal instances of further cell divisions were observed . These observations are in line with standard IVF practice; such embryos are not transferred or cryopreserved because they are known not to produce live offspring . Although morphological categorization of embryos is of limited value in predicting cell number, the higher cell number associated with cavitation may predict greater potential for the success of hESC derivation . Also, the majority of irreversibly arrested embryos contain a high proportion of vital cells regardless of the stage of arrest, indicating that harvesting cells and deriving hESCs from such non-viable embryos should be feasible .
To date, 14 hESC lines have been successfully derived from non-viable embryos that were irreversibly arrested, according to these criteria, thus demonstrating the feasibility of this alternative approach . The first cell line (hES-NCL9) was derived from 132 arrested embryos . In a different study, 11 hESC lines were derived from 413 poor-quality embryos rejected for clinical use . Recently, our group has derived two hESC lines: CU1 and CU2 from 159 ED6 irreversibly arrested, non-viable human embryos . Pluripotency and differentiation potential were demonstrated in vitro and/or in vivo for all 14 hESC lines derived . While it is common for arrested embryos to be aneuploid , analysis revealed normal or apparently normal karyotype in all 14 hESC lines derived to date .
Derivation of hESC lines from organismically dead embryos has the likelihood of being accepted by the strongest opponents of hESC research . This approach is unique because it defines a common ground in the stem cell debate. Eleven hESC lines derived by Daley and colleagues have been included in the NIH stem cell registry and are available for research with NIH funding . Despite the low efficiency of isolation of hESCs from organismically dead embryos, large-scale derivation is feasible because in the US alone nearly half a million such embryos are generated yearly as a by-product of IVF for reproductive purposes . Available data suggest that hESCs derived from organismically dead embryos are of equal quality to lines derived by the classical, inner cell mass (ICM) derivation approach, but further characterization of these lines is needed .
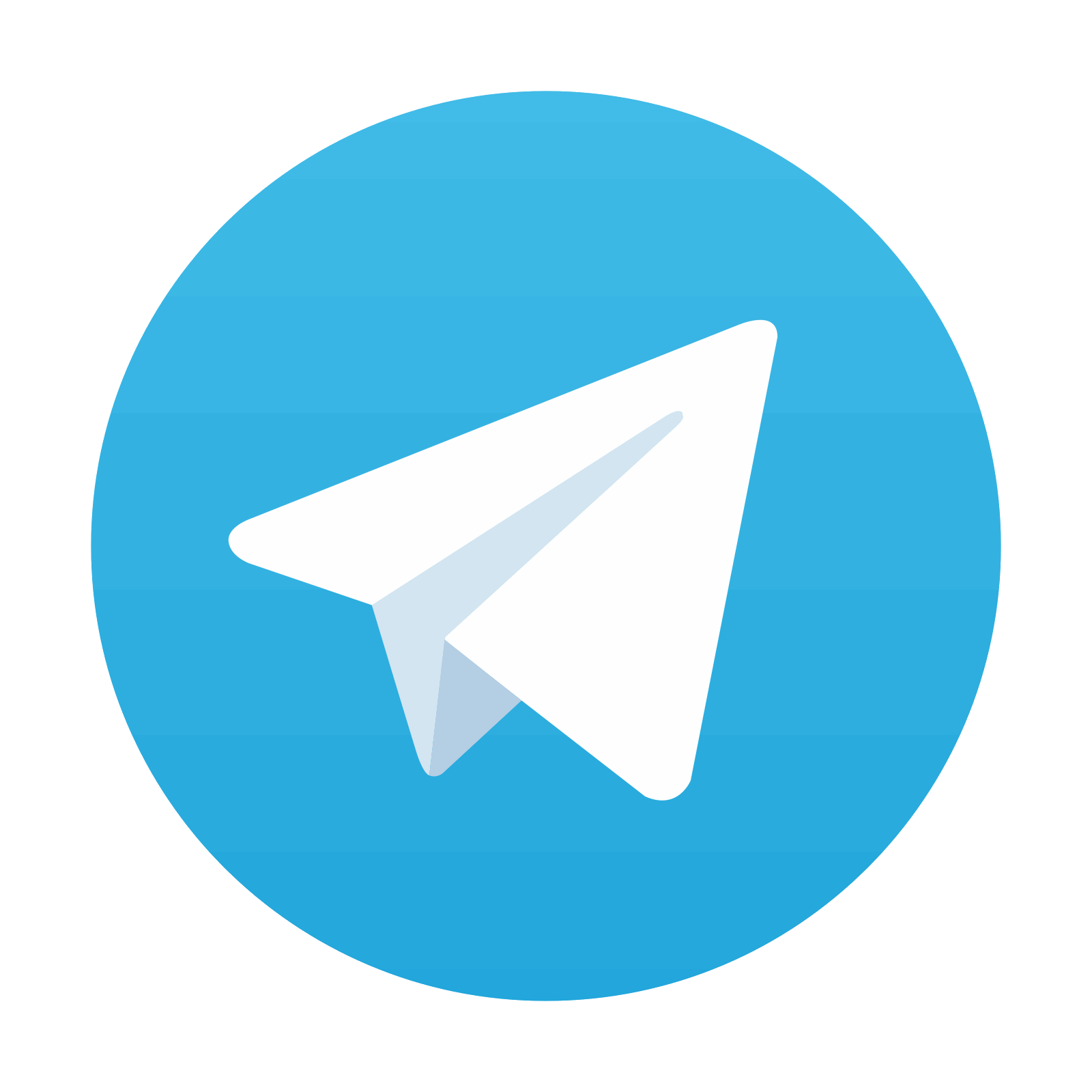
Stay updated, free articles. Join our Telegram channel

Full access? Get Clinical Tree
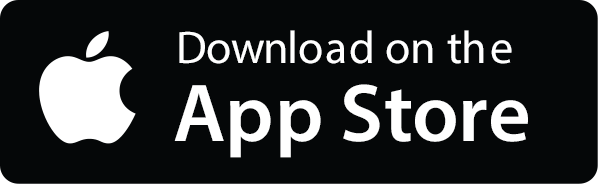
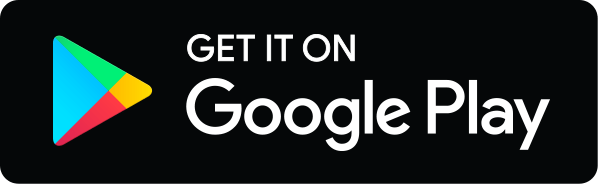