Fig. 6.1
Flow diagram for the management of preterm newborns with feeding intolerance. Note: *Erythematic abdominal wall, absence of bowel sounds, blood in the stools or in aspirates, bile in the aspirate, or radiological or ultrasonographic markers of NEC Bell’s stage > I
Nutrition During the Intermediate and Stable Growing Period
During this period, parenteral fluid is progressively reduced and, thus, interrupted as soon as 120 ml/kg/day (100 kcal/kg/day) by enteral route is well tolerated, Subsequently, enteral feeding can be slightly increased, up to 140–180 ml/kg/day (115–135 kcal/kg/day), according to nutritional requirements and type of formula used. During any phase of nutritiona approach, protein to energy ratio (PER) should be conserved and parenteral nutrition adjusted in relation to nutrients given by enteral route. Human milk is the preferred feed for preterm infants during the intermediate and stable growing period. Weaning period from parenteral nutrition may be at high risk of relative malnutrition , contributing to cumulative nutritional deficit and postnatal growth restriction [9]. It is commonly accepted that human milk needs to be fortified to meet the nutrient needs of the preterm infant. Fortified human milk feeding regime significantly improves growth. Fortification provides adequate intakes when the composition of human milk plus fortifier reaches 2.5 g protein/100 ml with a PER of 3.2 g/100 kcal [1]. Measuring the composition of human milk feeds prior to fortification and targeting the fortification according to individual needs of each infant would be ideal [9]. When human milk is unavailable, preterm formula is used for feeding very preterm infants. All preterm formulas are protein and energy enriched (~ 80 kcal/dl, PER 3.0–3.6 g/100 kcal), have low osmolarity (325 mmol/kg H2O), and typically contain added whey protein, glucose polymers, medium-chain triglycerides (MCT), a source of long-chain polyunsaturated fatty acids (LCPUFAs) , calcium, phosphorus, electrolytes , folate, and fat-soluble vitamins . Upper limits of recommended nutritional intake are met when preterm formula is fed at rates approaching 160 ml/kg/day [5].
Feeding Mode
Bolus or (Semi-) Continuous Feeding
Bolus feeding results in a significant increase in blood flow to the portal-drained viscera (i.e., stomach, spleen, intestine, and pancreas). There is a surge in GI hormones, which is higher than when neonates are fed continuously. It has been also suggested that in preterm infants fed with fortified human milk, continuous feeding leads to important nutrients’ loss on nasogastric tube walls (i.e. fat and calcium). However, it has been demonstrated better tolerance with continuous milk feeding in preterm neonates [10, 11]. Bolus feeding may result in apnea, deteriorating respiratory mechanics with decreased tidal volumes, and transient presence of blocking materials of the nostrils, such as nasogastric tubes, or noninvasive respiratory support devices. However, a large randomized controlled trial showed that even extremely-low-birth-weight (ELBW, < 1000 g) infants may tolerate bolus feeding well [10, 11]. In conclusion, there is still not enough evidence to recommend either bolus or (semi-) continuous feeding as the preferred method, but the change from one to another can be justified when feeding intolerance persists in smaller neonates.
Oral Feeding
Oral feeding is possible when an infant is capable of an adequate suck–swallow reflex. In the term infant, the full, complex, integrated mechanism of swallowing, with the movement of the bolus of milk into the stomach, protection of the airway, inhibition of respiration, and appropriate relaxation of the esophageal sphincter and gastric fundus is achieved within 2 days of life. The swallow function is present from 16 weeks of gestational age, and GI motor activity, in small bursts, from 24 weeks onwards [2]. Organized motility is present from around 30 weeks of gestation and nutritive and swallowing function from 32 weeks. Enteral nutrition promotes postnatal maturation of intestinal motor activity. It follows that oral feeding is only possible from 32 weeks onwards, but Nonnutritive sucking can start earlier. The very preterm infant (< 32 weeks) may be put on the breast but the likelihood that the infant will ingest a significant volume of milk is very small. However, it may offer the mother a considerable psychological benefit and should therefore be strongly encouraged.
Intragastric Feeding
As oral feeding is not possible in VLBW infants, most frequently gastric tubes are used [11]. Either nasogastric or orogastric tubes can be installed, both checked in the right position (i.e., fundus) by measuring external distance (i.e., distance from nose or mouth to ear plus distance from umbilicus and xiphoid process), and checking the aspirate for acidity with litmus paper. Correct position should be confirmed at the first radiographic examination performed for such reasons and reported in the clinical chart. The nasogastric tube has the advantage of easier fixation but has the disadvantage of (partially) blocking one nostril. With the use of some respiratory devices, only the orogastric tube is possible. Finally, tubes and syringes may lead to loss of nutrients such as lipids and calcium that may not reach the infant [11].
Transpyloric Feeding
Start, Check the Tolerance and Increments of Enteral Feeding
Enteral nutrition is started at 10–30 ml/kg of body weight per day. Feeds could be given by gavage or with a continuous infusion pump and increased by 20–30 ml/kg/day according to feeding tolerance . [12]. Feeding intolerance may sometimes be obvious when severe GI manifestations are identified (emesis, vomiting, severe abdominal distension, ileus with visible intestinal loops, blood in the stools) or when GI symptoms are associated with systemic disorders like apnea, bradycardia, poor perfusion, and/or hemodynamic instabilities. However, intermediate situations are frequent in VLBW infants. In particular, abdominal distension is frequently observed during noninvasive ventilation and antegrade peristalsis with bilious gastric aspirates is also commonly described during the first week of life in VLBW infants.
The aspiration of gastric residuals is common practice while tube-feeding infants and aspirate usually need to be reinfused to reduce the loss of enzymes and electrolytes . Most clinical studies demonstrated little if any predictive value of gastric residuals > 3–4 ml/kg or > 30–50 % of the previous feed and of bilious-stained feed [12]. Therefore, in the absence of significant clinical signs or symptoms, the presence of gastric residuals < 4 ml/kg or < 50 % of 3-h previous feed is not a valid reason to interrupt or reduce enteral feeding [12]. Maintaining sufficient enteral feeding can in fact improve GI maturation and feeding tolerance. If enteral feeding has been interrupted but clinical evolution rules out a significant disease, enteral feeding need to be restarted as soon as possible to promote intestinal maturation.
In the absence of signs of feeding intolerance, the volume of enteral nutrition could be daily increased. The ideal rate of progression of milk volume given to VLBW infants is controversial. Cochrane Review has concluded that a rapid rate of advancing feeds was not associated with a higher risk of NEC [13]. Although additional studies are needed, a small progressive increment of 20 ml/kg/day after a few days of stabilization can be suggested. (Fig. 6.1). In the presence of erythematic abdominal wall, absence of bowel sounds, blood in the stools or in aspirates, enteral nutrition should be discontinued and serial abdominal ultrasounds and radiological examinations should be started in order to early identify NEC [8, 12, 14].
Nutrient Needs in Enterally fed Neonates
Protein
Protein Requirements
The optimal quantity of dietary protein for preterm infant formulas is still a matter of debate. Dietary protein needs for preterm infants were calculated through two different methods [15]. The first, the empirical approach, measures biochemical or physiological responses to graded intakes. The second method, the factorial approach, considers the requirements as the sum of the essential losses (e.g., urine, feces, skin), plus the amount incorporated into newly formed tissues accounting theoretical fetal accretion at the same PMA. The empirical approach evaluates physiological and biochemical variables to determine minimum and maximum protein needs in the growing preterm infant.
In the factorial approach, compositional analysis of fetal tissues has been a valuable source of data for our understanding of the nutrient needs of the fetus and, by extension, those of the growing preterm infant. Fetal accretion rates have been obtained from compositional analyses of aborted fetuses or stillborn infants. From these data, the protein increment for growth has been estimated as approximately 2.5 g/kg/day [5]. Protein requirement is thus calculated by adding to this value the obligatory losses, and the amount needed for the additional catch-up growth. In a large population of preterm infants receiving a controlled energy intake, the minimal protein supply necessary to obtain a zero nitrogen balance (to cover all nitrogen losses) was calculated to be 2 g/kg/day. A similar value was estimated to cover the need for catch-up growth. Thus, the protein requirements of premature infants are between 3.5 and 4.0 g/kg/day (Fig. 6.2) [5].
Protein intake must be sufficient to achieve normal growth without negative effects such as acidosis, uremia, and elevated levels of circulating amino acids [1, 15, 17]. A recent Cochrane analysis aimed to determine whether higher (≥ 3.0 g/kg/day) versus lower (< 3.0 g/kg/day) protein intake during the initial hospital stay of formula-fed preterm infants or LBW infants (< 2500 g) results in improved growth and neurodevelopmental outcomes without evidence of short- and long-term morbidity [17]. Five studies comparing low versus high protein intake were included. Improved weight gain and higher nitrogen accretion were demonstrated in infants receiving formula with higher protein content while other nutrients were kept constant. No significant differences were seen in rates of NEC, sepsis, or diarrhea. One study compared high (3 g/kg/day) versus very high (> 4 g/kg/day) protein intake during and after an initial hospital stay [18]. Very high protein intake improved gain in length at term, but differences did not remain significant at 12 weeks corrected age. Three of the 24 infants receiving very high protein intake developed uremia, defined as serum urea nitrogen level greater than 6 mM/ml. Formula with higher protein content improves all growth parameters and neurodevelopment [19, 20]. However, available evidence is not adequate to permit specific recommendations regarding the provision of very high protein intake (≥ 4.5 g/kg/day) from formula during the initial hospital stay or after discharge.
Enteral Nutrition Protein Composition
Nitrogen absorption rate (absorbed/intake) differs significantly according to the feeding regimen (Fig. 6.3) [21] . It is higher with powder whey-predominant protein preterm formulas than with human milk supplemented with fortifiers powder protein hydrolyzed formulas or ready-to-use liquid whey-predominant preterm formulas. These differences result from the nature of the various types of protein supply. Also net protein utilization is higher for preterm formulas compared with human milk, with or without protein supplementation. Technical processes (i.e., hydrolysis) may alter macronutrient concentrations of formula and the pasteurization of human milk before delivery to newborn also cause a reduction in the protein concentration of about 4 % [22].
The whey/casein ratio significantly influences individual amino acid intakes and plasma amino acid concentrations. Plasma threonine increases and tryptophan relatively decreases in infants fed a whey-predominant formula, whereas methionine and aromatic amino acids increase in those fed a casein-predominant formula [23]. The high plasma threonine concentration observed in preterm infants fed whey-predominant formulas is related to the glycomacropeptide obtained from casein by enzymatic casein precipitation of cow’s milk proteins . Acidic precipitation, by contrast, removes the glycomacropeptide rich in threonine from the soluble phase [23]. In preterm infants receiving either an enzymatic or an acidic whey-predominant formula, a significant reduction in plasma threonine concentration was observed in acidic whey protein formula-fed infants, compared to those receiving conventional enzymatic whey protein formulas. All other plasma amino acid concentrations were similar, with the exception of valine, which was slightly reduced in acidic whey protein formula-fed infants [15, 24].
The relative percentage of α-lactalbumin depends on the cow’s milk protein content [25]. This protein fraction is naturally rich in tryptophan and helps normalize the low levels of this amino acid frequently observed in whey-predominant formula-fed infants. The use of a higher percentage of whey in protein-hydrolyzed formulas may worsen the plasma amino acid pattern by increasing threonine and decreasing aromatic amino acid concentrations [25]. Moreover, a significant decrease of plasma histidine and tryptophan concentrations, probably due to a relative reduction in amino acid bioavailability, could be also observed. Using a more appropriate technology, these formulas have been corrected for the threonine content and supplemented with histidine and tryptophan.
An interesting observation is the relatively lower absorption rate of ready-to-use liquid preterm formulas or protein hydrolyzed preterm formulas, where the technical process seems to impair nitrogen absorption in relation to heat treatment—inducing some Maillard reaction—or to preliminary hydrolysis, altering the physiological absorption process in the lumen or at the border of the GI tract. Formulas based on hydrolyzed proteins have been recently proposed for the feeding of preterm infants to reduce GI problems such as delayed gastric emptying, abdominal distension, hard stools, and feeding intolerance [26]. The technological processes of hydrolysis may modify the amino acid content and/or amino acid bioavailability [27]. The efficiency of protein differs also according to non-protein nitrogen contentof different feeding regimens. The highest values were obtained in preterm infants fed powder and liquid preterm formulas . It was significantly lower in those fed protein-hydrolyzed formulas and fortified human milk. The lower value obtained with fortified human milk may be related to nonprotein nitrogen content, which represents 20–25 % of total nitrogen content of human milk, but still 13.5–17 % of total nitrogen content of fortified human milk. The contribution of nonprotein nitrogen fraction of fortified human milk to protein gain is lower than that of the α-lactalbumin or the casein content of human milk [1, 15, 23, 24]. Due to its biological activities, the nonprotein nitrogen of human milk may not be included in protein intakes when human milk is compared to formulas. This may explain why we still frequently observe differences between infants fed human milk or PTF with theoretical similar nitrogen content .
Formulas do not contain any of the biologically active immune proteins nor enzymes, hormones, or growth factors are found in human milk [2]. The long-term implications of this deficiency have not been completely determined. Further long-term randomized studies with higher number of patients, and standardized supplemental amounts and experimental conditions are needed to establish the efficacy of nonnutritional compounds, such as nucleotides, polyamines, and growth factors supplementation in preterm formula [1, 5].
Energy
The energy demands for preterm infants depend on many factors including PMA, genetically determined metabolic rate, thermal environment , activity, sleep status, nutritional status, nutrient intake, body composition, and occurrence of illness [1]. Energy expenditure, measured by indirect calorimetry, increases with postnatal age and varies from 45 to 55 kcal/kg/day. The energy cost for a postnatal weight gain of 17–20 g/kg/day with adequate lean body mass (LBM) accretion vary widely from 50 to 70 kcal/kg/day in premature infants [5, 15, 28]. Energy absorption from enteral nutrition, in healthy growing premature neonates after the first 2–3 days of life, is about 80–90 % of the energy intake. The remainder is lost in stool and a small quantity as urea in the urine. Energy absorbed is in part stored in tissues, mainly as fat but also as protein, and in part spent by the metabolism (energy expenditure). Energy intakes less than or equal to 100 kcal/kg/day may not meet the needs of some preterm infants before discharge. Where PER are adequate (3.2–3.6 g/100 kcal) in a formula providing well-absorbed nutrients, an energy intake around 120 kcal/kg/day is generally appropriate and may result in a fat mass deposition close to intrauterine references. Small-for-gestational-age (SGA) infants, in particular if they experienced intrauterine malnutrition , may need higher energy intake than appropriate-for-gestational-age (AGA) infants [1]. Energy intake recommended for preterm infants is 115–135 kcal/kg/day [5]. All recommendations are related to preterm formula , but energy requirement could differ in preterm receiving human milk in contrast to those fed with preterm formula [22, 28–30] .
Protein:Energy ratio
Protein intake and PER are the main determinants of weight gain [17]. Protein intake is the only determinant of LBM gain in contrast to fat mass gain, which is positively related to energy intake and negatively to PER. Protein and energy needs are reciprocally limiting, the intake of one affecting the ability of the infant to assimilate the other [29, 30]. A suboptimal range of the PER leads to untoward consequences [1]. If energy intake is inadequate, proteins are used as an energy source and the nitrogen balance becomes less positive. Increasing the caloric intake will spare protein loss and improve nitrogen retention. If there is a surfeit of energy with limited protein intake, the protein retention reaches a plateau and the energy excess is used for excessive fat deposition. Thus, an adequate PER should be respected at all PMA, with adequate partition of energy intake between fat and carbohydrates . This crucial point should be respected particularly in the period of clinical instability, when nutritional regimens in a significant part depend on parenteral nutrition [16]. Recommended intakes for preterm infants at 26–30 weeks’ PMA should be 3.8–4.2 g of protein/kg per day with a high PER above 3.2 g/100 kcal and ideally around 3.6 g/100 kcal [5]. A recent experts panel has recommended 3.6–4.1 g/100 kcal in VLBW infants while the European Society for Pediatric Gastroenterology Hepatology and Nutrition (ESPGHAN) have recommended 3.6–4.1 g/100 kcal in ELBW infants and 3.2–3.6 g/100 kcal for other preterm infants < 1800 g [5, 16].
Fat
Fat Requirements
Fat provides the major source of energy in growing preterm infants. Recommended fat intake is 4.8–6.6 g/kg/day [5]. Although some infants with restricted fluid may need high fat content in their feeds to meet energy needs, 4.4–6.0 g/100 kcal is a reasonable range for most preterm infants [16]. Lipid availability by enteral route depends more on digestion and absorption capability than on enteral nutrition content and composition. Digestion of lipids is not fully developed in premature infants [2]. Lipid malabsorption is the result of low levels of pancreatic lipase and bile salts. The bile acid pool is only half the size of that of a full-term infant. Human milk provides additional lipases: lipoprotein lipase and bile salt-stimulated lipase , which contribute to intestinal lipolysis. However, additional fat should be also provided to human milk because of the possible reduction of fat content in expressed human milk, especially when various processes are applied to human milk prior to delivery to neonates [19, 20].
Enteral Nutrition Fat Composition
Fats represent approximately 50 % of the nonprotein energy content of human or formula milk. Fat could be provided in different form with different nutritional power .
Medium Chain Triglycerides
MCTs are hydrolyzed more readily than long-chain triglycerides (LCT) and fatty acids with more double bonds are absorbed more efficiently [16]. In order to improve fat absorption, commercial formulas contain a significant quantity of MCTs that are more easily absorbed than LCTs and transported directly to the liver via the portal vein as nonesterified fatty acids. The use of MCTs instead of LCTs in preterm infant formulas can reduce the formation of calcium and magnesium soaps with unabsorbed long-chain saturated fatty acids and, thereby, increase calcium and magnesium absorption. However, it needs to be stressed that the energy content of MCTs corresponds only to 85 % of that of LCTs, the use of MCTs in formulas induces an increase in plasma ketones as well as in urinary excretion of dicarboxylic acids, suggesting that MCT metabolism could be slightly limited in preterm neonates [16]. Moreover, since MCTs do not contain essential fatty acids, a high MCT intake can reduce the availability of the essential long-chain fatty acids. For these reasons, it is recommended to limit the maximum MCT intake in preterm formula at 40 % of the total fat content [5, 31].
Long-Chain Triglycerides
Because humans cannot insert double bonds at the n-3 and n-6 positions, fatty acids with double bonds in these positions cannot be synthesized endogenously. Therefore, either specific n-3 and n-6 fatty acids or the precursor of each series such as linoleic acid (LA; 18:3n-3) and α-linolenic acid (ALA; 18:2n-6) must be provided as a component of the diet [5, 15] . Both LA and ALA acid are metabolized by a series of desaturation and elongation reactions to more unsaturated longer-chain fatty acids. Important metabolites of these two fatty acids include 20:5n-3 eicosapentaenoic acid (EPA), 22:6n-3 docosahexaenoic acid (DHA), and 20:4n-6 arachidonic acid (AA) [32–34] .
Recent studies have repoKrted outcome data in preterm infants fed milk with a DHA content two to three times higher than the current concentration in infant formulas. Overall, these studies show that providing larger amounts of DHA supplements, especially to the smallest infants, is associated with better neurologic outcomes in early life [34]. DHA intakes of 55–60 mg/kg/day from the time of preterm birth to expected term have been tested and appear to be safe, to promote normal DHA status, and to improve visual and neurocognitive functions. These values are likely to represent an adequate intake for very preterm infants, but further research is needed to confirm that they represent adequate intake for other infants subgroups (i.e., extremely, very, and moderately preterm infants, with or without intrauterine growth restriction , and males and females). Because the maximum DHA content of human milk is 84 mg/kg/day (equivalent of 1.5 % of fatty acids), and because no studies with such a high intake in preterm infants have been reported, no upper limit can be set with certainty.
Synthesis of long-chain LCPUFAs is limited in preterm infants, thus, these molecules are considered as semi-essential or essential. Several studies have suggested that the LCPUFAs supply in preterm infants has a beneficial effect on growth, visual, and cognitive function, as well as on the immune system . Although some methodological issues in these studies do not allow definitive conclusions to be drawn, most of the formulas in Europe and the USA are currently supplemented with LCPUFAs [32–34] .
Recommended intakes are 12–30 mg/kg/day or 11–27 mg/100 kcal for DHA and 18–42 mg/kg/day or 16–39 mg/100 kcal for AA [5]. The ratio of AA to DHA should be in the range of 1.0–2.0 to 1 (wt/wt), and EPA supply should not exceed 30 % of the DHA supply [5, 34]. Recently, an experts panel has recommended (18-) 55–60 mg/kg/day or (16.4-) 50–55 mg/100 kcal for DHA and (18-) 35–45 mg/kg/day or (16.4-) 32–41 mg/100 kcal for AA [16].
Fat Composition of Human Milk
Human milk composition of fat is highly variable and is affected within hours and to a large extent by maternal nutrition intake . Gestation, lactation, parity, milk volume, caloric, and carbohydrate intake, and weight changes are among the maternal factors that can alter the fat content and composition of human breast milk. The lengths of both gestation and lactation affect the lipids that constitute the milk fat globule membrane. Total phospholipid and cholesterol level is higher in colostrum and transitional milk than in mature milk. The period of colostrum lasts less than 10 days, but during this short time the higher lipid levels are beneficial in such processes as neonatal cell membrane production needed for growth, brain development, and bile salt synthesis. Preterm milk may contain a slightly higher proportion of medium- and intermediate chain fatty acids than term milk, which may be advantageous for fat and calcium absorption in preterm infants. LCPUFA are higher in preterm and transitional milk and remain high for the first 6 months in women who deliver preterm. In term milk, on the other hand, LCPUFA declines throughout the first 6–12 months of lactation. Fatty acids rise with a high-carbohydrate maternal diet. Palmitic acid content of breast milk increases in a low-caloric diet. Weight gain during pregnancy is positively associated with higher milk fat content. Milk fat content changes during each feeding. During feedings, foremilk has less fat content than hind milk. Also, the higher the volume of breast milk, the lower the milk fat concentration. Modalities of human milk collection and storage may also influence fat composition. The estimated mean fat content in banked human milk is around 3.2 g/100 ml, somewhat lower than the generally accepted value for mature human milk [35]. This may be related to inadequate emptying of the breast during pumping or to processes applied before delivery bank human milk to neonates.
Pasteurization and storage of banked human milk induces lipolysis, inactivates bile salt-stimulated lipase and lipoprotein lipase, reduces fats, and increases the absolute amount of free fatty acids in pooled samples. Holder pasteurization involves a modification of the biological activity of a large number of enzymes, including lipases [19, 20]. These effects alter the integrity of human milk and may contribute to slower growth in preterm infants fed banked milk versus their mothers’ own milk . On the basis of these evidences, a further supplementation with specific fatty acids could be considered for these infants [34–36].
Carbohydrates
Carbohydrates Requirements
Carbohydrates are essential to provide adequate energy supply and they represent a major source of dietary energy. Based on carbohydrate equivalents of total energy expenditure, the experts have recommended a minimum of 10.5 g/100 kcal and a maximum of 12.0 g/100 kcal [5, 16]. Carbohydrates are essential to provide adequate energy supply. Additionally, carbohydrates promote epithelial cell proliferation, insulin secretion, and calcium absorption [5, 16]. Thus, they are also essential to the overall health of the GI tract other than the fulfillment of energy requirements.
Enteral Nutrition Carbohydrates Composition
The predominant carbohydrate in mammalian milk and in term infant formula is lactose. After digestion by lactase, lactose is absorbed as glucose and galactose, which utilize the same carrier mechanism. In the human fetus, intestinal lactase activity is measurable by 10–12 weeks of gestation [37] . There is a gradual increase in lactase activity with advancing gestation, although the activity remains low until about 36 weeks of gestation, when it reaches the levels seen in full-term neonates [37]. Based on the low lactase activity in early gestation and the estimated length of the bowel, it was calculated that a preterm infant weighing 1300–1400 g might be expected to absorb only 30–50 % of the ingested lactose. The lactose that is not fully digested serves as a source of nutrition for bacterial flora in the colon, where it is transformed into short fatty acids and then absorbed [2]. Through the process of fermentation, this not only facilitates colonic water and electrolytes absorption but also stimulates cell turnover of both the colon and the small intestine. After lactose, oligosaccharides are the largest other carbohydrates component in mature human milk. They represent the third largest solute load of the diet after lactose and fat [37]. Oligosaccharides are hydrolyzed by salivary, pancreatic, and intestinal amylase and maltase to free glucose, which is rapidly absorbed. Many of the protective effects of human milk (i.e., against diarrheal diseases, respiratory, and ear infections) have been attributed to oligosaccharides. A further advantage of oligosaccharides for preterm infant formulas includes the improved gastric emptying [38, 39]. Oligosaccharides may be considered as prebiotics. There are some reports that such prebiotics have beneficial effects on various markers of health. For example, primary prevention trials in infants have provided promising data on prevention of infections and atopic dermatitis. However, additional well-designed prospective clinical trials and mechanistic studies are needed to advance knowledge further in this promising field .
Fluids and Electrolytes
The goal of fluid administration is to replace water loss, maintain water and electrolyte homeostasis to provide extra water and electrolytes to build up new tissues. Total fluid intake is related to ingested caloric and protein intake as well as to the renal solute load [40–44]. Randomized controlled trials on enteral fluid intake of preterm infants are lacking as are studies comparing different fluid volumes providing identical nutrient intakes. From data of combined parenteral/enteral regimens, and assuming full enteral absorption, it follows that fluid volumes between 96 and 200 ml/kg/day are tolerated, and that these values may serve as lower and upper limits [5]. When exclusively enteral feeding is reached, a rate of 150–180 ml/kg/day by standard formula or fortified breast milk should be provided to meet nutrient requirements. Some infants may need higher volumes to meet higher requirements of substrates other than fluid. Preterm infants of less than 35 weeks gestation have obligate high renal and intestinal sodium losses during the first 2 weeks of life, leading to cumulative negative sodium balance in most and hyponatremia in many. It has been demonstrated that increasing dietary sodium intake to 4 mmol/kg/day for infants born at 31–34 weeks, and to 5 mmol/kg/day for those born before 31 completed weeks, prevent the negative sodium balance and hyponatremia and led to more rapid weight gain and earlier discharge from hospital [42, 43]. Premature infants require a higher sodium intake in the first 2 weeks of postnatal life than those born at or near at term, and failure to provide such an intake may predispose to poor neurodevelopmental outcome [43]. Due to the rapid rate of growth and the requirements for intracellular potassium in LBM accretion, potassium intakes are estimated around 2–3 mmol/kg/day and are also high in very preterm infants, like for protein [5, 16] .
Calcium and Phosphorus
Calcium and Phosphorus Requirements
Several nutrients cause a reduction of calcium bioavailability [45–47]. Various factors affect calcium absorption: vitamin D status, solubility of calcium salts, and the quality and quantity of fat intake. In preterm infants, the vitamin D body stores at birth depend mainly on maternal vitamin D status. In the USA, where dairy products are routinely supplemented with vitamin D, a daily additional intake of 400 IU in the formula may sometimes be sufficient to maintain an adequate plasma concentration of 25-OH and 1–25(OH)2 vitamin D. By contrast, in most parts of Europe, cord blood concentration of 25-OH vitamin D of premature infants is frequently less than 10 μg/ml; in this case, up to 1000 IU/day of vitamin D are recommended [5]. The increased needs of premature infants could be partly due to a relative malabsorption of vitamin D, resulting from a low secretion of bile acids [48]. A calcium retention close to 90 mg/kg/day could currently be expected in preterm infants fed preterm formula with a highly soluble calcium content. These values are still lower than the estimated fetal accretion during the last trimester of gestation (100–120 mg/kg/day). However, there are dramatic physiological changes in bone metabolism resulting from various factors after birth: disruption in maternal mineral supply, stimulation of calciotropic hormone secretion, change in hormonal environment, and relative reduction in mechanical stress. These events stimulate the remodeling process leading to an increase in endosteal bone resorption and a decrease in bone density. In preterm infants, these adaptation processes modify the mineral requirement, since, by itself, the increased remodeling and bone turnover provides a part of the mineral requirement necessary for postnatal bone growth [46].
< div class='tao-gold-member'>
Only gold members can continue reading. Log In or Register a > to continue
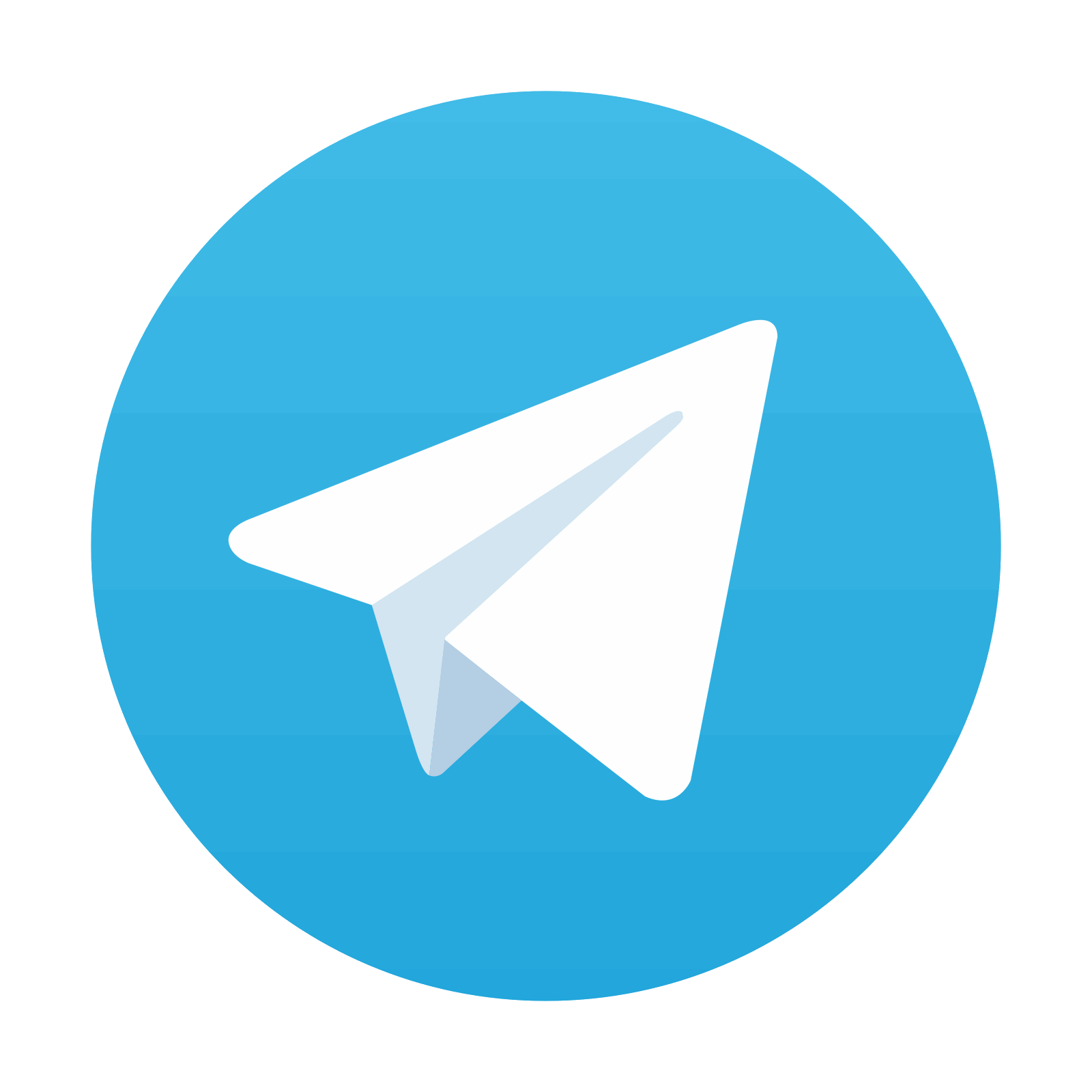
Stay updated, free articles. Join our Telegram channel

Full access? Get Clinical Tree
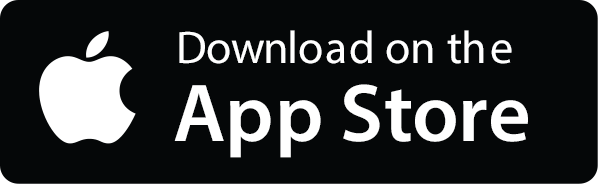
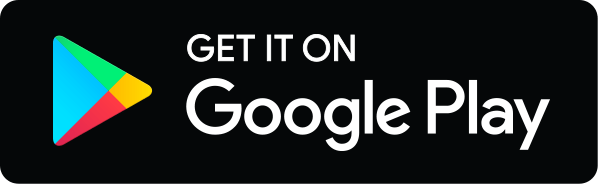