3 Ghalib A. Jibara1 & Michael E. Lipkin2 1 Department of Surgery, Division of Urology, Duke University Hospital, Durham, NC, USA 2 Department of Surgery, Division of Urology, Duke University Medical Center, Durham, NC, USA Innovations and improvements in video imaging technology have paved the way for significant advancements in endourology and minimally invasive urologic surgery. Recent advances in technology have made what once appeared to be science fiction into reality. Augmented reality, three‐dimensional (3D) video imaging, photodynamic and fluorescence‐enhanced optics, and video image overlay are all being incorporated into current surgical practices. These technologies are not only facilitating the performance of complex surgical procedures but are allowing wider dissemination of the skills needed to perform these procedures. As these technologies continue to develop, their applications in minimally invasive endourological practice will only grow. This chapter will review the current state of the art of video imaging systems within endourology. Technological advancements and their application in current endourological practice will be discussed. It is anticipated that this information will not only help the reader to enhance their video imaging practices today, but also prepare one for tomorrow’s tremendous innovations in advanced imaging modalities. There have been a number of major improvements which have greatly enhanced videoendoscopic surgery [1–3]. These significant advances have been the development of the charge‐coupled device (CCD) chip camera and the introduction of digital video imaging. Once an optical image is focused on the CCD chip, there is a conversion of the optical (light) image to an electronic signal [4]. This electronic information, which includes both color and light (luminance), can be scanned by a video monitor to produce an image on the screen. Standard National Television Systems Committee (NTSC) video signals utilize a limited “band width” which include both the color and luminance information in a single or “composite” signal. Problems with this format include “signal noise,” which is caused by the camera having to first process color and luminance information separately and then combine the two pieces of information to create a video signal. This video noise or “crosstalk” may be the cause of decreased resolution, grainy images, and loss of information around the edges of the video image. With the advent of digital imaging, newer video formats have been introduced [5]. The first format allows the color and luminance information to be carried as two separate signals. This “component” video signal is called Y/C (S‐video), with Y standing for luminance or light brightness and C referring to color. This component signal contains less crosstalk and therefore appears cleaner and sharper than images generated by composite signals. The video signal in a Y/C format is carried by a single cable, as with the NTSC format, although the color and luminance information are still separated. Another video format is known as RGB (Red‐Green‐Blue). This RGB format is also a component signal, yet it is distinct from the Y/C format in that the video information (color and luminance) is separated into four signals. These include a red, green, and blue signal as well as a timing signal. Each individual signal carries its own luminance information. Separation of the video signals is performed electronically in the camera head. The RGB format requires less electronic processing than do the NTSC or Y/C formats since the color and luminance information are separate from the start. Therefore, the RGB image quality is greatly enhanced compared to the other two formats. Also, in contrast to the single‐cable NTSC and Y/C formats, the RGB video signal is carried by four separate cables (red, green, blue, and sync). Both Y/C and RGB formats offer superior image quality. The technical advantages created by video monitoring include binocular vision and magnification of the monitor images. Magnification may be as great as 20 times, depending on the initial optics system used. This enlarged image permits better inspection of detail and allows the operator, by virtue of the enlarged image size, to perform the procedure off the monitor rather than looking directly through the endoscope [6]. This approach significantly decreases operator strain because the surgeon no longer needs to conform his body to the angle of the viewing lens. Instead, the urologist can simply attach a video camera to the scope and manipulate the endoscope while standing comfortably and observing the video monitor [3]. Moreover, the upright position assumed by the urologist decreases the likelihood of coming in contact with potentially infected body fluids. All video systems are currently composed of a CCD solid‐state chip camera, a video processor with or without a video recorder, and various video accessories. All of the chip cameras are fully immersible and shielded from electrical interference that may be created by cutting or coagulating currents during endoscopic procedures [7]. The CCD chip high‐resolution camera should have an automatic white balance feature which allows one‐touch color balance assuring true‐to‐life color reproduction. Additional features of the CCD camera may include a high‐gain option which will boost the video signal to provide an exceptionally clear image, as well as a color bar option that allows the surgical team to adjust the monitors to represent accurate camera color prior to beginning the case. Advances in CCD camera technology have significantly downsized current endoscopic video cameras, thereby allowing easier movement of the endoscope with the attached camera. With the recent significant improvements in CCD technology, tube cameras are no longer utilized due to their large size. When recording an actual endoscopic procedure, the camera may provide a remote control switch for a video recorder allowing instant fingertip pausing of the recording device during documentation. Additionally, the camera head should be designed to prevent fogging between the coupling device and the camera head. All manufacturers can supply camera‐head adapters for other endoscopic systems. Finally, the entire camera head cable and edge card should be completely cold‐soakable in disinfectant solutions for easy sterilization between endoscopic cases. As noted, CCD chip cameras have supplanted tube cameras, which were the first video systems on the market. A significant improvement in CCD camera technology has been the development of the three‐chip camera, which contains three individual CCD chips for the primary colors: red, green, and blue (Figure 3.1). In addition to composite SVHS and component signals, the three‐chip cameras also provide an “uncoded” red, green, and blue signal. Color separation is achieved using a prism system overlying the chips [8]. This three‐chip camera design provides improved color fidelity and enhanced image resolution. Moreover, three‐chip cameras produce less “noise” due to the pure red‐green‐blue signals [9, 10]. A digital converter captures each voltage signal as an image and translates the voltage values into discrete numbers, either as 0 or 1. The encoded numbers for each image element, or pixel, include information on color, light intensity, and contrast. These variables can then be modified using image‐processing software within the camera [11]. Figure 3.1 Schematic representation of three‐CCD‐chip and one‐CCD‐chip designs. Red, green, and blue are sent to three separate CCDs by a prism. Reproduced with permission of Olympus. Many CCD chip cameras (both single‐chip and three‐chip) contain electronic shuttering mechanisms that automatically adjust exposure time from 1/60 to 1/16 000 of a second (Figure 3.2). The advantage of such a sophisticated detection circuit is that the camera can react to varying light conditions almost instantaneously, reducing the flaring, “washout,” and “blackout” which were noted with early video systems. Moreover, electronic shuttering mechanisms within the camera usually work more quickly than automatic light sources. Figure 3.2 Traditional technology (top) compared with electronic transmission videoendoscopes (bottom). Reproduced with permission of Olympus. Some of the newer additions to three‐chip camera technology are improved ergonomic designs (light weight and 90° rotation functionality) (Figure 3.3), wireless transmission capabilities when used with wireless monitor systems, and programmable buttons that allow high‐definition (HD) image capture, video recording, image magnification, auto focus, and light source control by the operating urologist. Compatibility with the latest video‐enhancing devices, such as Clarity (Stryker, Kalamazoo, MI, USA) and with modern imaging technology such as narrow band imaging (Olympus, Center Valley, PA, USA) and IMAGE 1S (Karl Storz, Tuttlingen, Germany) further improve visualization and facilitate video documentation. Figure 3.3 The PENDUAL ENDOCAM Logic HD camera head from Richard Wolf. It can be rotated from a 90° angle to a straight camera head which can provide better control in different endoscopic procedures. Reproduced with permission of Richard Wolf GmbH, Knittlingen, Germany. A major advance in the endoscopic systems has been the development of the digital videoendoscope. Miniaturization of chip technology now allows a CCD chip to be incorporated at the distal end of the endoscope (Figure 3.2). Instead of relaying optical images from the objective lens at the distal end of the scope to a camera attached to the eyepiece, the image is immediately captured by the CCD chip, digitized, and converted into electrical signals for transmission (chip‐on‐the‐tip technology). This endoscope design has fewer interfaces allowing the digital information to be directly transmitted to an image display unit with minimal image loss, interference, or distortion [12, 13]. The creation of true videoendoscopes especially benefits the flexible ureteroscope and cystoscope. As internal optics are not required in the long and flexible shafts of these instruments, durable deflection mechanisms can be utilized to improve the durability of flexible endoscopes [14, 15]. With no need to attach a camera head to the eyepiece of the scope, the videoscope cable can be secured to the light cord for attachment to the video system, providing a more lightweight and convenient setup. This technology has been incorporated into larger, rigid endoscopes (laparoscopes), as well as rigid and flexible cystoscopes and ureteroscopes [16–18]. This innovation is due to recent technological advances which have now allowed miniaturization of CCD chips. One can expect to see such an integrated digital videoendoscope to replace many endourologic endoscopes in the near future. Another development in digital camera technology includes the use of a single monochrome CCD chip with alternating red, green, and blue illumination to form a color image rather than using three chips with three separate color filters. This design reduces the space requirements and takes advantage of established high‐resolution monochrome CCD chip technology [12]. Other techniques under development for image resolution enhancement include the use of complementary metal oxide semiconductor (CMOS) technology to replace the CCD sensors [19]. Both CMOS and CCD imagers are manufactured in a silicon foundry and the equipment used is similar. But alternative manufacturing processes and device architectures make the imagers quite different in both capability and performance. It is technically feasible but not economical to use a CCD processor to integrate other camera functions, like the clock driver and signal processing. These are normally implemented into secondary chips. Thus, most CCD cameras comprise several chips. On the other hand, one of the major benefits of CMOS cameras over the CCD design lies in the high level of product integration that can be achieved through virtually all of the electronic camera functions onto the same chips. Typically, a CMOS processor allows lower power usage and lower system cost. This technology is currently being used for digital ureteroscopy [20]. Several in vivo and in vitro studies have compared the new digital flexible cystoscopes to fiber‐optic flexible cystoscopes in terms of their optical characteristics [21–24]. The compared optical characteristics included image resolution, grayscale imaging, color representation, color differentiation, depth of field, image brightness, and the urologist’s subjective assessment of image quality. The digital flexible cystoscopes were superior to fiber‐optic flexible cystoscopes in almost all optical parameters. In addition to the improved imaging quality of the white light digital cystoscopes, the use of optical and video enhancing techniques, such as fluorescence cystoscopy/photodynamic diagnosis (PDD), narrow band imaging (NBI), confocal laser endomicroscopy (CLE), and optical coherence tomography (OCT) have extended the quality of information that these cystoscopes can deliver [25]. The PDD modality, or hexaminolevulinate‐guided blue‐light cystoscopy, uses photosensitive protoporphyrin analogues as intravesical contrast agents combined with a blue‐light‐source (375–440 nm) cystoscope to stimulate fluorescence. Selective accumulation of the photosensitizers by cancer cells causes tissue to appear distinctively red when visualized with blue light cystoscopy (Figure 3.4). There is evidence that the use of this modality improves detection, and consequently treatment, of non‐muscle invasive bladder cancer. A newer body of evidence suggests that blue‐light cystoscopy helps decrease recurrence, and even progression, rates of non‐muscle invasive bladder cancer through better visualization and diagnosis and it has proven to be cost effective [26–29]. Figure 3.4 PPD: small bladder micropapillary lesion. (a) Traditional white light cystoscopy. (b) Blue‐light fluorescence cystoscopy. Reproduced with permission of Elsevier [94]. The NBI technique (Olympus) is based on a light source that filters white light into two narrow wavelengths (415 and 540 nm) that are strongly absorbed by hemoglobin, enhancing the contrast between capillaries and mucosa without the use of dye. Enhanced contrast helps differentiate the more vascularized malignant areas from normal and benign urothelium. The Olympus High Definition Flexible Cysto‐Nephro Videoscope (HD CYF‐VH; Olympus) is equipped with this functionality. In addition to its HD quality images, this cystonephro videoscope has a wide 120° field of view, improved deflection (220° up, 130° down), 150% increased light output, and 20% brighter NBI when compared to the previous model, CYF‐V2. When compared to white light cystoscopes, NBI‐supported flexible cystoscopes have been shown to improve urothelial cancer detection in multiple studies [30–34]. Based on a similar technology, Karl Storz (Tuttlingen, Germany) has recently introduced the Storz Professional Image Enhancement System (SPIES) that is used with their IMAGE 1 S camera unit. Homogenous illumination, color enhancement, and shifting the color spectrum of the image obtained by the digital flexible ureteroscope, Flex‐Xc, offers clearer tissue differentiation through three different viewing options (CLARA, CHROMA, and SPECTRA) that can be displayed side by side with the traditional white light digital image. The SPIES technology is currently at early stages of clinical investigation [35–37]. CLE and OCT are two of the newer imaging modalities. These microscopic modalities are based on fiber‐optic probes used with standard cystoscopes that enable high‐resolution subsurface characterization of suspected lesions, providing information on tissue microarchitecture and cellular morphology that is not possible with traditional white light, PPD, or NBI imaging technologies. The clinical validity and applicability of these technologies are still under investigation [23, 33, 38, 39]. Similar advanced optical technologies have been incorporated into the newer digital ureteroscopes. Use of the CCD and CMOS chip‐on‐the‐tip digital technology has allowed high‐definition visualization, autofocus capabilities, and digital magnification with decreased diameter of the ureteroscope shaft (Figure 3.2). Several studies have compared the currently available digital ureteroscopes with their previous generations and fiber‐optic counterparts in terms of resolution, contrast, depth of field, color representation, illumination, and mechanical properties. The digital ureteroscopes have been shown to have superior visualization and imaging quality compared to the fiber‐optic scopes in all of these studies [40–49]. The available modern flexible digital ureteroscopes are the Flex‐Xc (Karl Storz), the URF‐V2 (Olympus), and the BOA Vision (Richard Wolf, Knittlingen, Germany) (Figures 3.5–3.7). All of these flexible ureteroscopes have one or two integrated light emitting diodes (LED) light source, are capable of auto‐focus and automatic adjustment of light intensity, offer wide (90°) field of view, have a 3.6 Fr working channel and can deflect 270° in both directions. Figure 3.5 The Olympus digital flexible ureteroscope URF‐V2 with chip‐on‐the‐tip technology, NBI, and axial rotation control. Reproduced with permission of Olympus. Figure 3.6 The Karl Storz digital flexible ureteroscope Flex‐Xc with chip‐on‐the‐tip technology, Laserite ceramic insert to decrease laser thermal damage, and the Storz Professional Image Enhancement System (SPIES) modes: (a) CLARA, (b) CHROMA, and (c) SPECTRA A,B). Reproduced with permission of Karl Storz GmbH. Figure 3.7 The Richard Wolf digital flexible ureteroscope BOA Vision with chip‐on‐the‐tip technology and dual LED light source. It is also equipped with Wolf’s image sensor technology at the tip. Reproduced with permission of Richard Wolf GmbH, Knittlingen, Germany. The Richard Wolf’s BOA Vision and the Karl Storz’s Flex‐Xc ureteroscopes use CMOS, while the Olympus URF‐V2 ureteroscope uses a micromini CCD, chip‐on‐the‐tip technology. This technology eliminates the need for fibers to transmit images, which allows for decreased diameter of the scope (8.9, 8.5, and 8.4 Fr for the BOA Vision, Flex‐Xc, and URF‐V2 ureteroscopes, respectively). The Olympus URF‐V2 flexible ureteroscope is equipped with NBI technology that uses different light wavelengths to enhance tissue contrast, as discussed. The role of NBI technology is well established in urothelial carcinoma of the bladder. Although its role in upper tract urothelial carcinoma is theoretically promising, it is still in the early stages of investigation [50]. The Karl Storz Flex‐Xc flexible ureteroscope is equipped with the Karl Storz SPIES technology that changes color enhancement and shifts the color spectrum to produce different image modalities with unique tissue enhancements. The clinical applicability and presumed advantage in detecting upper tract urothelial carcinoma using this technology has not been investigated yet. In addition to SPIES technology, the Flex‐Xc flexible ureteroscope has PDD capability that uses photosensitizers (5‐aminolevulinic acid) to enhance urothelial malignant tissue fluorescence [40]. The use of this technique has been described in few reports and thus its clinical application is still under investigation [37, 51–53]. A newer addition to the flexible digital ureteorscope family is the LithoVue System (Boston Scientific, Marlborough, MA, USA), which is a single‐use digital flexible ureteroscope. This ureteorscope uses CMOS chip‐on‐the‐tip technology. Its light source is built into the handle, which is wired to a touch‐screen monitor that is mounted on a mobile cart for portability (Figure 3.8). The ureteroscope has an outer diameter of 9.5 Fr and a single 3.6 Fr working channel. It can deflect 270° in both directions. Although the visibility and maneuverability of this ureteroscope has been shown to be comparable to those of a fiber‐optic flexible (URF‐P5; Olympus) and a digital ureteroscope (URF‐V; Olympus) in one small study [54], its incorporation into daily clinical practice and cost effectiveness is yet to be explored. Figure 3.8 The Boston Scientific single‐use digital flexible ureteroscope LithoVue with chip‐on‐the‐tip technology. Note the portable display cart that connects to the ureteroscope. Reproduced with permission of Olympus. Laparoscopy has witnessed similar, if not further, advancements in optical technology. HD digital laparoscopes are currently available for use and offer many significant advantages over standard‐definition (SD) laparoscopes. Surgeons have subjectively rated HD images superior to SD images during laparoscopy in both the laboratory and clinical settings [55]
Enhanced Endoscopic Imaging
Introduction
Digital imaging
Standard video systems
Video cameras
Videoendoscopes
Stay updated, free articles. Join our Telegram channel

Full access? Get Clinical Tree
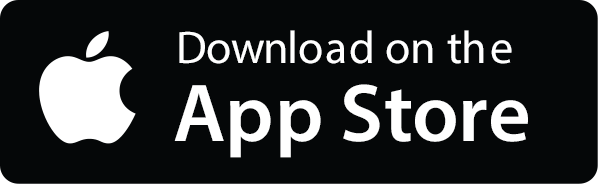
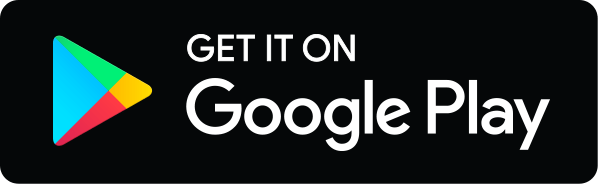