Traditional open surgical repair of thoracic aortic disease is associated with significant morbidity and mortality.
Endovascular stent-graft therapies have emerged as a minimally invasive approach to treating thoracic aortic pathologies.
Stent-graft devices consist of membrane material mounted on an expandable metal stented framework.
Although several imaging modalities have proved useful in planning and executing stent-graft deployment, computed tomography has emerged as the dominant imaging modality because of its wide availability, high resolution, and ability to obtain a variety of three-dimensional reconstructions of the thoracic and abdominal aorta.
Endovascular stent grafts are deployed through sheaths placed in the common femoral or iliac artery. It is often useful to obtain vascular access through an extension conduit anastomosed to the common iliac artery via a retroperitoneal approach.
There are four types of “endoleaks,” or seepage of blood between the walls of the aorta and/or from graft material.
Endovascular stent grafts have been used to repair aortic aneurysms, Stanford type B aortic dissections, penetrating atherosclerotic ulcers, and traumatic aortic transections. The results of these repairs are promising but still are accumulating.
Complications of endovascular stent-graft repairs of the thoracic aorta include spinal cord ischemic injury, retrograde aortic dissection, and iliofemoral arterial injury.
Traditional open surgical repair of thoracic aortic disease continues to be associated with significant morbidity and mortality. Endovascular stent grafts have emerged as an exciting new technology to treat thoracic aortic pathologies. This minimally invasive approach decreases operative risk by minimizing aortic manipulation, avoiding large incisions and surgical hemorrhage, and circumventing the complications associated with cardiopulmonary bypass. This chapter presents the advantages and challenges of thoracic endovascular aortic repair (TEVAR) of the following pathologies of the thoracic aorta: aneurysm, dissection, penetrating atheromatous ulcers, intramural hematomas (IMHs), and traumatic lesions.
Before one begins a review of endovascular repair of thoracic aortic pathology, a review of the current state of open thoracic replacement is required. It is important to draw the clinical distinction between descending thoracic aortic (DTA) replacement and thoracoabdominal aortic (TAA) replacement. The current literature is replete with series of studies of TAA surgery,1 but series detailing the results of lesser thoracic aortic operations are sparse. In DTA replacement, the duration of renal–visceral ischemia is minimal as the thoracic aortic anastomosis often can be accomplished with less than 30 min of aortic interruption. Indeed, if distal perfusion techniques are employed, renal–visceral ischemia may be avoided completely. Thus, extrapolating the series detailing TAA replacement to the arena of DTA replacement overestimates the attendant risk of the pathologies now being addressed by endovascular means. Nonetheless, reviewing the literature of thoracic aortic replacement elucidates the risks both avoided (large cavitary incisions and blood loss) and assumed (paraplegia).
The largest series reporting results of DTA surgery for repair of degenerative aneurysms—not thoracoabdominal aneurysms (TAAs)—was published by Coselli and associates in 2004.2 This series compared the results of surgical repair employing the clamp-and-sew technique with the results of distal aortic perfusion via a left heart bypass (LHB) circuit. Over a 15-year period, 387 patients underwent surgical repair by clamp-and-sew (341 patients; 88.1 percent) or distal perfusion (46 patients; 11.9 percent). Patients requiring profound circulatory arrest because of inability to cross-clamp the aorta (as a result of rupture, large size, or arch involvement) were excluded. Cerebrospinal fluid drainage (CSFD) was used in 24 patients (6.2 percent). The only statistically different preoperative characteristic of the two groups was that the presence of acute dissection was more often associated with the use of LHB (p = 0.02). All other variables were similar between the two groups. Intraoperative variables in the two groups were also similar in regard to the extent of the thoracic aorta replaced, the emergent presentation, and reattachment of intercostal arteries. The LHB patients required a longer cross-clamp time (35.9 ± 10.4 min vs 26.9 ± 9.9 min, p < 0.001), more intraoperative blood products, and had more frequent proximal clamp placement between the left common carotid and the left subclavian than did those treated with clamp and sew (76 vs 54 percent, p = 0.006). Postoperative complications were encountered at similar rates regardless of technique. Thirty-day mortality data revealed 2 percent mortality in the LHB group and 2.9 percent mortality in the clamp-and-sew group (p = 0.1). Renal failure was noted in 7 percent of patients in both groups. Paraplegia rates were quite similar: a striking 4 percent in LHB patients and 2.3 percent in the clamp-and-sew group (p = 0.3). Although the authors hypothesized that patients in the LHB group had a higher risk profile and thus benefited from a subtle protective effect against paraplegia, further propensity analysis failed to support this conclusion. Thus, the application of LHB in patients undergoing thoracic aortic replacement for thoracic aneurysms may be left to the discretion of an experienced aortic surgeon.
Apart from distal perfusion techniques to prevent the dreaded complication of paraplegia, other strategies have been developed to protect the cord from interruption of spinal blood flow. Epidural cooling using an iced saline infusion into the intrathecal space is done to produce a temperature of 25°C to 28°C in the spinal canal, decreasing the neuronal energy requirement.3 The flow rate into the epidural space may be limited by the measured cerebrospinal fluid (CSF) pressure (CSFP), as the target CSF perfusion pressure [mean arterial pressure (MAP)–CSFP] should be above 40 mm Hg.4 Admirable paraplegia rates (6 percent) were reported from the Massachusetts General Hospital in its series of over 300 TAA repairs, a series that included a substantial number of high-risk patients many of whom presented acutely with rupture.5 Commenting on that series, Cambria et al.5 note that the application of local hypothermia avoids the need for systemic moderate hypothermia and the associated coagulopathy. Besides cooling, other mechanisms have been explored to lower spinal metabolism. Acher et al. reported the use of naloxone therapy and barbiturate-based anesthetics to decrease energy expenditure during the critical period of interruption of spinal blood flow.6 In their series of 110 thoracic aortic repairs, an admirable 1.6 percent paraplegia rate was observed with this strategy, despite the deliberate oversewing of intercostal arteries in the critical thoracolumbar zone.
CSFD is perhaps the most studied and widely accepted strategy employed for the prevention of paraplegia in thoracic aortic surgery. Experimental data suggest that CSF pressure may rise with proximal aortic clamping and that reducing that pressure may improve spinal perfusion.3 Thus, placement of a drainage catheter into the subarachnoid space to allow efflux of fluid during the operation and in the postoperative period may benefit the fragile circulation of the spinal cord. Generally, the fluid is able to drain when the CSF pressure exceeds 10 mm Hg as measured at the level of the spine. The beneficial effect of CSFD was demonstrated in a randomized trial comparing repair of type 1 and type 2 TAAs performed with or without CSFD.7 A total of 145 patients underwent repair, with 76 receiving CSFD and the other 69 undergoing repair without CSFD. Aortic clamp times, LHB use, and the number of intercostal artery reattachments were similar between the groups. Nine patients (13.0 percent) in the control group had paraplegia or paraparesis; in contrast, only two patients in the CSFD group (2.6 percent) had deficits. Overall, CSFD delivered an 80 percent reduction in the risk of postoperative spinal ischemic complications after type 1 and type 2 TAATAA repairs. As CSFD catheter placement is not risk-free, it generally is accepted that CSFD is beneficial in operations in which aortic topography may mandate combined spinal and visceral ischemia, which includes most type 1 and all type 2 TAATAAs.
In addition to paraplegia, patients undergoing complex repairs of the thoracic aorta are at high risk of acute renal failure (ARF), an important contributor to postoperative morbidity and mortality. Unfortunately, the incidence of postoperative ARF is high and the consequences are severe. In a review of 234 consecutive repairs for TAATAAs or descending thoracic aortic aneurysms (DTAAs), Safi et al.8 reported a 17.5 percent incidence of renal failure, with 15 percent requiring hemodialysis. Although 86 percent of survivors had resolution of their ARF within 30 days, renal failure was associated with a 49 percent mortality rate. Because of the high incidence and serious consequences of postoperative renal failure, many different interventions have been evaluated to reduce this complication. Although intraoperative treatment with mannitol, dopamine, calcium channel blockers, and various free radical scavengers are common, insufficient data exist to support their use. Indirect perfusion of the kidneys via distal aortic perfusion has been utilized with success, with at least one study demonstrating a protective effect.8 Several authors have reported results involving direct perfusion of the kidneys via cannulation of the renal ostia. Koksoy et al.9 have demonstrated that in patients with type II TAATAAs, direct renal perfusion with hypothermic (4°C) lactated ringers resulted in a three-fold reduction in ARF compared with normothermic blood. Perfusion with cold blood, instead of cold crystalloid, does not offer additional benefit.10
There are a number of approaches to the open replacement of the thoracic aorta. The authors’ approach has been described in detail elsewhere11 and is summarized here. Our operative approach begins with detailed preoperative planning utilizing high-quality imaging, computed tomography angiography (CTA) if possible, to evaluate the aorta for aortic atherosclerotic disease, dense calcifications, and aortic branch ostial disease to properly delineate the optimal sites for proximal cross-clamp, revascularization, and need for renal and/or visceral ostial endarterectomy.
For type I to III TAATAAs, CSFD, and LHB are employed. For type 4 TAATAAs, LHB and CSFD rarely are employed as no data exist to support their efficacy in these groups. The abdominal aorta is exposed via retroperitoneal dissection while the thoracic aorta is approached through a standard, posterolateral incision. The diaphragm is divided in a circumferential fashion to avoid injuring the phrenic nerve. LHB is instituted through the cannulation of the inferior pulmonary vein and an 8-mm Dacron chimney to the left common femoral artery. Distal perfusion is performed with moderate hypothermia (32°C) via a heat exchanger on a Bio-Medicus centrifugal pump. During the opening of the abdominal aorta, the renal artery ostia are flushed with 250 cm3 of cold saline to aid in renal preservation.
To perform aortic reconstruction, the proximal descending aorta is isolated first via a proximal aortic clamp. This anastomosis is followed by subsequent sequential clamping of the aorta during each arterial anastomosis and reimplantation of intercostal, renal, and visceral arteries (Fig. 42-1).
Figure 42-1
Sequential stages of type II TAA repair. In Stage 1, the proximal anastomosis is performed while distal blood flow maintains renal and visceral perfusion. In Stage 2, the graft is clamped distal to the proximal anastomosis while the intercostals are reimplanted. In Stage 3, the graft is clamped distal to the intercostal patch and distal blood flow maintains pelvic perfusion during the renovisceral reconstruction. In Stage 4, the aortic bifurcation is reconstructed. (Reproduced with permission from Black JH 3rd. Technique for repair of suprarenal and thoracoabdominal aortic aneurysms. J Vasc Surg 2009 Oct;50(4):936–941. Copyright Elsevier)

First-generation stent grafts for repair of thoracic aortic pathology were bulky handmade devices that employed pressed Dacron material hand-sewn onto Gianturco stainless steel stents that necessitated large-diameter sheaths (26F) and stiff delivery systems, often requiring that conduits be sewn onto the iliac or abdominal aorta to accommodate the diameter of the delivery device. Newer-generation devices offer improved flexibility with small introducer systems. The US Food and Drug Administration (FDA) approved the first endovascular device for the treatment of degenerative thoracic aneurysms in late 2004.
There are currently three FDA-approved devices commercially available for endovascular repair of the thoracic aorta: the TAG endoprosthesis (W.L. Gore and Associates, Inc, Flagstaff, AZ), the Zenith TX2 TAA Endovascular Graft (Cook, Inc, Bloomington, IN), and the Talent Thoracic Stent Graft System (Medtronic Vascular, Santa Rosa, CA). All three devices, the TAG, the Zenith, and the Talent endoprostheses, consist of a series of self-expanding metal stents (either nitinol or stainless steel) affixed to tube grafts [made of polytetrafluoroethylene (PTFE), polyester, or woven graft, respectively]. Although the TAG device consists of single tube graft, the TX2 and the Talent are designed as two-piece systems with proximal and distal ends so that a combination of multiple proximal pieces and a distal piece can be utilized depending on the characteristics of the patient’s individual aorta. In addition, the proximal component of the Talent device consists of several uncovered nitinol springs that allow the device to be deployed across a vessel (the left subclavian or left common carotid artery) without impeding blood flow. The treatable lengths and diameters for each device are listed in Table 42-1.
Parameter | TAG | Zenith TX 2 | Talent Thoracic |
---|---|---|---|
FDA-approved indication | DTAA | DTAA and PAU | DTAA and PAU |
Treatable aortic diameters | 23–37 mm | 24–38 mm | 18–42 mm |
Length of proximal and distal landing zones | ≥20 mm | ≥25 mm | ≥20 mm |
Percent oversizing to aorta | 6–19% | 10–20% | 18–42% |
Required sheath delivery size | 20, 22, 24F | 20, 22F | 22, 24, 25F |
A wide range of diagnostic tests are helpful in confirming suspected thoracic aortic pathology, including chest radiography, aortography, transthoracic echocardiography (TTE) or transesophageal echocardiography (TEE), magnetic resonance imaging (MRI), computed tomography (CT), and recently serum biomarkers. The benefits and limitations of each of those methods are reviewed below, along with their applicability to aneurysmal and dissection pathology.
Classically, widening of the cardiac or aortic silhouette is cited as indicative of aortic disruption, along with pleural effusions and the displacement of aortic calcifications. Specifically, widening of the aortic silhouette was found in 60 to 90 percent of cases of aortic dissection by Hagan and coworkers in their review.12 However, chest radiography has limited specificity and sensitivity.
This modality has both high sensitivity (86–88 percent) and high specificity (75–96 percent) for the diagnosis of aortic dissection.13 Findings suggestive of dissection include distortion of the normal contrast column, flow reversal or stasis in a false channel, failure of major branches to fill, and aortic valvular regurgitation.13 For the diagnosis of thoracic aortic aneurysm and treatment planning, aortography may improve the accuracy of length measurements of intended aortic seal zones (Fig. 42-2). In addition, aortography may be of benefit in defining aortoiliac occlusive disease, which may require angioplasty or a planned conduit for access for the stent-graft delivery. Nonetheless, enthusiasm for this modality is tempered by the potential for diagnostic confusion about malperfusion after pressurized injection, the risk of contrast nephropathy, the time delay to diagnosis, and the expense.
TTE has a sensitivity of 35 to 80 percent and a specificity of 40 to 95 percent for the detection of aortic dissection,14 whereas the sensitivity of TEE is reportedly as high as 98 percent, with 63 to 96 percent specificity.15 TTE may be limited technically by body habitus, emphysema, and narrow intercostal spaces. Although TEE is more invasive, it expands the diagnostic ability of echocardiography, potentially demonstrating entry tear sites, flow within the false lumen, involvement of the arch or coronary arteries, degrees of aortic valvular regurgitation, and pericardial effusions. However, one must be mindful of its limitations in imaging the anatomic blind spot in the distal ascending aorta and arch secondary to the air-filled trachea and left mainstem bronchus. In addition, TEE cannot image aortic pathologies past the diaphragm.
MRI has unparalleled sensitivity and specificity for detecting aortic pathology, for instance, 95 to 100 percent for dissection.16 Recent advances in three-dimensional (3-D) reconstruction of gadolinium-enhanced magnetic resonance angiography (MRA) are beneficial in planning thoracic aortic stent-graft treatment in patients whose comorbidity profile includes renal insufficiency. However, this enthusiasm must be tempered by the realities of long examination times, inability to monitor critically ill patients, expense, and lack of immediate availability. Moreover, MRI is contraindicated for patients with implants or pacemakers.
CT offers multiple benefits and is the most commonly used diagnostic test in patients with suspected aortic pathology. Readily available, CT scanning carries a sensitivity of 83 to 95 percent with a specificity of 87 to 100 percent for the detection of acute aortic dissection.17 This modality can be particularly helpful in examination of the true versus the false lumen. A slit-like compressed true lumen should heighten suspicion for branch vessel compromise and malperfusion syndrome substantially (Fig. 42-3). In addition, CTA has evolved so that multiple reconstructions can be created from the source axial images, rendering this diagnostic modality vital for preoperative planning.
Serum biomarkers, particularly D-dimer, represent an emerging diagnostic tool for detecting aortic dissection, particularly for screening patients with chest pain in whom high-quality, axial imaging would be prohibitively expensive. When the aortic media tears, the extrinsic pathway is triggered resulting in the cross-linking of fibrin. D-dimer is a degradation product of this cross-linking reaction and thus represents a potential biomarker of aortic dissection. A cutoff value of <500 ng/mL can be used to rule out aortic dissection, yielding a negative likelihood ratio of 0.07 and a negative predictive value of 95 percent.18 However, up to 18 percent of patients with confirmed aortic dissection will not have a D-dimer level greater than 400 ng/mL19 likely secondary to the variability of serum levels based on the time since dissection. Thus it is currently most useful to rule out patients who are considered low risk.
Endovascular repair of thoracic aortic pathology requires facility with the interpretation of axial imaging such as CTA and MRA to inspect aortic topography for device insertion and fixation. In addition, planning of thoracic aortic endografting may be aided by conventional diagnostic arteriography, using both subtracted and unsubtracted techniques, especially when 3-D imaging cannot be obtained from available axial data. A key tenet of thoracic aortic endovascular repair is the understanding that device selection and operative planning should be confirmed and verified to the greatest extent possible preoperatively as errors in device measurements often translate into immediate treatment failures.
Insertion planning for endovascular devices requires a thorough understanding of the aortic diameters at the proximal and distal sealing sites as measured from outer wall to outer wall. The indications and sizing guidelines for the current FDA-approved devices are summarized in Table 42-1.
Oversizing the graft too aggressively may place undue forces on the aortic endograft and result in migration or collapse of the entire graft.20 The length of proximal fixation should be more than 20 mm (or 25 mm in the case of the Zenith device) with an acceptable aortic diameter throughout this seal zone. If an adequate proximal fixation length is not immediately obtainable, the left subclavian artery (LSA) can be crossed and excluded with little consequence to the patient (mean left arm pressure decreased, with few symptoms); if the patient has a prior left internal mammary-to-coronary bypass, a left-carotid-to-left-subclavian bypass is required to maintain coronary circulation before coverage. Further encroachment into the arch generally requires salvage of the left common carotid circulation by a prior carotid–carotid bypass. If excessive tortuosity is encountered in either fixation zone, a longer seal length of up to 30 mm may be needed to prevent blood from escaping around the ends of the stent (type 1 endoleak). When multiple devices are required to cover long lengths of aortic pathology, each piece should be made to overlap 3 to 5 cm with the neighboring endograft. The distal aortic seal zone is usually cephalad to the celiac axis.
A common theme in complications of aortic endografting is the occurrence of damage to access vessels such as the common femoral and iliac arteries. Therefore, if the measured diameter of an intended access vessel is demonstrated to be less than 8 mm, serious consideration should be given to a surgical conduit. To create a conduit, 10-mm Dacron conduits generally are anastomosed to the common iliac or abdominal aorta via a miniflank incision with retroperitoneal exposure of the vessel. In particular, severe calcification of an access vessel may increase the risk of tear, perforation, dissection, or frank rupture. It is not uncommon for such iatrogenic injuries to become obvious when the device sheath is being withdrawn, not at the time of sheath insertion. As the device sheath is removed, the obturation of the injury is relieved and hemorrhage ensues.
The procedure of aortic endografting for thoracic aortic pathologies should be performed in an operating room with a high-resolution digital imaging system and a radiolucent table. The choice of anesthetic generally is based on preoperative cardiac risk stratification and may include local, epidural, and general anesthesia. The application of CSF drainage should be considered when coverage of the entire thoracic aorta is planned or if a prior abdominal aortic aneurysm (AAA) repair has been performed. Grafts are inserted via cutdown on the intended access vessel, most commonly the right common femoral artery. After this surgical exposure, systemic anticoagulation is achieved, using heparin to maintain an activated clotting time (ACT) greater than 250 s. An angiographic catheter is inserted percutaneously via the opposite common femoral artery or brachial artery to the level of the aortic arch. Using digital subtraction angiography, aortic arch or thoracic aortic angiography is performed to determine the approximate positioning of the endoprosthesis. To view the landing areas in the arch, it often is necessary to view the arch with a 60-to-90 degree left anterior oblique (LAO) projection. To view the distal seal zone, a similar steep, nearly lateral projection best depicts the level of the celiac axis (Fig. 42-4).
To minimize manipulation, particularly in the arch, the final diagnostic studies before deployment should be performed with the device nearly in place; the stiffness of the wires and delivery systems may alter the course of the aortic arch and affect the landmarks being used to mark the proximal limits of deployment. “Road-mapping” techniques or “fluoro-fade” may improve operator accuracy in proximal fixation. Maneuvers to minimize stroke include flushing of all air from the device sheaths and lumens carefully. Also, manipulation of stiff wires into and from the arch should be done carefully under fluoroscopic guidance. After deployment, fixation should be improved by using a large-diameter compliant occlusion balloon to maximize apposition of the stent graft to the aortic seal zones and regions of device overlap in a distal-to-proximal order (Fig. 42-5). The access sheath is removed carefully, with attention paid to MAP as an early indicator of hemorrhage from iatrogenic insertion injury. If a tear in a vessel is suspected, the sheath can be reintroduced to obturate the injury. If the sheath will not pass, an occlusion balloon can be passed proximal to the injury and inflated to achieve vascular control. Frank transections usually require a flank incision and reconstruction, whereas small tears or dissections often are salvaged by endovascular stent placement without conversion.
In light of the preponderance of iatrogenic access injuries, the authors aggressively apply retroperitoneal conduits via a flank incision. Most patients undergo general anesthesia both for flexibility of access approach and to avoid patient movement at critical imaging moments. The operations are performed in an endovascular operating room with a ceiling-mounted imaging device.
With an estimated incidence of 6 to 10 cases/100,000 person-years, a thoracic aortic aneurysm carries a substantial risk of mortality. Coady and associates21 found a 27.9 percent total incidence of rupture or dissection for DTAAs larger than 6.0 cm. The long-term survival of patients with untreated DTAAs at 1 and 5 years was 88 and 6 percent, respectively.21 As with AAAs, the yearly risk of rupture or dissection for DTAAs has been found to correlate with size: 2 percent for small aneurysms, 3 percent for aneurysms 5.0 to 5.9 cm, and 6 percent for those equal to or larger than 6.0 cm. The risk of death along with rupture and dissection increases markedly at 6.0 cm: 6.5 percent yearly for aneurysms 5.0 to 5.9 cm but 14.1 percent for those equal to or greater than 6.0 cm.22
The results of TEVAR in DTAAs are summarized in Table 42-2.23–26 In the table, endoleak refers to the seepage of blood between the previously sealed walls of the aorta and graft material. Endoleaks are divided into four types: Type I endoleaks occur as a consequence of a poor seal between the aorta and23 the proximal or distal attachment sites of the graft, type II endoleaks are characterized by back bleeding from branch vessels, type III leaks involve blood excursion between multiple components of grafts, and type IV endoleaks occur when blood transgresses from the lumen through the material of the graft itself.
Dake (1994) | Czerny (2004) | Makaroun (2008) | Matsumura (2008) | |
---|---|---|---|---|
Technical success | 100% | 94.4% | 98% | 98.8% |
30-day mortality | 0% | 3.7% | 2.1% | 1.9% |
Complication rate | 7.7% | 1.9% | 57.9% | 41.9% |
Paraplegia | 0% | 0% | NR | 1.25% |
Endoleak | 30.8% | 28.9% | 21% | 13% |
Mean follow-up | 11.6 months | 38 months | 37 months | 12 months |
Long-term survival | 100% | 92.6% | 68% | 91.6% |
The use of stent grafts to treat DTAAs was pioneered by Dake et al. in 1994.25 Although endovascular repair previously had been described for AAAs, subclavian artery aneurysms, arteriovenous fistulas, and femoral occlusive disease, this group from Stanford extended the technology to the thoracic aorta. Their series of 13 patients had 100 percent technical success and no mortality nor paraplegia. The authors reported that two patients had “small patent proximal tracts communicating with the aneurysm” that resolved over the ensuing months and that two more patients required a second procedure to eliminate “residual filling from either a proximal communication or a distal communication,” presumably type I endoleaks (30.8 percent). In light of those promising early results, the authors advocated endovascular treatment for highly selected patients.
The first long-term outcomes were reported by Makaroun et al.26 who reported the midterm follow-up results of the Gore TAG trial. This trial compared 191 patients who underwent endovascular repair of DTAAs with the TAG device (51 patients were enrolled after redesign of the endograft device) to 94 patients who underwent traditional, open repair. At 5-year follow-up, patients undergoing endovascular treatment had a decreased rate of aneurysm-related mortality (2.8 vs 11.7 percent, p = 0.008) and a similar rate of all-cause mortality (68 vs 67 percent, p = 0.433). TEVAR was associated with a lower incidence of major adverse events in the first postoperative year (42 vs 77 percent, p < 0.001) and these patients were less likely to have bleeding, pulmonary, renal, neurologic, or wound complications; however, TEVAR patients were more likely to have vascular complications at 5 years. Endoleaks, predominantly type I, occurred in 21 percent of patients who underwent endovascular surgery, and 3.6 percent patients in the endovascular group required postoperative reintervention. Only one patient required conversion to an open procedure 73 days after endovascular treatment secondary to the detection of an aortoesophageal fistula. At 5 years, the aneurysmal sac size had decreased in 50 percent of patients and increased in only 19 percent. In addition, at 5 years, there were no ruptures, no stent-graft collapse, and only one incident of significant stent migration. These encouraging long-term results lead the authors to conclude that TAG thoracic endografting is not only a safe and effective method of repairing DTAAs, but superior to open repair in terms of aneurysm-related morality.
Although initial trials were performed with the TAG device, additional trials have since followed utilizing other devices. Matsumura et al.23 evaluated the Zenith TX2 device, comparing 160 patients who underwent endovascular repair of their DTAA to 70 patients who underwent traditional, open replacement. Although the 30-day and 1-year survival rates were similar between the endovascular and open groups, patients undergoing TEVAR had fewer cardiovascular, pulmonary, and vascular adverse events (41.9 vs 68.9 percent, p < 0.01). Endovascular repairs were complicated by a similar rate of reintervention as those undergoing open repair. In addition, at 1 year, there were no aneurysm ruptures, a 3.9 percent incidence of endoleaks, and a 2.8 percent incidence of graft migration. This study demonstrated that endovascular repair with the TX2 device is a safer and equally effective alternative to open surgical repair for DTAAs.
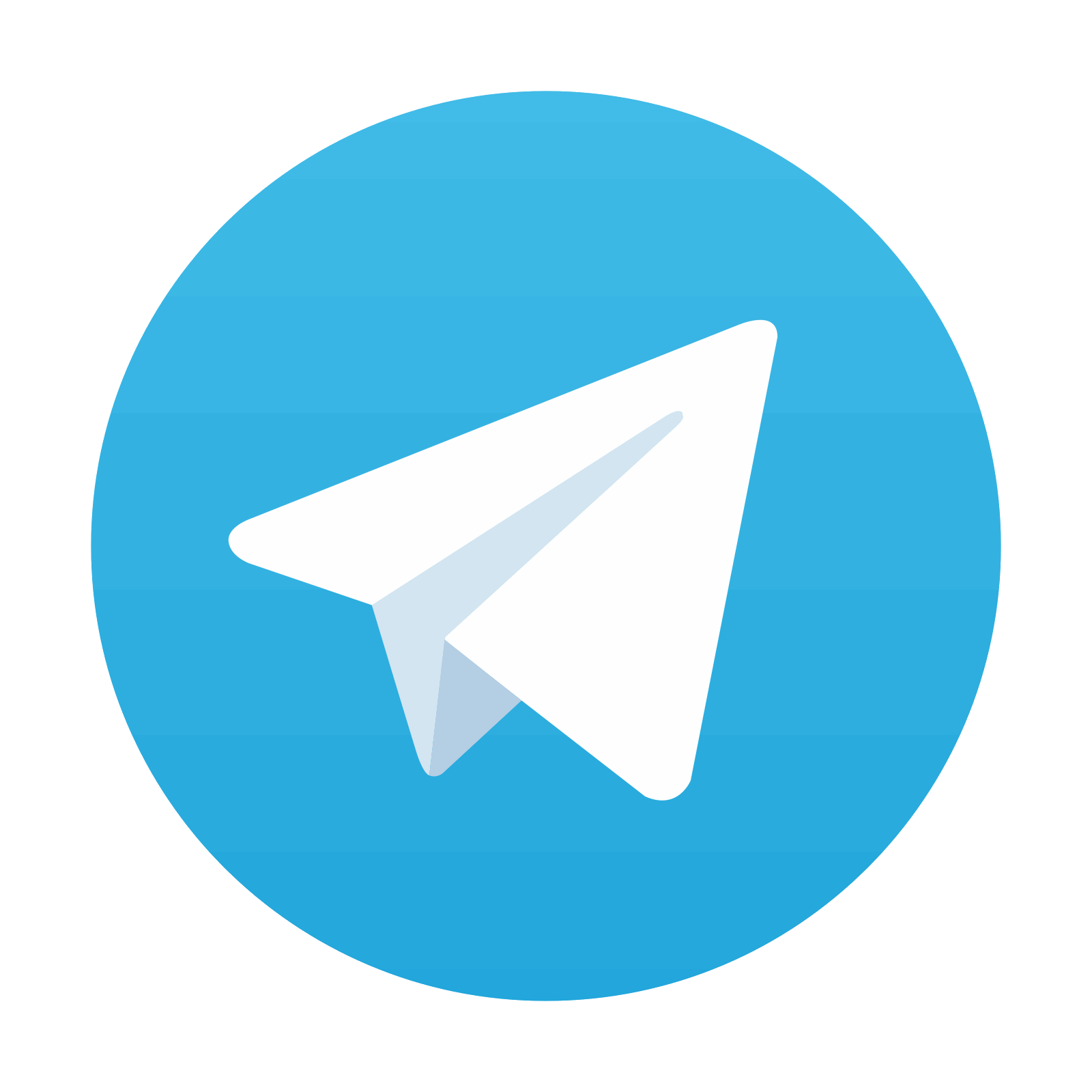
Stay updated, free articles. Join our Telegram channel

Full access? Get Clinical Tree
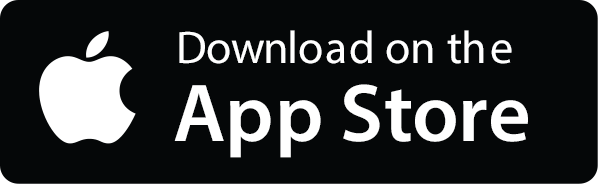
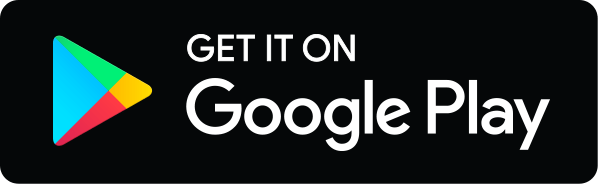