Outline
Introduction 167
The Concept of Endothelial Progenitor Cells 167
Endothelial Progenitor Cells as an Indicator of Cardiovascular Disease 168
Early Outgrowth Cells: Myeloid Endothelial Progenitor Cells 168
Circulating CD34 + (KDR + ) Endothelial Progenitor Cells 168
Endothelial Progenitor Cells and Colony-forming Units 169
Microvascular Remodeling by Circulating Cells 170
Endothelial integrity is a critical factor for long-term organ survival. In the conditions of prolonged redox stress, as occur in renal disease, endothelial repair mechanisms may be a determinant of such integrity. Apart from local endothelial repair, involvement of circulating progenitors has been postulated. Although a role of such circulating progenitor cells has been clearly demonstrated, it remains unclear whether there are prespecified progenitor populations that can do this. Moreover, it also remains controversial whether such progenitor cells become endothelial cells themselves or rather contribute through remodeling of the microvascular environment. Current thinking postulates that both CD34+ hematopoietic stem cells and myeloid progenitor cells may be involved in vascular and endothelial regeneration. This chapter will discuss the current methods used to identify and enumerate progenitor cell populations in patients with renal disease, and their potential role in renal disease progression.
Introduction
As are many other organs, the kidney is capable of self-maintenance under physiological conditions. On a daily basis, epithelial cells are shed in the urine and replaced. Likewise, after tubular necrosis, the tubular epithelium can be fully regenerated. More recently, it has been postulated that even podocyte loss can be repaired from the parietal epithelial niche. Many insults that lead to epithelial damage are associated with endothelial injury and activation.
For example, during ischemia–reperfusion injury endothelial cells become physically detached and rarefaction of the capillary bed in the kidney may occur . Recent data suggest that the endothelial–pericyte interaction may play an important role in development of renal fibrosis and progression of renal disease. Endothelial activation may drive a regulatory phenotype of pericytes, which is involved in stabilization of the microvascular network, into a myofibroblast phenotype that leads to extracellular matrix deposition . Endothelial integrity and control of endothelial function thus may be important determinants of renal repair processes and, when they fail, of renal disease progression. Careful labeling studies with proliferation markers such as Ki67 have demonstrated, even in healthy subjects, the presence of a very high glomerular and peritubular and endothelial cell turnover. In fact, the proliferation index doubles that of the tubular epithelial cells . This is likely to be a reflection of ongoing microvascular repair. Consequently, endothelial regenerative capacity may be limited owing to subsequent telomere shortening and replicative senescence. This repair mechanism may even be further compromised in renal disease, which is usually characterized by increased redox stress in the vasculature. Such redox stress has been associated with the development of senescence by itself .
In recent years, attention has been drawn to circulating cells that could serve as a potential source for endothelial repair and regeneration . These cells are often referred to as endothelial progenitor cells (EPCs). However, it turns out that this concept is largely based on different in vitro systems used to characterize these cells. Consequently, phenotypically different circulating cells have been coined EPCs and their in vivo relevance may differ from model to model. This does not distract from the fact that such circulating cells, either as a direct source for endothelial repair or by indirectly modulating the microenvironment of the vasculature, could be of great importance in endothelial repair and preservation of kidney function. This chapter reviews the various populations that have been proposed as EPCs, the methods used to identify them and their respective in vivo importance.
The Concept of Endothelial Progenitor Cells
In 1997 Asahara et al. performed a seminal experiment that led to the concept of EPCs. Using magnetic beads coated with an antibody to CD34, they isolated mononuclear blood cells and plated them on fibronectin. After 7 days the investigators found that these cells, when cultured under conditions that favor endothelial cell growth, differentiated into an endothelial-like phenotype. In addition, they demonstrated that labeled CD34 + cells in vivo could home to sites of ischemia where they appear to incorporate into the microcirculation and favor recovery of ischemia . The idea behind these experiments is that the hematopoietic lineages and the endothelial lineages may share a common ancestor in embryology and that in this way this concept of EPCs would recapitulate this phenomenon. Indeed, recent lineage tracing experiments demonstrated in both zebra fish and mice that hematopoietic stem cells originate from a subset of endothelial cells of the aortic floor .
The concept of repair by EPCs was subsequently expanded by a vast array of studies usually involving a bone marrow chimera model where, in models varying from cancer to ischemic heart disease, incorporation of bone marrow cells could be demonstrated . In the renal field incorporation of endothelial cells that apparently were derived from a bone marrow precursor population were found in models of renal disease such as anti-Thy1 glomerular nephritis and Adriamycin (doxorubicin) nephrosis . The functional importance of such incorporation was demonstrated by the fact that administering bone marrow cells therapeutically could enhance the recovery of anti-Thy1 glomerular nephritis . Along the same lines, inhibition of apoptosis in Adriamycin nephrosis resulted in increased incorporation of bone marrow cells in the peritubular capillaries, leading to preservation of renal perfusion. This was associated with a reduction in interstitial fibrosis .
Endothelial Progenitor Cells as an Indicator of Cardiovascular Disease
Based on the aforementioned observations, clinicians became interested in measuring EPCs as a potential marker of vascular repair. Unfortunately, a somewhat confusing situation has developed where three different assays have been advanced to assess the number of EPCs. These assays probably reflect a different biology of circulating cells and are hard to relate to the actual in vivo biology of vascular repair.
Early Outgrowth Cells: Myeloid Endothelial Progenitor Cells
When peripheral blood mononuclear cells are isolated by Ficoll gradient centrifugation and cultured on fibronectin-coated tissue culture plates in vascular endothelial growth factor (VEGF)-enriched medium, spindle-shaped cells appear after 3–4 days. These attaching cells incorporate acetylated low-density lipoprotein (LDL), bind various lectins such as Ulex europaeus agglutinin and express endothelial markers, such as von Willebrand factor, eNOS or CD31. They display proangiogenic capacities in animal models, notably in ischemic hindlimb , and the enumeration of these attaching cells has been associated with the risk of developing coronary heart disease . Even though these cells have been described as EPCs, the phenotypic characterization of the attaching cells is usually not consistent with that of a mature EPC phenotype. For example, markers that are frequently used include the uptake of acetylated LDL and the presence of CD31. Both markers, however, are also present on myeloid cells. Indeed, using lineage tracing methods with endothelial nitric oxide synthase (eNOS) green fluorescent protein (GFP) transgenic mice, it was recently demonstrated that the majority of the endothelial-like cells in this assay are derived from myeloid precursor cells . There are also indications that part of the endothelial markers in the attaching cells could be derived from microparticles taken up from endothelial cells or platelets, thus further defying the concept that the endothelial properties of these cells can be defined by surface markers .
Although angiogenic properties have been attributed to these cells when exposed in vitro to VEGF, the circulating myeloid precursors appear to lack such angiogenic properties. Urbich et al. used both freshly sorted human CD14 + cells from Ficoll-isolated human peripheral blood mononuclear cell (PBMCs) and CD14 + -derived EPCs (cultured in VEGF-enriched medium) to inject into nude mice that underwent hindlimb ischemia. They showed significant neovascularization after 2 weeks in the mice receiving the CD14 + EPCs compared with the mice that received no cells or fresh CD14 + . Moreover, they used CD14 − subfractions and cultured under the same conditions as CD14 + to generate EPCs. These CD14 − -derived EPCs also showed neovascularization properties . These results strongly suggest that myeloid cells per se are not capable of inducing angiogenesis, but need a proangiogenic stimulus to acquire this property.
Circulating CD34 + (KDR + ) Endothelial Progenitor Cells
Another method used to identify EPCs is to detect circulating cells according to the expression of their surface markers by flow cytometry.
CD34 + cells can be isolated from human peripheral blood through magnetic beads cell sorting. Dead cells are excluded by propidium iodide, and possible contaminating monocytes are excluded by gating out the CD14 + cells. Cells are subsequently incubated with monoclonal antibodies against the extracellular parts of VEGFR-2 or CD133. Granulocyte colony-stimulating factor (G-CSF) can be used to stimulate the mobilization of CD34 + cells from the bone marrow (from 0.4% to 2.0% of the CD34 + population) to enhance sensitivity of the measurement. Other groups used peripheral blood directly with the monoclonal antibodies, resulting in a count of 0.03% of circulating PBMCs.
During fluorescence-activated cell sorting (FACS) analysis, circulating progenitor cells are characterized as cells located in the lymphocyte gate (based on forward-side scatter) and expressing CD34 + and CD45 dim . Some research groups also add the presence of the VEGF receptor-2/KDR or the stem cell marker CD133 to characterize the progenitors further. It is customary to express the number of CD34 + cells per 10 3 CD45 + leukocytes.
Numerous studies have shown a correlation between circulating CD34 cells, thus measured, and cardiovascular risk. In addition, most forms of renal disease are associated with reduced numbers of these cells ( Table 10.1 ). It is, however, doubtful whether such reduced numbers also reflect reduced vascular repair. Clearly, CD34 + cells that have been primed in vitro with endothelial cell growth factors exert proangiogenic effects. However, whether naïve CD34 + cells can rescue in vivo ischemia is less clear.
EPC type | Context | Readout | Findings | Improved | Ref. |
---|---|---|---|---|---|
Myeloid | HD | Number of colonies, migratory capacities | ↓ colonies, positive correlation with kT/V | VEGF improves migratory capacities | |
KTx | Number of colonies cultured with patients’ serum | More colonies with serum from graft with CrCl > 50 ml/min | |||
ESRD | Number of colonies | ↓ colonies | 8 weeks of rhEPO | ||
ESRD | Number of colonies from patients and cultured with patients’ serum | ↓ colonies in ESRD or with ESRD serum | HD | ||
ESRD | Number of colonies and angiogenic properties | ↓ colonies, decrease tube formation | Worsened by HD | ||
KTx | Number of colonies, migration assay | Improvement of migratory capacities | KTx | ||
ESRD | CD14 + EPC count | Non-significant increase with decline in GFR | |||
CD34 + | ESRD | Peripheral count of CD34 + KDR + | CD34 + KDR + increases with eGFR | ||
KTx | Peripheral count of CD34 + | Decreased in ESRD compared to KTx | |||
ESRD | Peripheral count of CD34 + | Transient increase under rhEPO | rhEPO | ||
ESRD | Peripheral count of CD34 + | Decreased circulating cells | HD | ||
ESRD | Peripheral count of CD34 + KDR + | CD34 + KDR + decreased in number | EPO | ||
KTx | Peripheral count of CD34 + , CD34 + KDR + and CD34 + CD133 + | CD34 + and CD34 + CD133 + decreased in number | KTx | ||
ESRD | Peripheral count of CD34 + | Decreased in number with age, urea and Pi levels | HD, statins | ||
HD | Peripheral count of CD34 + | Decreased number compared to healthy control and genomic damage | Just after HD session | ||
HD | Peripheral count of CD34 + | Decreased number correlates with PTH and phosphate values |
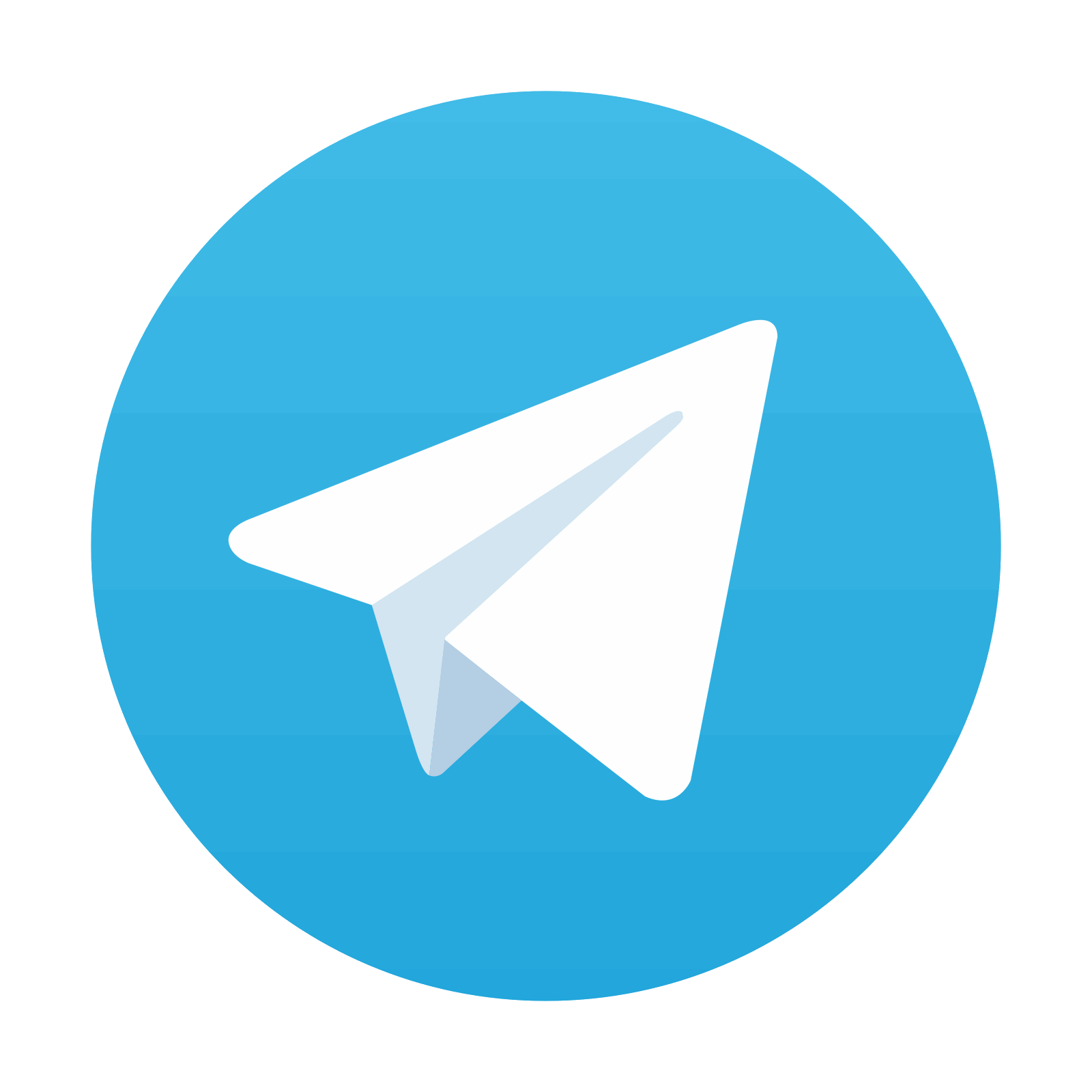
Stay updated, free articles. Join our Telegram channel

Full access? Get Clinical Tree
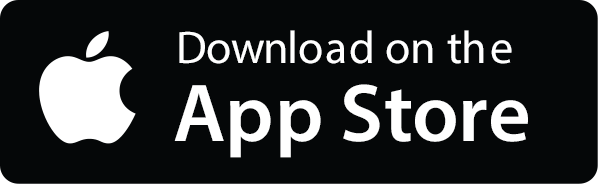
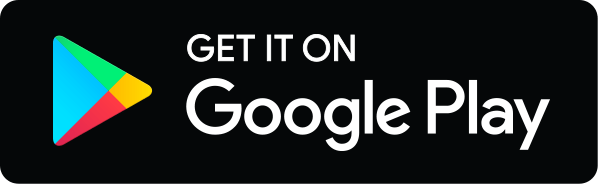
