Electron Microscopy
Taofic Mounajjed, MD
5.1 INTRODUCTION
The rise of immunohistochemical, molecular, and serologic techniques in pathology over the past decades has somewhat sidelined certain diagnostic uses of the more traditional ultrastructural pathology1, 2, 3; however, electron microscopy remains a powerful tool in the evaluation of liver disease, both for research and for diagnosis. This chapter considers clinical conditions where ultrastructural analysis is particularly useful. It also briefly reviews correct retrieval and processing methods for electron microscopy, focuses on the ultrastructural features of nonneoplastic liver conditions most commonly evaluated by electron microscopy, and when relevant, discusses their differential diagnoses. Of course, this brief chapter cannot be all-inclusive to the vast field of ultrastructural liver pathology, but attempts to cover the most clinically relevant issues to the practicing surgical pathologist.
5.2 FIXATION METHODS FOR ELECTRON MICROSCOPY
Electron microscopy images are the outcome of a complicated and time-consuming processing of liver tissue. Interpretation of electron microscopy images requires, in addition to knowledge of the hepatic ultrastructure, experience with potential artifacts of fixation, embedding, sectioning, contrast staining, and microscopic imaging. If the clinician or pathologist has deemed that a liver biopsy might require ultrastructural evaluation, adequate tissue submission and processing is essential at the time of the biopsy. Small specimens (no larger than 1 mm3 for optimal fixative penetration) should be submitted separately for electron microscopy.
Fixation
Fixation arrests cell metabolism and stops autolytic processes that start within minutes or even seconds following specimen retrieval.4 Fixation also leads to crosslinking of organelles and substructures, which preserves them. For these reasons, fixation of tissue intended for electron microscopy use should be done as immediately as possible in order to achieve better visualization of ultrastructural detail, avoid artifacts, and prevent poor preservation or injury to organelles, which could
mimic true pathology. Fixatives should be isotonic and isothermic to the cells4; although different labs have their own preferences, 1.5% to 2.5% buffered gluteraldehyde is universally accepted.
mimic true pathology. Fixatives should be isotonic and isothermic to the cells4; although different labs have their own preferences, 1.5% to 2.5% buffered gluteraldehyde is universally accepted.
Table 5.1 Ultrastructural features that can be used to judge the quality of tissue preservation | ||||||||||||||
---|---|---|---|---|---|---|---|---|---|---|---|---|---|---|
|
Although perfusion fixation methods have proven optimal for electron microscopy studies, this method is somewhat difficult to accomplish in needle biopsies, which generally undergo immersion fixation and have results of sufficiently good quality for diagnosis.4, 5, 6, 7 In the case of needle biopsies, two 3 mm × 1 mm cylinders of tissue are cut from the end of the tissue core, immersed immediately in fixative, then cut into pieces measuring no more than 1 mm in greatest dimension each. The small tissue fragments should be placed in glutaraldehyde fixative (for at least 1 to 2 hours) at room temperature. Tissue can be refrigerated but should not be frozen. The quality of tissue fixation can be inferred from certain ultrastructural features (Table 5.1)4,6,8,9; these features should be evaluated in each clinical case in order to avoid misinterpretation of fixation artifacts. In poorly fixed tissues, common artifacts include nuclei with serrated uneven edges, ballooned mitochondria, myelin-like whorls in mitochondria, and dense deposits in mitochondrial matrix.
Processing after fixation
After glutaraldehyde fixation, specimens are subjected to a washing buffer, osmium postfixation, ethanol dehydration, and embedding. Samples then undergo drying via critical point drying (routinely used) or hexamethyldisilazane-drying and are then mounted on stubs and sputter coated with 10 nm gold for scanning electron microscopy.10,11 For transmission electron microscopy, thick sections (0.5 to 1 µm) are produced, stained with Toluidine Blue, and examined for optimal tissue selection. Then, 40 to 100 nm sections (ultrathin sections) are produced from Epon-embedded tissue, spread on mesh copper grids and stained with a uranyl acetate and lead citrate solution for ultrastructural evaluation.
Visualization
Toluidine Blue-stained thick sections provide a useful histological introduction to further detailed study by transmission electron microscopy. They allow examination of relatively large areas of tissue to determine the quality of fixation and to identify areas that are the most representative or have the most pronounced pathology for further trimming. In general, it is best to focus the study of immersion fixed needle biopsies on the outer and middles zones of the tissue, which are the best fixed.
Scanning electron microscopy offers more details about the endothelial lining (fenestrae) and the space of Disse. Therefore, scanning electron microscopy preparations are very useful for judging the condition of sinusoids,12 though they do not allow for detailed evaluation of intracellular structures. Transmission electron microscopy, on the other hand, allows detailed examination of the fine structure of the various cell types present in the liver, and is therefore the primary tool used for diagnostic purposes in ultrastructural pathology; it can allow magnifications of up to 1,000,000×, with a resolution of about 5 nm. Transmission electron microscopy sections are surveyed first at low magnification (as low as 50×), which functions as a bridge between Toluidine Blue light microscopy and high-power transmission electron microscopy examinations. This survey provides information about the quality and content of the sections and allows for preselection of interesting areas for further examination.
Electron microscopy in formalin fixed paraffin electron microscopy bedded tissue
Formalin fixation results in poor tissue preservation; it leads to tissue extraction, especially affecting lipids, glycogen, ribosomes, membranous structures, and other cellular and intercellular matrix materials. Tissue preservation can be especially poor in tissue fixed by Bouin fixative.13 Paraffin electron microscopy bedding per se, on the other hand, has little effect on the ultrastructure. Several factors can improve fine structure of paraffin electron microscopy bedded tissues, such as careful thin slicing and judicious selection.
Although preservation of fine structures in formalin fixed paraffin electron microscopy bedded tissue is generally imperfect, compactly organized cells with abundant cytoplasmic contents, such as hepatocytes, are usually better preserved than other tissue types. In comparison with glutaraldehyde fixation, formalin fixation leads to the following ultrastructural alterations13:
The tissue becomes less homogenous in electron density (exaggerated contrast).
The tissue has a pale and extracted appearance.
Details are compromised at higher magnification, resulting from the uneven loss of phospholipids, membranous structures, matrix, and ribosomes.
The cell membranes of discohesive cells, such as Kupffer cells, frequently show discontinuities at high magnification.
There is loss of mitochondrial detail. The mitochondria appear contracted or distended and partly disintegrated. The cristae are poorly preserved.
Among cytoplasmic contents, lipid, glycogen, and ribosomes are poorly preserved.
Among organelles, the smooth endoplasmic reticulum and Golgi apparatus are poorly preserved.
The cytoplasm shows irregular rarefactions representing lost liposomes, glycogen, endoplasmic reticulum, Golgi apparatus, and vesicles.
Lipofuscin shows moderate alterations.
Cell nuclei show peripheral condensation of their chromatin, with incomplete preservation of the nuclear membranes. The nuclear pores are difficult to identify.
The following structures show the least amount of alterations13:
Cellular borders, including cilia and microvilli, are well defined.
Intercellular junctions, such as desmosomes are well preserved.
Closely packed rough endoplasmic reticulum is well preserved.
Lysosomes, including large secondary lysosomes with phagocytized material, are usually well preserved.
Collagen and basement membranes are well delineated.
Microfilaments, such as seen in the pericanalicular region, are relatively well preserved.
Although many authors believe that transmission electron microscopy is a futile exercise in formalin fixed paraffin electron microscopy bedded tissue, others have found surprisingly well-preserved fine structure and others have even been able to identify viruses and other microorganisms by transmission electron microscopy.13, 14, 15, 16, 17, 18 In fact, transmission electron microscopy can be useful if separately submitted tissue in glutaraldehyde is not available. In such instances, the use of formalin fixed paraffin electron microscopy bedded tissue for transmission electron microscopy can provide the benefit of a more integrated approach, linking the light microscopic and ultrastructural findings. In our experience, transmission electron microscopy can be useful diagnostically in liver pathology, especially in conditions involving identification of abnormally accumulated materials (e.g., glycogen storage disease, lysosomal storage disorders, amyloid, and identification of hepatocellular inclusions). Abnormalities that require detailed examination of the delicate structure of small organelles (e.g., mitochondrial cytopathies), on the other hand, can be more difficult to ascertain in formalin fixed paraffin electron microscopy bedded tissues.
Artifacts
Artifacts can result at any step (from operative to photography) and should always be kept in mind when examining electron microscopy images. It can be very difficult to distinguish between genuine structures and artifacts.4 Common artifacts include large endothelial gaps, cell shrinkage, widening of the space of Disse, changes in cell and/or nuclear shape, mitochondrial swelling, blebbing of membranes, and vesicle formation in intercellular spaces.6
5.3 THE NORMAL LIVER
In order to understand ultrastructural liver pathology, knowledge of the normal structures is essential. The next section provides a brief description of the fine structure of the different cell types seen in the liver.
The hepatocyte
The hepatocyte is the predominant cell type in the liver, accounting for approximately 60% of the total cell population19,20 and approximately 80% of the liver volume.21 Ultrastructurally, the hepatocyte has a polyhedral appearance, with quadrangular to hexagonal shapes in cut sections. The average hepatocyte diameter is 25 µm.20 Approximately 55% of the hepatocellular cytoplasmic volume is cytosol.20,21 The cytoplasm of the hepatocyte is remarkable and unique for being essentially packed with numerous organelles (Fig. 5.1), including mitochondria, peroxisomes, Golgi apparatus, smooth endoplasmic reticulum, rough endoplasmic reticulum, lysosomes, microtubules, microfilaments, and intermediate filaments. Glycogen abounds in the cytoplasm of normal hepatocytes and is predominantly in rosette (rather than monoparticulate) form, and distributed diffusely, but has an association with the smooth endoplasmic reticulum.22,23 Lipid droplets can be found in small numbers under normal conditions within the cytosol (Fig. 5.2), especially near the sinusoids; lipid has a rounded, electron dense, and overall homogeneous appearance. Lipofuscin and hemosiderin pigment are also not uncommon (Figs. 5.3 and 5.4).
Polarity
The normal hepatocytes do not rest on a basement membrane, but they do have polarity (Fig. 5.5), featuring sinusoidal, lateral, and canalicular regions. The sinusoidal pole (Fig. 5.6), comprising approximately 40% of the hepatocellular surface, is in contact with the space of Disse and is covered by microvilli. Owing
to the high level of endocytosis on this surface, the underlying cytoplasm contains many pinocytotic vesicles.
to the high level of endocytosis on this surface, the underlying cytoplasm contains many pinocytotic vesicles.
![]() Figure 5.1 The normal hepatocyte. Normal hepatocytes (upper center) have a polyhedral appearance, with voluminous cytoplasm, abundant glycogen contents and numerous organelles. |
![]() Figure 5.2 The normal hepatocyte. Lipid droplets can be found in small numbers within the cytoplasm of hepatocytes under normal conditions. |
The lateral pole (Fig. 5.7), which is opposed to a neighboring hepatocyte, is straight and lacks microvilli. It contains various types of intercellular junctions (saccular unions, gap junctions, desmosomes, and intermediate junctions),20,24, 25, 26, 27, 28 which provide
cell-to-cell communication,29,30 and tight junctions that seal the bile canaliculi.
cell-to-cell communication,29,30 and tight junctions that seal the bile canaliculi.
![]() Figure 5.3 The normal hepatocyte. Lipofuscin consists of coarse, electron-dense granules, lipid droplets, and a heterogeneous matrix. |
![]() Figure 5.4 The normal hepatocyte. Hemosiderin consists of lysosomal aggregates of finely granular electron-dense material. |
The canalicular pole (Fig. 5.8) is sealed on both sides by tight junctions, delineating it from the lateral pole. The canalicular pole constitutes approximately 15% of the hepatocellular surface and, like the sinusoidal pole, contains microvilli. The pericanalicular ectoplasm, an organelle-free cytoplasmic zone that surrounds the bile canaliculus, is rich in microfilaments, which facilitate canalicular bile motility.31,32
Mitochondria
Mitochondria are the most numerous organelles in the hepatocyte (Fig. 5.9), accounting for approximately 18% of the hepatocyte volume.20,33 They are bound by a double membrane with many shelf-like projections protruding from the inner membrane, known as “cristae” (Fig. 5.10). The lumen of mitochondria contains a “matrix” that is slightly more electron dense than the hepatocellular cytosol in normal hepatocytes. Matrical dense granules are commonly seen in normal mitochondria. Mitochondrial functions are numerous and involve cell respiration, oxidative phosphorylation, electron transport, ATP synthetase activity, urea cycle, fatty acid oxidation, and the citric acid cycle.20
The endoplasmic reticulum
The endoplasmic reticulum, a convoluted network of continuous membrane-bound cisterns, tubules, and saccules, constitutes approximately 15% of the hepatocellular volume.21 It consists of the rough endoplasmic reticulum (60%, studded with ribosomes) and smooth endoplasmic reticulum (40%), which are in continuity with one another. Rough endoplasmic reticulum (Fig. 5.11) forms parallel stacks usually located around the nucleus, toward the vascular and biliary poles of the hepatocyte.
The rough endoplasmic reticulum is the site of protein synthesis, especially proteins for secretion.34 The tubules of the smooth endoplasmic reticulum (Fig. 5.12) are devoid of ribosomes, and are located more toward the periphery of the hepatocyte; smooth endoplasmic reticulum is usually associated with rich collections of glycogen.20,35, 36, 37 The smooth endoplasmic reticulum is the site of remarkable metabolic activity, including cytochrome P450 (located in endoplasmic reticulum membranes)20; its functions include lipid synthesis, glycogen and glycoprotein metabolism, bile acid synthesis, and drug detoxification. The latter function is reflected ultrastructurally by the expansion and shrinkage of the smooth endoplasmic reticulum in response to administration and withdrawal, respectively, of certain medications.20,35,38, 39, 40, 41
The rough endoplasmic reticulum is the site of protein synthesis, especially proteins for secretion.34 The tubules of the smooth endoplasmic reticulum (Fig. 5.12) are devoid of ribosomes, and are located more toward the periphery of the hepatocyte; smooth endoplasmic reticulum is usually associated with rich collections of glycogen.20,35, 36, 37 The smooth endoplasmic reticulum is the site of remarkable metabolic activity, including cytochrome P450 (located in endoplasmic reticulum membranes)20; its functions include lipid synthesis, glycogen and glycoprotein metabolism, bile acid synthesis, and drug detoxification. The latter function is reflected ultrastructurally by the expansion and shrinkage of the smooth endoplasmic reticulum in response to administration and withdrawal, respectively, of certain medications.20,35,38, 39, 40, 41
The Golgi apparatus
The Golgi apparatus is a group of layered (4 or more layers) parallel, curved, and flattened saccules, with dilated peripheral rims associated with clusters of vesicles. The Golgi apparatus’s main function is to modify secretory molecules arising from the smooth endoplasmic reticulum and rough endoplasmic reticulum (by processes such as glycosylation and sulfation) and sort them into individual chemically distinct vesicles, and then release them for delivery and fusion with target membranes.20,42 The concave (aka trans) region of the Golgi apparatus is rich in very low-density lipoprotein vesicles and lysosomes.43,44
Lysosomes
Lysosomes are derived from the Golgi apparatus. They are membrane-bound organelles containing pleomorphic electron-dense material (Fig. 5.13). The pericanalicular region of the hepatocyte is the richest region in lysosomes.20,44,45 The main lysosomal function is removal of cellular waste; they contain numerous enzymes that degrade the material within them; enzyme deficiencies result in various lysosomal storage disorders.46 Such abnormal accumulations within lysosomes often allow at least broad categorization into a metabolic disease group based on the ultrastructural appearance of the deposits (e.g., phospholipidosis); but further classification often requires additional clinical and molecular characterization. Lysosomes are seen in hepatocytes under normal conditions; they represent normal cell turnover. Lipofuscin granules are lysosomes containing coarse, electron-dense granules, lipid droplets, and a heterogeneous matrix; they increase in hepatocytes with increased patient age.
Peroxisomes
Peroxisomes (Fig. 5.14), whose functions include hydrogen peroxide metabolism and oxidation of long chain fatty acids,47 occupy approximately 2% of the total hepatocellular cytoplasmic volume.20 Peroxisomes are bound by a single membrane and have a finely granular and homogeneous matrix. They are seen usually in association with smooth endoplasmic reticulum, but can also have random localization
within the cytoplasm.48 They are more numerous in Zone 3 hepatocytes. It is essential to examine electron microscopy images methodically for the presence of peroxisomes within hepatocytes in every case; the absence of peroxisomes, characteristic of Zellweger syndrome, can be otherwise easily missed.
within the cytoplasm.48 They are more numerous in Zone 3 hepatocytes. It is essential to examine electron microscopy images methodically for the presence of peroxisomes within hepatocytes in every case; the absence of peroxisomes, characteristic of Zellweger syndrome, can be otherwise easily missed.
The nucleus
Nuclear abnormalities are seldom of diagnostic importance in the current practice of liver ultrastructural pathology; however, a brief description is provided of the hepatocellular nuclear fine structure. The hepatocellular nucleus is large, round, and is surrounded by two membranes separated by “the perinuclear cisterna.” The membranes meet periodically, creating many nuclear pores (3,000 to 4,000 per cell).20 The outer membrane is continuous with the rough endoplasmic reticulum; the perinuclear cisterna is also in continuity with the cisterna of the rough endoplasmic reticulum.49 The nucleus has one or more 8 to 10 µm nucleolus; the mean number of nucleoli per hepatocyte is 2.7.20 Nuclear inclusions such as glycogen inclusions and lipid inclusions (Figs. 5.15 and 5.16) can be seen in pathologic conditions, but can occasionally be seen in normal hepatocytes as well.20 In addition to being occasionally seen in normal hepatocytes, nuclear glycogenosis can be seen in various conditions including diabetes mellitus, Wilson disease, Gilbert syndrome, Rotor syndrome, glycogen storage disease, and total parenteral nutrition.
![]() Figure 5.15 The normal hepatocyte: nuclear inclusions. Nuclear inclusions such as glycogen inclusions are often seen in pathologic conditions, but can occasionally be seen in normal hepatocytes. |
The cytoskeleton
The hepatocellular cytoskeleton has three components:
Microfilaments. In hepatocytes, microfilaments, whose main function involves motility, are composed mostly of actin filaments. The microfilaments abound in microvilli and are located beneath the cell membrane, especially
around the bile canaliculus.50, 51, 52 In the pericanalicular region, microfilaments are visible as an organelle-free ectoplasmic zone, which acts as miniature musculature for the bile canaliculi, facilitating bile flow.32,53,54
around the bile canaliculus.50, 51, 52 In the pericanalicular region, microfilaments are visible as an organelle-free ectoplasmic zone, which acts as miniature musculature for the bile canaliculi, facilitating bile flow.32,53,54
Intermediate filaments. Intermediate filaments constitute the cytoskeletal framework of hepatocytes and consist mainly of cytokeratin fibrils. Of note, Mallory bodies are pathological alteration of the hepatocellular intermediate filaments (see Alcoholic liver disease).55, 56, 57, 58
Zonal differences in hepatocellular ultrastructure
The hepatocytes have different functions depending on their location in the lobules, such as increased bile acid uptake in Zone 1 hepatocytes and drug detoxification in Zone 3 hepatocytes. These specialized functions are also reflected by some ultrastructural differences. The following zonal differences are noteworthy20:
1. Increased and more oval-shaped mitochondria in Zone 3 hepatocytes.
2. More abundant rough endoplasmic reticulum in Zone 1 hepatocytes.37
3. More abundant smooth endoplasmic reticulum in Zone 3 hepatocytes.
4. Increased peroxisomes in Zone 3 hepatocytes (can outnumber mitochondria) (Fig. 5.17).
5. Larger bile canaliculi with more prominent microvilli in Zone 1 hepatocytes.37
6. Increased hemosiderin-filled lysosomes in Zone 1 hepatocytes.
The sinusoids
The sinusoidal lining allows direct bathing of hepatocytes with plasma, although protecting them from the mechanical trauma of blood flow.8,9,61 The following cells constitute the structure of the sinusoids and space of Disse (Figs. 5.18 and 5.19).
The endothelial cells
Endothelial cells are separated from hepatocytes by the space of Disse. The space of Disse is occupied by hepatocellular microvilli, some collagen fibers, and hepatic stellate cells. Endothelial cells are flattened and elongated and have numerous cytoplasmic projections separated by characteristic fenestrae. The latter allow the endothelium to act as a filtration barrier, permitting contact between the hepatocytes and plasma, although excluding the cellular components of the blood and protecting the hepatocytes from trauma.20
Another characteristic feature of sinusoidal endothelial cells is the presence of numerous pinocytotic pits and vesicles. Each endothelial cell is loosely attached to its neighboring endothelial cells by junctions.62 Unlike other endothelia, the sinusoidal endothelium lacks a basement membrane.9
Another characteristic feature of sinusoidal endothelial cells is the presence of numerous pinocytotic pits and vesicles. Each endothelial cell is loosely attached to its neighboring endothelial cells by junctions.62 Unlike other endothelia, the sinusoidal endothelium lacks a basement membrane.9
![]() Figure 5.17 The normal hepatocyte: zonal differences. Peroxisomes can be increased in Zone 3 hepatocytes, sometimes outnumbering mitochondria. |
The hepatic stellate cells
Although usually indiscernible by routine light microscopy in normal livers, hepatic stellate cells are relatively easy to identify ultrastructurally in the space of Disse (Fig. 5.20), where they tend to occupy perisinusoidal recesses. There is approximately one hepatic stellate cell per 20 hepatocytes, and they tend to be more abundant in Zone 3. The hepatic stellate cell in its inactivated state has a round to oval, eccentrically placed nucleus, and many large lipid droplets occupying the bulk of the cytoplasm.
Kupffer cells
Kupffer cells occupy one-third of the endothelial volume.20 They have a pyramidal or star shape (Figs. 5.21 and 5.22), with many cytoplasmic pseudopodia and are rich in lysosomes. They are more frequently observed in Zone 1 and their primary function is phagocytosis. Many lysosomal storage disorders manifest mostly in Kupffer cells (see Lysosomal storage disorders).
![]() Figure 5.20 Hepatic stellate cells. Hepatic stellate cells have round to oval, eccentrically placed nucleus, and many large lipid droplets occupying most of the cytoplasm. |
Biliary epithelial cells
Unlike hepatocytes, biliary epithelial cells (Figs. 5.23 and 5.24) rest on a well-formed basement membrane, which completely surrounds the bile duct. They have basally located nuclei and are smaller and have less cytoplasm than hepatocytes. Their mitochondria are sparse and smaller than those of hepatocytes, and have characteristic cristae, which traverse the entire mitochondrion rather than being shelf-like. Numerous microvilli project into the bile duct lumen and many pinocytic vacuoles underlie the luminal plasma membrane of the biliary epithelial cell. Desmosomes between neighboring cells are clustered near the apical border, and apical tight junctions are present as well. The most relevant ultrastructural abnormalities evaluated in routine practice involve hepatocytes and Kupffer cells; evaluation of biliary epithelial fine structure seldom reveals additional critical ultrastructural findings.
5.4 GENERAL FEATURES IN ULTRASTRUCTURAL HEPATOPATHOLOGY
Before discussing specific disease conditions, it is important to identify common abnormalities seen in electron microscopy images and understand their significance.
Hepatocellular injury
In general, ultrastructural features of hepatocellular injury follow this sequence of events: cytoplasmic edema, mitochondrial swelling, cristolysis, flocculated mitochondrial matrix, clumping of nuclear chromatin, nuclear apoptosis (characterized by chromatin margination and fragmentation), rough endoplasmic reticulum degranulation, dense deposits in mitochondria and peroxisomes, and, finally, cell
necrosis. In practice, general ultrastructural patterns of hepatocyte injury include the following:
necrosis. In practice, general ultrastructural patterns of hepatocyte injury include the following:
1. Ballooning degeneration (Fig. 5.25) reflects ongoing hepatocyte damage.63,64 Of note, the term ballooning degeneration, when used in ultrastructural descriptions, refers to extensive dilatation of the endoplasmic reticulum and is a different finding than the ballooning seen on hematoxylin and eosin (H&E) in diseases, such as fatty liver disease. In electron microscopy studies, ballooning is characterized by marked dilatation of the rough endoplasmic reticulum with detachment of the ribosomes, sometimes to a degree that hinders distinction between the rough endoplasmic reticulum and the smooth endoplasmic reticulum. The dilated rough endoplasmic reticulum often displaces other organelles. Although ballooning degeneration is considered a hallmark of viral hepatitis, it can be seen in many other conditions. As this pattern of hepatocellular damage continues, it can be followed by injury of the plasma membrane, which results in flattening of the villi and bleb formation (especially at the vascular pole), and by mitochondrial changes (swelling, low matrical density, fragmentation of cristae, and loss of matrical granules); these latter ultrastructural changes indicated an irreversible damage to the hepatocyte.63
![]() Figure 5.25 Hepatocellular injury: ballooning. Ballooning degeneration is characterized by marked and diffuse dilatation of the endoplasmic reticulum |
2. Focal cytoplasmic degeneration (Fig. 5.26) is a pattern of hepatocellular injury that is characterized by necrosis of a portion of the hepatocellular cytoplasm, which is recognized ultrastructurally by the appearance of empty spaces in the cytoplasm, followed by breaking down of organelles and accumulation of amorphous electron-dense and granular material in the affected areas. Foci of focal cytoplasmic degeneration have irregular margins and vary in size; they are initially surrounded only by well-preserved organelles but eventually become bound by a distinct membrane, eventually producing an autophagic vacuole, then a cytolysosome.
3. Oxyphilic/oncocytic change (Fig. 5.27) is characterized by increased mitochondria, some of which might show minor changes such as reduction of dense granules. It is considered to represent an adaptive change in response to various stimuli, including hypoxia and toxic injury. It is not a specific change because it can be seen in viral hepatitis, drug reactions, and in up to 50% of livers with cirrhosis.65
![]() Figure 5.27 Hepatocellular injury: oncocytic change. Hepatocytes with oncocytic change are packed with numerous mitochondria. |
4. Acidophil bodies, in contrast to ballooning degeneration, are characterized by cytoplasmic water loss, with cells becoming shrunken because of plasma membrane injury.63,66 In addition to shrinkage, the cell loses its microvilli and plasma membrane junctions, resulting in detachment from neighboring hepatocytes.64,67 Acidophil bodies are eventually engulfed by macrophages.
Cholestasis
Given that electron microscopy is frequently performed to evaluate hyperbilirubinemia in infants, cholestasis is commonly encountered in liver samples. Furthermore, electron microscopy is extremely sensitive in detecting cholestasis; signs of hepatocellular bile retention can be seen in the absence of clinical or biochemical evidence of jaundice.68, 69, 70, 71 For instance, focal subcellular cholestasis can be seen in asymptomatic gallstone patients whose livers are otherwise normal clinically, by laboratory testing, and by routine histology.68,69,71
The ultrastructural features of cholestasis include20 the following changes:
1. Retained bile pigment in hepatocellular (Figs. 5.28 and 5.29) and Kupffer cell lysosomes. Occasionally, the biliary pigment in hepatocytes can be associated with cholesterol deposits (e.g., in cases of total parenteral nutrition), mimicking cholesteryl ester storage disease.
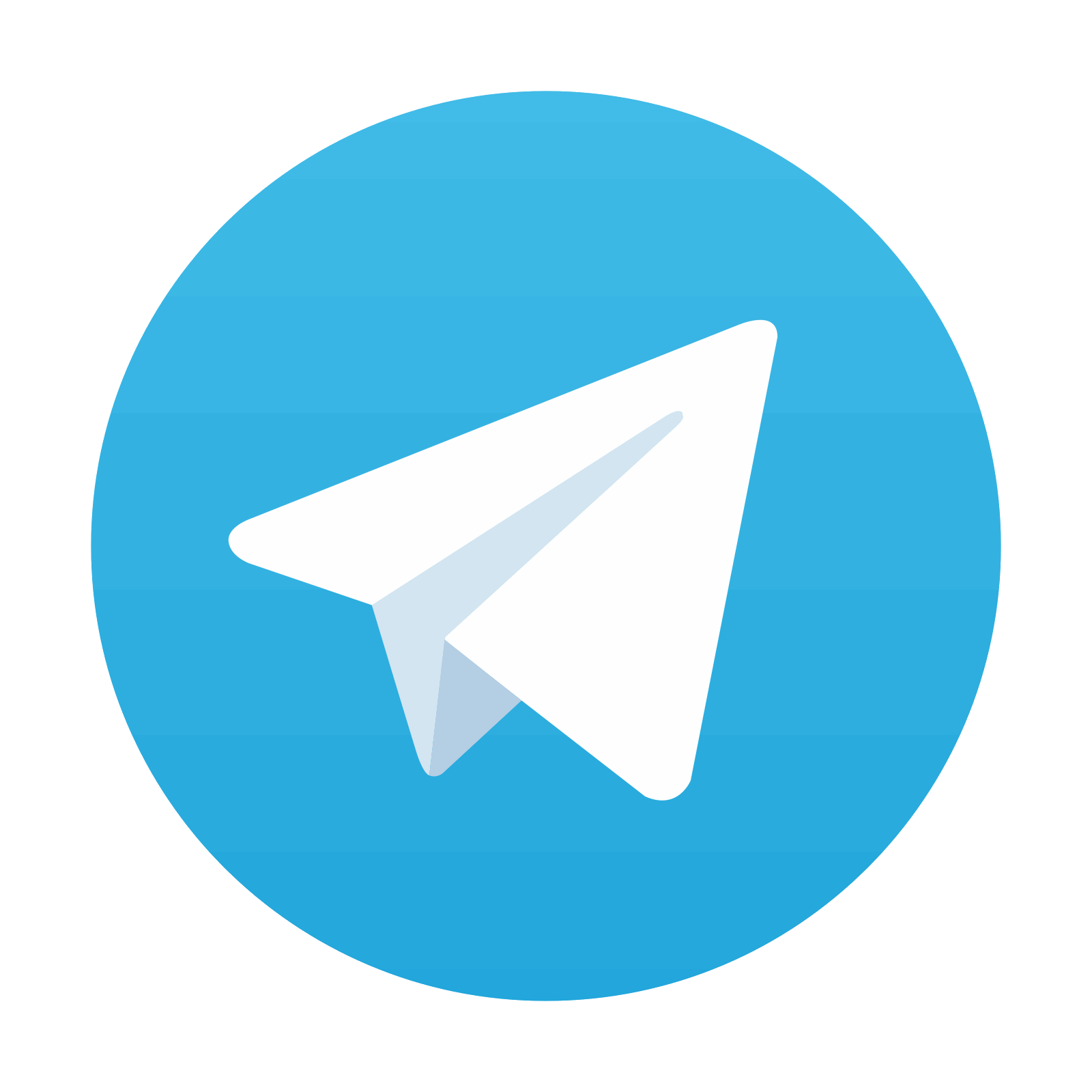
Stay updated, free articles. Join our Telegram channel

Full access? Get Clinical Tree
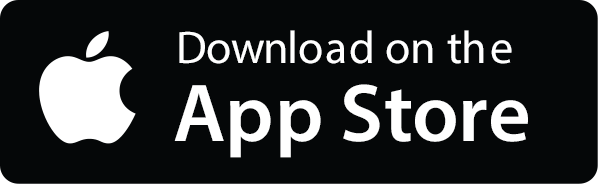
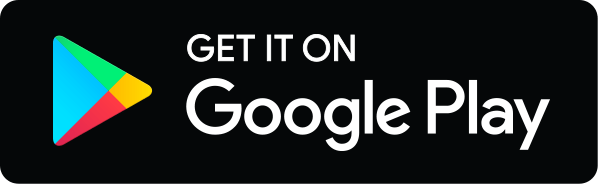