Molecular Genetics of Liver Diseases
Rondell P. Graham, MBBS
23.1 INTRODUCTION
Molecular techniques are increasingly used in the characterization of diseases and specimens in clinical practice and research. These scientific advances have advanced the understanding of liver diseases. This chapter will provide an overview of the most commonly used molecular techniques for diagnostic and theranostic purposes. Thereafter, this chapter will review the clinically relevant molecular discoveries to date in common primary liver tumors. The liver is a common site for metastatic disease. As such, this chapter will also review molecular targets for a select group of metastases to the liver. Finally, the chapter will review the molecular defects in a collection of hereditary liver diseases, which may lead to early cirrhosis.
23.2 MOLECULAR TECHNIQUES
The most commonly employed modality for germline genetic testing is Sanger sequencing. This method works by dideoxy termination and allows for amplification and sequencing of part of a gene or an entire single gene at a time. This method has been the main workhouse in clinical laboratories for some time, but the advent of next-generation sequencing has changed this. Next-generation sequencing employs a massively parallel sequencing approach, allowing for the sequencing of several genes or the entire exome (DNA) or transcriptome (RNA) at a given time. Whole exome sequencing has been shown to be a cost-effective and powerful approach for germline molecular diagnostics in several studies and is now in routine use in large academic centers.1, 2, 3 Smaller sequencing panels that evaluate genes known to be involved in specific disorders have also been created for more focused testing, as exemplified by a recent report on Wilson disease.4
Targeted genotyping assays are used for diseases that result from one or two mutations in a single gene, for example, in HFE-related hereditary hemochromatosis and α-1-antitrypsin deficiency. These targeted assays may be based on a number of different platforms, but they all operate on the same principles as sequencing. It is important to note that targeted assays will only detect the alteration(s) for which they were designed.
Other approaches are also being developed. As one example, mass spectrometry-based testing can identify the S and Z alleles of
SERPINA1 based on the affect these alleles have on the mass-to-charge ratio of the peptides of the SERPINA1 (α-1-antitrypsin) protein.5 The main advantage of this assay is that it is significantly less expensive than targeted genotyping assays. However, it has the same limitations as other targeted assays for α-1-antitrypsin deficiency in that it cannot detect mutations for which it was not specifically designed.5
SERPINA1 based on the affect these alleles have on the mass-to-charge ratio of the peptides of the SERPINA1 (α-1-antitrypsin) protein.5 The main advantage of this assay is that it is significantly less expensive than targeted genotyping assays. However, it has the same limitations as other targeted assays for α-1-antitrypsin deficiency in that it cannot detect mutations for which it was not specifically designed.5
For somatic tumor testing, sequencing assays such as those described above play a very important role. Given the improved analytic and clinical sensitivity of next-generation sequencing and its reduced cost, whole exome or large gene panel sequencing assays are being utilized with increasing frequency.6, 7, 8, 9, 10 Also, the characterization of an increasing number of tumors as translocation-associated tumors has led to the use of RNA sequencing clinically to support diagnoses.11, 12, 13, 14 Frozen tissue provides a better quality and quantity of nucleic acids for somatic testing than formalin-fixed paraffin-embedded tissue.15, 16, 17, 18 However, most pathology practices do not have the resources for routine collection of frozen tissue for clinical care. Fortunately, numerous studies have shown successful global transcriptome (RNA) and multigene DNA sequencing studies from formalin-fixed paraffin-embedded tissue.6, 7, 8, 9,19, 20, 21, 22, 23, 24, 25 Indeed, clinically validated assays for large gene panels from formalin-fixed paraffin-embedded tissue are commercially available and in use in clinical care. Despite this broad success, individual cases may not yield sufficient quantity or quality nucleic acids leading to noninformative test results.
The most commonly identified alterations from DNA sequencing are point mutations and small insertions and deletions. As mentioned above, RNA sequencing can detect translocation events which produce a novel fusion transcript, in addition to detecting sequence alterations. It is important to point out that RNA sequencing cannot directly detect translocations (i.e., genomic rearrangements) that lead to a promoter switch or a change in regulatory region/elements of a gene, but do not create a fusion transcript. Instead, these latter changes are best detected in clinical practice by fluorescent in situ hybridization (FISH). Mate pair sequencing and other methods can also be used for detecting such large structural changes, but these latter methods are not routinely used in clinical practice.
Several more targeted test methods are still very commonly used. Reverse transcriptase polymerase chain reaction (PCR) is widely used for the detection of specific fusion transcripts that characterize a variety of different tumor types. This technique includes extraction of total RNA from tissue followed by reverse transcription to cDNA. Primers for specific fusion event(s) are then used to amplify the fusion transcript, if it is present. Next, the results of the reaction are detected by any of a number of techniques. Classically, gel electrophoresis is used to detect the amplification products (Fig. 23.1).
PCR-based detection of nucleic acids of microorganisms in formalin-fixed paraffin-embedded tissue is being increasingly employed.26 These assays use a variety of detection systems including Taqman or FRET probes, depending on whether or not melt curve analysis is desired.26 As one example, the molecular detection of viral hepatitis E RNA has contributed to considerable improvement in the clinical care of immunocompromised solid organ transplant recipients.27,28 Briefly, it has recently been recognized that viral hepatitis E can cause chronic liver disease in the immunosuppressed patient, manifesting with a nonspecific histologic picture of chronic hepatitis.27, 28, 29 Chronic viral hepatitis E can lead to allograft loss, and serologic tests for hepatitis E are difficult to interpret. Detection of hepatitis E RNA can definitively make the diagnosis of chronic hepatitis E infection of the allograft, which can be cured with antiviral therapy.30 This example illustrates the power of molecular techniques in clinical scenarios where serology and morphology do not allow for specific diagnosis of an infectious organism involving the liver.
FISH is another modality often used for molecular cytogenetic characterization of liver neoplasms. FISH can be used to detect translocations or to identify copy number abnormalities. For the former, different color probes are used that are specific to the genes or regions involved in the genomic change. The presence of the translocation gives rise to an altered signal pattern with probes being brought together (fusion
probe set; Fig. 23.2) or probes being separated (break apart probe set; Fig. 23.3). For copy number changes different color probes to the gene/locus of interest and the centromere of the chromosome in question are used to enumerate the number of copies of the gene and the chromosome (Fig. 23.4). Multiple probes can be combined to allow for simultaneous enumeration of several loci or chromosomes. This combination of multiple enumeration probes is used in the biliary FISH assay used to evaluate bile duct brushings for neoplasia, which will be discussed later in this chapter.
probe set; Fig. 23.2) or probes being separated (break apart probe set; Fig. 23.3). For copy number changes different color probes to the gene/locus of interest and the centromere of the chromosome in question are used to enumerate the number of copies of the gene and the chromosome (Fig. 23.4). Multiple probes can be combined to allow for simultaneous enumeration of several loci or chromosomes. This combination of multiple enumeration probes is used in the biliary FISH assay used to evaluate bile duct brushings for neoplasia, which will be discussed later in this chapter.
Array comparative genomic hybridization (array CGH) provides a pan-genomic view of the copy number state of a large number of genomic loci, the autosomes, and the X chromosome. The density of the array determines the resolution of the platform. A platform for formalin-fixed paraffin-embedded tissue is commercially available. Despite the potential of array CGH to simultaneously evaluate for a large number of copy number alterations, this technology is not currently in routine use for the evaluation of liver neoplasms.
23.3 TUMORS
Hepatocellular carcinoma
Hepatocellular carcinoma is the most common primary hepatic malignancy. Several large-scale exome and transcriptome sequencing studies have been reported in the literature, which have repeatedly yielded a pattern of low-frequency mutations for a number of genetic drivers.31, 32, 33, 34 The most common alterations are in TP53, CTNNB1 (beta catenin gene), CDKN2A, and the promoter of TERT. TERT promoter mutations
are present in approximately 50% of hepatocellular carcinoma, with β-catenin and TP53 mutations in approximately 25% and 20% of hepatocellular carcinoma respectively.31,32,34 CDKN2A mutations are present in approximately 10% of hepatocellular carcinoma. All other mutations are identified at a much lower frequency. The patterns of mutations present across large cohorts of hepatocellular carcinoma have been correlated to various etiologic agents.33,35,36 One study revealed a correlation between alcohol and β-catenin mutations and viral hepatitis B and TP53 mutations.36 In another study, TERT promoter mutations were significantly correlated with Wnt pathway activation in nonviral hepatitis B cases.34 Finally, ARID2 mutations, which are uncommon in hepatocellular carcinoma overall (approximately 6%), were found to be enriched in individuals with underlying chronic viral hepatitis C.33 Currently, none of these mutations represent targets for therapy and a role in routine diagnostics has not yet been shown.
are present in approximately 50% of hepatocellular carcinoma, with β-catenin and TP53 mutations in approximately 25% and 20% of hepatocellular carcinoma respectively.31,32,34 CDKN2A mutations are present in approximately 10% of hepatocellular carcinoma. All other mutations are identified at a much lower frequency. The patterns of mutations present across large cohorts of hepatocellular carcinoma have been correlated to various etiologic agents.33,35,36 One study revealed a correlation between alcohol and β-catenin mutations and viral hepatitis B and TP53 mutations.36 In another study, TERT promoter mutations were significantly correlated with Wnt pathway activation in nonviral hepatitis B cases.34 Finally, ARID2 mutations, which are uncommon in hepatocellular carcinoma overall (approximately 6%), were found to be enriched in individuals with underlying chronic viral hepatitis C.33 Currently, none of these mutations represent targets for therapy and a role in routine diagnostics has not yet been shown.
Fibrolamellar carcinoma
Fibrolamellar carcinoma is currently classified as a variant of hepatocellular carcinoma. However, fibrolamellar carcinoma shows several distinctive features: (1) the tumors have a predilection for younger patients (>85% of patients are under 35 years at diagnosis)37; (2) the tumors arise in livers without chronic liver disease38; (3) the tumor shows characteristic histologic features (almost all fibrolamellar carcinomas look the same)38; (4) the neoplastic cells typically display coexpression of cytokeratin 7 and CD6839; and (5) the tumors have a recurrent fusion gene that is found in fibrolamellar carcinoma but not in conventional hepatocellular carcinoma.40,41
Almost all fibrolamellar carcinomas are characterized genetically by the recurrent DNAJB1-PRKACA fusion gene, owing to an intrachromosomal deletion at chromosome 19p13.40 This fusion gene is the oncogenic driver of the fibrolamellar carcinomas.40 Detection of this fusion transcript is specific for the diagnosis of fibrolamellar carcinoma in the context of primary hepatic neoplasms.41 A commercially available FISH test to detect PRKACA rearrangement has been clinically validated and can also be used to confirm the diagnosis of fibrolamellar carcinoma. The FISH test displays a sensitivity of 99% and specificity of 100% in the context of primary hepatocellular neoplasms and is informative even when there are as few as 50 tumor nuclei available for analysis.42 Very rare cases of fibrolamellar carcinoma are negative for the DNAJB1-PRKACA translocation and instead reveal PRKAR1A biallelic inactivation in the context of the Carney complex.42 Biallelic inactivation of PRKAR1A provides an alternate means for activation of the Protein kinase A pathway, which appears to be a major driver of tumorigenesis in fibrolamellar carcinoma. No molecularly targeted treatment has yet been developed for fibrolamellar carcinoma.
Hepatocellular adenoma
Hepatocellular adenomas are molecularly heterogeneous tumors, reflecting dysregulation of a number of different pathways.43 The epidemiology, morphology, and immunohistochemical stains used in the classification of these tumors are discussed in detail in Chapter 27. However, the salient molecular features will be described here. The first type of hepatocellular adenomas are the HNF1-α inactivated adenomas.43, 44, 45 These are characterized by loss of LFABP expression and tumors can occur sporadically (most cases) or occur in patients bearing germline HNF1-α mutations. HNF1-α functions as a tumor suppressor, and both copies of the gene require inactivation for tumorigenesis. Germline HNF1-α mutations are also associated with maturity onset diabetes of the young type 3.
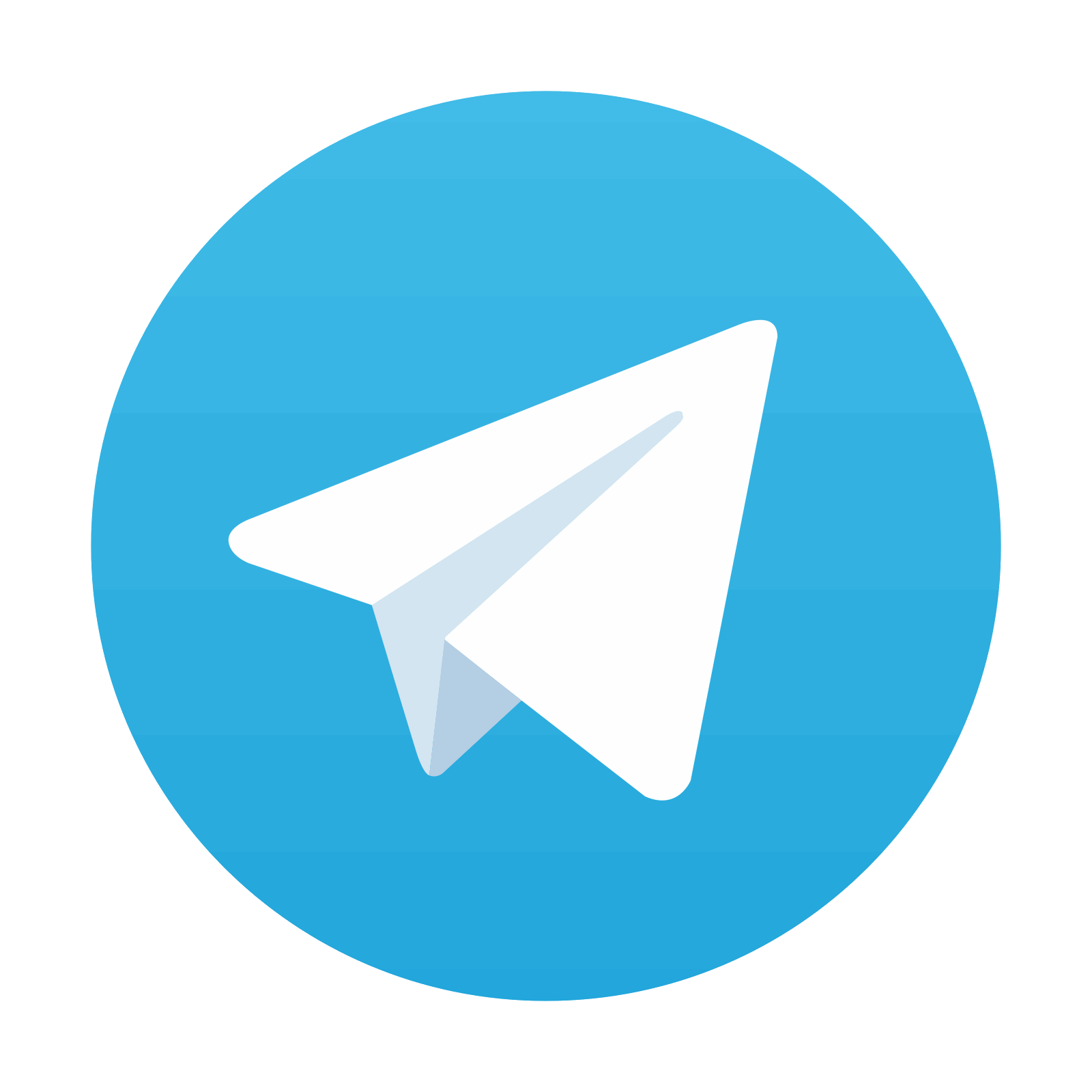
Stay updated, free articles. Join our Telegram channel

Full access? Get Clinical Tree
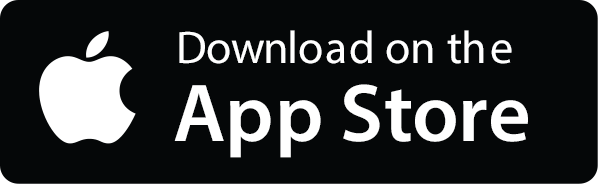
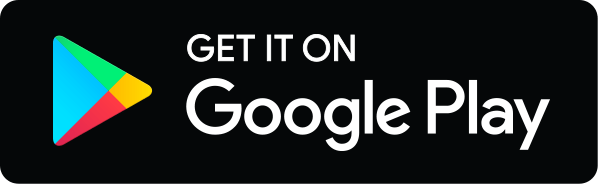