Fig. 7.1
The principal participants in normal bone remodelling include three cell types (bone marrow stromal cells, osteoblasts [OB], and osteoclasts [OC]) and three proteins (receptor activator of nuclear factor kappa B [RANK], receptor activator of nuclear factor kappa B ligand [RANK-L], and osteoprotegerin [OPG]). OCs are derived from myeloid precursors and promote bone resorption. RANK, a protein complex that controls the transcription of DNA, is activated by RANK-L, a member of the TNF superfamily and a key osteoclastogenic cytokine which is produced mainly by bone marrow stromal cells and OBs. When RANK-L binds its receptor RANK on OC progenitor cells, this promotes osteoclastogenesis, OC proliferation, OC activation, and consequently, bone resorption. RANK-L activity is inhibited by OPG, a soluble decoy receptor for RANK-L. Activated T cells and numerous pro-inflammatory cytokines (interleukins 1β and 6 [IL-1β, IL-6]I, tumor necrosis factor-α [TNF-α]), and macrophage colony stimulating factor (M-CSF), all found in the neoplastic milieu, have been shown to promote RANK-L driven bone resorption, thereby perturbing the RANK/RANK-L/OPG balance
Bone metastases have been characterized predominantly as osteoblastic or osteoclastic. In actuality, the majority of metastatic bone lesions involves both OC and OB activity, but with a dysregulation in the normal balance between OB and OC activity. For example, although most patients with breast cancer have predominantly OC-mediated bone destruction, there is secondary OB bone formation in response to osteolysis [11]. Similarly, while metastatic prostate cancer is associated with predominant osteoblastic lesions, there is increased bone resorption at the sites of these osteoblastic lesions[12]. Uniquely, multiple myeloma (MM) can cause purely lytic bone lesions. Indeed, bone scans of patients with MM and severe osteolytic lesions are normal in up to half of all patients [13]. RANK-L and macrophage inhibitory protein 1α, potent inducers of OC formation, appear to be the key mediators in bone destruction in these MM patients [14–16].
While a number of humeral factors present in the neoplastic milieu have been identified to promote bone resorption, PTH-rp accounts for an overwhelming majority of cases. PTH-rp production has been reported to be the pathogenetic mechanism in up to 88 % of cases of HM. PTH-rp was found in 92 % of breast cancer patients with bone metastases [17]. Local osteolysis and vitamin D production together accounted for the remaining 12 % of cases [18]. When vitamin D production is reported as the cause of HCM, it has been most often associated with lymphomas and MM.
PTH-rp is a protein member of the parathyroid hormone family and has biological roles in development of mammary glands, lactation, endochondral bone formation, and islet function. There is homology between PTH and PTH-rp at the amino terminal end, but the divergence in the remainder of the molecule accounts for their immunologic distinctiveness. There is no cross reactivity between the assays for PTH and PTH-rp. Like PTH, PTH-rp can stimulate OC activity; increase renal calcium absorption in the loop of Henle and distal convoluted tubule, and stimulate vitamin 1,25(OH)2 production. Interestingly, there is a higher incidence of cancer in patients with primary hyperparathyroidism (HPT), and of primary HPT in patients with cancer. Therefore, it is recommended that both the PTH and PTH-rp be checked in cancer patients since primary HPT portends a better prognosis and requires specific therapy [19, 20].
Hypercalcemia can affect the neuropsychiatric, cardiac, gastrointestinal, and renal systems. From a renal standpoint, the relevant clinical manifestations include nausea, lethargy, acute kidney injury, polyuria, and thirst. Polyuria develops because the high serum calcium levels activate calcium sensing receptors (CaSR), which in turn, results in decreased transport of NaCl in the loop of Henle, and consequently, a decrease in the countercurrent mechanism and a decline in renal concentrating ability. Moreover, activation of the CaSR blunts the response to antidiuretic hormone (ADH) in the collecting duct [21]. The resulting polyuria induces volume contraction and stimulates thirst. These patients often cannot hydrate themselves adequately due to hypercalcemia induced nausea, vomiting, and lethargy. Hypercalcemia induced vasoconstriction of the renal blood vessels, coupled with volume contraction, can both contribute to acute kidney injury in these patients.
The primary goals in the management of hypercalcemia are to (1) increase urinary excretion of calcium and (2) inhibit OC bone resorption. To this end, in the absence of edema, aggressive volume resuscitation with isotonic NS to promote a urine output of 100–150 cc/h is recommended. These patients are often significantly dehydrated and may require several liters of isotonic saline to restore their intravascular volume deficit. Once the patient is adequately volume replete, furosemide can be used to promote a calciuresis. It is recommended that furosemide should not be given prior to volume resuscitation, since the diuretic can further compromise the patient’s hemodynamic status. Calcitonin can begin to lower serum calcium within 4–6 h of administration, with a maximum effect of 1–2 mg/dL (0.3–0.5 mmol/L) [22, 23]. It does so by inhibiting bone resorption and OC maturation, and by increasing urinary calcium excretion. Unfortunately, tachyphylaxis due to downregulation of it receptors occurs after repeated dosing, thus the medication loses efficacy after 48 h [24]. In patients who are volume overloaded or anuric, dialysis with a low calcium dialysate (2.5 mEq/L) may be necessary to manage HM.
Since receiving the FDA approval for treatment of osteolytic bone metastases in the 1990s, bisphosphonates (BP) have become a cornerstone for management of HM. Pamidronate and zoledronate currently have FDA approval for the management of HM. Ibandronate is available but does not have this FDA indication. BPs inhibit OC function via both intracellular and extracellular mechanisms. The BP molecule is structurally similar to native pyrophosphate molecules that normally adhere to hydroxyapatite crystal-binding sites on the bone surface, especially in areas undergoing active resorption. The BP molecules reduce osteoclast activity by preventing the OCs ability to adhere to the bone surface; to form the ruffled border; and to produce the proteins necessary for continued bone resorption [25–27]. At the intracellular level, BPs inhibits farnesyl diphosphate synthase in the mevalonate pathway, resulting in decreased OC progenitor development and recruitment by promoting OC apoptosis [28]. Since the onset of action of BP is 2–4 days, it is recommended that they be administered at the time that HM is recognized. Their nadir effect is evident at days 4–7 [1].
The farnesyl diphosphate synthase pathway is also present in human renal proximal tubular cell lines, and this may in part explain the acute tubular necrosis (ATN) and Fanconi’s syndrome that has been reported following exposure to BPs. Other well known renal complications of this class of drugs include collapsing focal segmental glomerular sclerosis (FSGS) and minimal change disease (MCD). Collapsing FSGS has been predominantly described with the use of pamidronate, and with few exceptions, most of these patients progressed to end stage renal disease requiring dialysis [29]. Avascular necrosis (AVN) of the jaw, and at other areas of high occlusal force, due to over suppression of OC bone turnover, has also been reported following BP exposure. Collapsing FSGS and AVN has been more frequently reported in cases when the BP was administered in excess of the recommended dose. Consequently, current recommendations are that BPs should not be dosed more frequently than every 3 weeks. Even though pamidronate has been associated with nephrotic syndrome and collapsing FSGS, it appears to be relatively safe when appropriately dosed in patients with chronic kidney disease [30]. Zolendronate is more often associated with renal tubular toxicity [29].
For pamidronate, the current dosing guidelines for patients with a creatinine clearance (CrCl) < 30 cc/min is the usual 90 mg dose to be given over 4–6 h. Zoledronate is contraindicated in patients with a CrCl < 30 cc/min. For those patients with a CrCl between 30–60 cc/min, dose of zoledronate should be reduced, with no dose adjustment for pamidronate. To date, intravenous ibandronate has not been reported to cause renal toxicity [31]. However, as stated previously, the drug does not yet have an FDA approval for treating HM.
Following BP therapy, 60–90 % of patients achieve normocalcemia for a 1–3 week period following drug administration. Given the potentially devastating clinical consequences associated with exceeding the recommended dosing guidelines for BPs, managing those patients who have HM that is resistant to BP therapy can be challenging. For those patients who present with hypercalcemia within that 3 week period following the last dose of BP. HM that is resistant to BP may be due to inadequate bone resorption [32–34]. Also PTH-rp augments renal calcium absorption, and this may explain recurrent hypercalcemia shortly after BP therapy [35].
Researchers have investigated therapeutic strategies for the management of HM that is resistant to BP therapy. The most promising agent is denosumab, a fully humanized monoclonal antibody against RANK-L. Urinary and serum N telopeptide levels start to decrease within 1 day of exposure to denosumab and this effect lasts for up to 64 days. The onset and duration of these indices following pamidronate dosing are 3 and 28 days, respectively [36]. Currently, the recommended dose of denosumab is 120 mg SQ every 4 weeks. Importantly, this drug does not yet have an FDA approval for the treatment of HM. It does have an FDA approval for prevention of skeletal related events in bone metastasis from solid tumors. The agent has no known nephrotoxic effects but has been reported in association with AVN of the jaw [37] and can cause severe hypocalcemia [38]. Presently, there is an ongoing clinical trial on the treatment of HM in subjects with elevated serum calcium despite recent treatment with IV BPs (ClinicalTrials.gov Identifier:NCT00896454). Another agent which has been used to treat HCM and those with parathyroid cancer is cinacalcet. This agent binds to calcium sensitive receptors (CaSR) on tumor cells and downregulates PTH synthesis and therefore decreases serum calcium [39]. This drug is not FDA approved for treating HM.
Case #1 Follow Up and Discussion:
The patient was started on IV hydration with NS. Once well hydrated over 24 h, the patient was given furosemide to promote calcium loss in the urine. This will acutely correct the hypercalcemia. This patient has HM that is resistant to BP therapy. Since a BP was given less than 2 weeks ago, it is not advisable to retreat with BP at this time. The patient does have bone metastasis, and therefore, can be given denosumab 120 mg SQ every 4 weeks for the indication to decrease skeletal related events. Note that denosumab does not yet have an FDA approval for managing BP resistant HM.
Hyponatremia
Case #2
A 25-year-old male with a nonseminomatous germ cell tumor who completed his first cycle of cisplatin and ifosfamide 5 days ago was brought to the ER for nausea, vomiting, and confusion. Significant vital signs reported upon presentation are a blood pressure of 90/54 mm/Hg and a heart rate of 140 beats/min. He responded appropriately to his name but could not answer questions appropriately. Initial laboratory tests show a sodium concentration of 109 mEq/L, creatinine 3 mg/dl (was 1.0 mg/dl at baseline), serum osmolarity 235 mOsm, urine osmolarity 650 mOsm, urine sodium 174 mEq/L, normal TSH, and a slightly elevated serum cortisol value. At the time of examination, he had received 2 L of 0.9 % NS. His blood pressure was 95/50, heart rate 120, and sodium concentration was stable at 109 meq/L.
What is the cause of hyponatremia in this patient and what is the appropriate treatment?
a.
Syndrome of inappropriate antidiuretic hormone (SIADH)
b.
Hypovolemic hyponatremia from gastrointestinal losses
c.
Renal salt wasting syndrome related to cisplatin
d.
Adrenal crisis
Hyponatremia has been observed in up to 46 % of hospitalized cancer patients, and when present, is associated with a poor prognosis compared with euvolemic patients, regardless of tumor type [40–46]. The reported incidence varies greatly, and is affected by the cancer type and the cutoff point for serum used to define hyponatremia. Hyponatremia has been reported most frequently with small cell lung cancer (SCLC) [47]. The syndrome of inappropriate antidiuretic hormone release (SIADH) is the most common cause of hyponatremia in cancer patients, with higher rates among those with SCLC than with other malignancies [48, 49]. Ectopic antidiuretic hormone (ADH) can be produced by tumors, chemotherapeutic agents, pain and nausea, and by other medications commonly prescribed in this population. Even though SIADH is a common cause of hyponatremia in cancer patients, these patients are also at increased risk for hypovolemic hyponatremia from volume contraction due to vomiting, diarrhea, and salt-wasting nephropathy.
There are two important points to keep in mind when approaching disorders of serum sodium: (1) Disorders of serum sodium are essentially disorders of serum osmolarity, and (2) disorders of serum sodium are disorders of relative concentrations of salt and water since serum sodium is expressed in mEq/L. So, even in the patient who has hypovolemic hyponatremia, there is an excess of total body water (TBW) relative to total body sodium .
To elaborate on the first point, disorders of serum sodium can be approached as disorders of serum osmolarity since serum osmolality = 2 Na + Glucose/8 + BUN/2.8. Therefore, in a euglycemic patient, serum sodium essentially equals serum osmolality. Serum osmolality is normally tightly maintained between 280–290 mOsm/L and within 1–2 % in a particular individual. Osmoreceptors located in the anterior hypothalamus detect serum osmolality, and, under physiologic conditions, an increase in serum osmolarity causes release of ADH from the posterior pituitary. In the kidneys, ADH binds to V2 receptors on the basolateral membrane of collecting ducts cells to promote insertion of aquaporin-2 channels to the apical membrane. Increased free water permeability of the apical membrane promotes free water absorption, and consequently, reduces serum osmolality closer to the normal range. ADH also stimulates thirst at higher values of serum osmolality. In the hyperosmolar patient, the end effect of ADH release is increased free water retention. The other physiologic stimulus for ADH release is a decline in plasma volume of > 7–9 % [50, 51]. These systems are bimodal and importantly, in the absence of an elevated serum osmolality or a significant decrement in plasma volume, ADH should not be released. Therefore, to make the diagnosis of SIADH, the patient should be euvolemic and have a normal serum osmolality .
An algorithm for evaluating these patients is proposed in Fig. 7.2.The first step in evaluating the patient with hyponatremia is to measure the serum osmolality. Hyperosmolar hyponatremia in cancer patients can occur in the setting of procedures like hysteroscopy or transurethral resection of the prostate. During these procedures, large volumes of nonconductive flushing solutions containing glycine are used to create a surgical field within the body cavity. The large volumes of these solutions create elevated pressures within the body cavity. This allows osmotically active particles like glycine to translocate into the venous circulation. Osmotically active particles in the extracellular space cause movement of water from the intracellular to the extracellular space to equalize the osmotic gradient across the cell membrane. As a result, the serum sodium decreases due to dilution. Hyponatremia can occur via the same mechanism when intravenous immune globulin is given in a maltose or sucrose solution, or when patients are hyperglycemic or given mannitol.


Fig. 7.2
Algorithm for the evaluation of hyponatremia
A normal serum osmolality in a hyponatremic patient signifies that the patient has pseudohyponatremia. As the moniker suggests, these patients have a normal serum osmolality and normal serum sodium, but, due to the presence of excessive amounts of lipids or paraproteins in circulation, there is a reduction in the fraction of serum that is water and an artificially low serum sodium concentration is measured. With the newer ion specific electrode method, this miscalculation should not be an issue. It is important to identify this category of patients since they require no further therapy for hyponatremia .
After excluding the patient with hyperosmolar hyponatremia and pseudohyponatremia, the remaining patients fall into the category of hypoosmolar hyponatremia. Since this group lacks an osmolar stimulus for ADH release, the only physiologic stimulus for ADH release would be a significant decrement in plasma volume. Therefore, determining the volume status in these patients, as well as measuring the urine osmolality and urine sodium, will help to identify the etiology of the dysnatremia, as well as provide information on the appropriate treatment for this group.
Assessing the volume status in cancer patients can be challenging. Edematous patients may actually have intravascular volume depletion if the edema is the result of a pelvic mass compressing the lymphatic system; if it is due to a deep venous thrombosis or to IVC compression; or if the patient has a capillary leak syndrome from the malignancy itself. Patients with liver metastasis as a cause of hepatorenal syndrome can also have significant ascites and edema on examination, but have significant intravascular volume depletion due to vasodilation within the splanchnic circulation. Resting tachycardia can be a misleading sign of volume contraction since these patients experience pain, anxiety, and discomfort. Therefore, when feasible, checking orthostatic blood pressures can provide valuable information on the volume status of these patients.
Since all hyponatremia cases are essentially disorders of relative concentrations of salt and water, with relatively more TBW than salt, knowing the volume status of the patient can help to clarify the pathogenesis of the electrolyte disorder. In the hypervolemic patient, total body salt and TBW are both increased. In the hypovolemic patient, both are decreased but there is relatively more TBW than sodium. In the euvolemic patient, TBW and sodium are fairly normal but these patients do have slightly more TBW than salt, but no appreciable edema .
As stated previously, the diagnosis of SIADH can only be made in a patient who has a normal serum osmolality and normal plasma volume. In patients with SCLC, the tumor itself is the source of inappropriate ADH release. Additional etiologies of inappropriate ADH release in cancer patients include pain, nausea, and chemotherapy. Chemotherapeutic agents can alter central ADH secretion and its effects in the renal tubules. Vincristine and vinblastine are directly toxic to the hypothalamic pituitary axis and disturb the normal osmotic regulation of ADH secretion [52, 53]. Cisplatin and cyclophosphamide both induce ADH release, and the latter also potentiates ADH effect in the kidney. Commonly prescribed medications like tricyclic antidepressants, serotonin reuptake inhibitors, monoamine oxidase inhibitors, and opioids stimulate ADH secretion. Nonsteroidal anti-inflammatory medications potentiate ADH effect in the renal tubules [53]. Conditions associated with cancer can also cause SIADH and include CNS or pulmonary diseases, such as subarachnoid hemorrhage, pneumonia, mechanical ventilation, and metastasis of the lungs and brain [51].
In the patient with hypovolemic hyponatremia, a review of the patient’s history and medication list will often reveal the source of their volume deficit. Volume losses from diarrhea, diuretics, or hyperglycemia are easily identified. A cause of hypovolemic hyponatremia that warrants special attention in cancer patients is saltwasting nephropathy. Cisplatin and ifosfamide directly injure renal tubule cells, thereby impairing renal sodium reabsorption [54, 55]. Cerebral salt wasting due to metastatic CNS disease, surgery, and trauma has also been described. Hyponatremia due to either nephrogenic or cerebral salt wasting may be difficult to distinguish from SIADH, since the urine osmolality and urine sodium can be elevated relative to the serum values. Importantly, patients with either type of salt wasting become volume contracted if their urinary losses of salt and water exceed the amount that they are able to ingest orally. Clinically, patients with salt wasting are hypotensive and/or orthostatic on examination, whereas the patients with SIADH appear euvolemic .
The clinical signs and symptoms of hyponatremia are in large part manifestations of increased intracerebral pressure due to brain edema. When the serum sodium and serum osmolality are lower than that within the brain cells, water shifts into the brain cells. Since the skull is a fixed cavity, it cannot expand to accommodate this increase in brain volume. Some clinical signs of increased intracranial pressure include nausea, confusion, vomiting, decline in mental status, ataxia, and seizures. If the brain volume markedly exceeds the skull volume, frank herniation of the brainstem occurs. There are adaptive mechanisms in place to mitigate brain edema in the setting of hyponatremia. When the serum sodium drops too rapidly relative to the ability of the brain to adapt to the change in osmolality, clinical signs and symptoms develop. This explains why one patient presents seizures and another can appear asymptomatic at equivalent serum sodium values.
The brain’s adaptive mechanisms are essentially aimed at decreasing its water content back to normal by extruding solute. In rat models, Na2+ and Cl−are extruded via the Na2+ and Cl− channels present in the cell membrane within 30 min of induction of hyponatremia. These electrolyte losses are maximal at around 3 h. After longer periods of persistent hyponatremia, organic osmolytes like glutamate, creatine, and taurine exit the brain cell [56]. These compensatory adaptations explain why rapid correction of chronic hyponatremia leads to rapid egress of water from the brain cells. In mild cases, dehydration of the brain tissue occurs, and in severe cases, osmotic demyelination can occur. The current recommendation is that the serum sodium should not be corrected more than 12 mEq/L within the first 24 h, and generally, at a rate of not more than 0.5 mEq/L/h [51] .
The severely symptomatic hyponatremic patient who are euvolemic or hypervolemic and are present with seizures, impaired mental status, or coma should be managed in consultation with a nephrologist and/or a critical care specialist. These patients require frequent neurological evaluations and monitoring of their serum sodium values while they receive 3 % NS. Importantly, the severely symptomatic patient with hyperosmolar hyponatremia should not receive 3 % NS. Administration of hypertonic solutions in this setting is contraindicated as they worsen the hyperosmolar condition in such patients. These patients may require urgent dialysis. Patients with significant hypovolemia should be treated with boluses of NS until a euvolemic state is achieved. Thereafter, the rate of correction of the serum sodium should not exceed 10–12 mEq/L in a 24 h period.
For patients with hypoosmolar hyponatremia who are minimally symptomatic, the appropriate treatment depends largely on their volume status and on the disease state that is responsible for their volume status. Hypervolemic hyponatremia due to iatrogenic volume overload is best managed by minimizing all intravenous solutions, with or without the addition of diuretics. Patients with congestive heart failure are best managed by minimizing all salt containing intravenous solutions (NS, Normosol, Lactated Ringers, and half NS) and with the use of diuretics. Furosemide is the diuretic of choice in the treatment of hyponatremia, since thiazide type diuretics can actually cause hyponatremia. The aquaretic agents, conivaptan and tolvaptan, have been shown to increase serum sodium by 6–8 mEq/L within a 48 h period without the hypokalemia and accompanying metabolic alkalosis that can result from treatment with diuretics [57].
The management of the asymptomatic patients with euvolemic hypoosmolar hyponatremia is a bit more multidimensional. If nausea, vomiting, and pain are stimulating ADH, then appropriate use of antiemetics and pain medications can diminish these nonphysiologic stimuli for ADH release. Any of the culprit medications that have been implicated as a cause of hyponatremia (see Fig. 7.3) should be discontinued if it is safe to do so. Additional interventions are all aimed at decreasing the relative concentrations of salt and TBW in the patient. Patients can be asked to restrict their fluid intake to 1–1.5 L of free water per day. Patient compliance with fluid restriction can be difficult since they are frequently told to “stay well hydrated” while on chemotherapy and because their pain medications and antidepressants can make them dipsogenic. Therefore, adjunctive pharmacologic therapy is frequently needed. In patients whose blood pressures can tolerate it, furosemide can be used to increase free water losses via the urine. Usual doses in patients with normal renal function are 10–20 mg per day.In euvolemic patients, NaCl tablets can be administered as well. V2 receptor antagonists are the new class of agents which can be used in the management of hypervolemic and euvolemic hyponatremia . V2 receptor antagonists block ADH-mediated insertion of aquaporin channels at the apical membrane of the collecting duct cells. Consequently, free water is lost in the urine. The currently available intravenous and oral formulations in the USA are conivaptan and tolvaptan, respectively. Although tolvaptan is administered orally, the package insert states that the medication needs to be started in the inpatient setting in order to check serial serum sodium values so that overly rapid correction of hyponatremia can be properly identified and managed. In a small study of cancer patients, tolvaptan was shown to be safe and to have superior efficacy in controlling hyponatremia when compared with standard therapy using fluid restriction, diuretics, and salt tablets. No patients overcorrected [58]. Any combination of fluid restriction, NaCl tablets, furosemide, and/or a V2 receptor antagonist can be used to achieve a normal serum sodium value in this group of patients.


Fig. 7.3
Causes of the syndrome of inappropriate antidiuretic hormone (SIADH)
Case #2 Follow Up and Discussion
Cisplatin and ifosfamide can both cause salt-wasting nephropathy. Correct answer is (c).
A diagnosis of SIADH is not tenable in the presence of hypotension and volume depletion. Patients with SIADH have elevated urine osmolarity in the absence of low urine sodium, and they are, by definition, normotensive (since hypotension would be a cause of appropriate ADH release). Moreover, when patients with SIADH are treated with 0.9 % NS, the serum sodium value typically decreases as they excrete the infused sodium in the NS (to be in sodium balance as their total body sodium is normal), but retain the water in the NS because of the inappropriate ADH, and as a result, the persistently open water channels. This patient would be best managed with an admission to the ICU. He can be given 0.9 %NS until he is no longer hypotensive, in order to avoid hemodynamic collapse. At that point, the serum sodium should be rechecked. Thereafter, the serum sodium can be corrected using 0.9 %, 2 % or 3 % NS to correct the serum Na, but not more than 10–12 mEq/L over a 24 h period. Patients like this may require oral sodium chloride tablets, midodrine, and fludrocortisone to maintain normal serum sodium and blood pressure until the salt wasting resolves.
Case #3
A 58-year-old female with metastatic breast cancer (brain, bone, liver) on paclitaxel is admitted for dizziness, palpitations and is found to be orthostatic on examination. She reports recent onset of urinary frequency and nocturia (up to 4 times per night). She drinks about 64 ounces of fluids per day as instructed by her oncologist and has no diarrhea or fevers. Her UA shows no evidence of infection; the serum glucose is normal and the urine specific gravity and osmolarity are 1.002 and 141 mOsml/kg, respectively.
Which of the following best clarifies the diagnosis?
a.
MRI of the abdomen/pelvis
b.
Water deprivation test
c.
MRI of the brain
d.
Administer DDAVP
Hypernatremia
Hypernatremia can be conceptualized in a manner similar to hyponatremia in that establishing the relative concentrations of salt and water in the patient and the volume status of the patient yields the correct diagnosis. Patients receiving exogenous sodium (hypertonic saline, IV NS, oral NaCl tablets, IV sodium bicarbonate) tend to be volume overloaded and/or hypertensive because they have an excess of salt relative to free water. Patients who have lost free water in excess of salt are volume depleted and/or hypotensive. Examples of such cases include free water losses from the kidney (diuretics), skin (high fevers, excessive sweating), respiratory tract (ventilated patients), and from the GI tract (diarrhea, vasoactive intestinal peptide producing tumors, or VIPomas). In all of these cases, if the patient cannot match or exceed the free water losses, the serum sodium concentration rises.
Because of ADH’s tight control of serum osmolarity within a very narrow range, when serum sodium increases (for example from 140–150 mEq/L) thirst is stimulated and individuals “drink themselves” back to a normal serum sodium. Therefore, hypernatremia develops when there is a defect in the synthesis or release of AVP from the pituitary (central diabetes insipidus, CDI) or unresponsiveness of the renal tubule to AVP (nephrogenic DI). The most common etiologies of CDI are neurosurgery, trauma, primary or metastatic tumors, and infiltrative diseases [59]. Leukemic infiltration of the pituitary stalk in patients with acute leukemias has also been reported to cause CDI [60, 61]. Most patients with CDI or NDI have a normal thirst mechanism and are also present with polydipsia. In the general adult population, the most common causes of NDI are chronic lithium use, hypokalemia and hypercalcemia [59]. In cancer patients, obstructive uropathy from masses at the bladder neck or ureter can also cause ADH unresponsiveness. Amyloid deposition along the basement membrane of medullary collecting ducts has been associated with unresponsiveness to AVP [62]. Chemotherapeutic agents and medications commonly used for the management of infectious complications following chemotherapy and bone marrow transplantation that have been associated with NDI include cidofovir, indinavir, tenofovir, foscarnet, amphotericin, ifosfamide, cyclophosphamide, methotrexate, and ofloxacin [63–67]. A water deprivation test can help to distinguish CDI from NDI and a detailed explanation of this test can be found elsewhere [59] .
Hypervolemic or euvolemic hypernatremia can be managed with discontinuing exogenous salt containing IVFs and by correcting the free water deficit orally with free water or intravenously with 5 % dextrose water. There are numerous online calculators to measure the water deficit, or the following equation can be used:
Free water deficit = 0.5* × body weight (kg) [140/plasma sodium −1]
*0.6 for lean males, 0.5 for females
To avoid brain edema, which can occur if the deficit is corrected too rapidly, only half of the calculated water deficit should be administered within the first 24 h. The serum sodium should not be corrected more than 12 mEq/L over the initial 24 h. When correcting hypovolemic hypernatremia, the best approach is to re-expand and the intravascular volume with 0.9 % or 0.45 % NS until the patient is no longer orthostatic. For patients with ongoing GI, renal, and insensible fluid losses, a portion of these losses should be added to the calculated deficit. Concomitant hypercalcemia and hypokalemia needs to be corrected as both conditions are associated with unresponsiveness of the renal tubules to AVP .
Case # 3 Follow Up and Discussion
The patient is orthostatic on examination so a water deprivation test is not appropriate to evaluate for diabetes insipidus. Administration of DDAVP in a patient with hypernatremia, a dilute urine and hypotension can help to distinguish if the patient has CDI or a NDI. Choice (d) is the most appropriate answer.
Case #4
A 40-year-old male with colon cancer on chemotherapy with irinotecan and cetuximab presents for routine follow up. His only complaints are fatigue and occasional but bothersome “twitchiness” of his eyes and some muscle spasms. Routine serum chemistries are drawn and the only abnormality is a magnesium level of 0.9 mEq/L. His medications include hydrochlorothiazide and omeprazole.
Which chemotherapy agent is the most likely culprit for the hypomagnesemia?
a.
Irinotecan
b.
Cetuximab
c.
Neither, it is the proton pump inhibitor
d.
Neither, it is the diuretic leading to hypomagnesemia
Hypomagnesemia
Among the general hospital population, hypomagnesemia has been observed in up to 15 % of patients [68, 69]. The incidence of hypomagnesemia appears to be higher among hospitalized cancer patients, especially critically ill cancer patients, with reported frequencies of 17 and 46 %, respectively [70–73]. Hypomagnesemia, when present, may be associated with poorer clinical outcomes. One study that looked at hypomagnesemic and normomagnesemic groups with comparable APACHE II scores found that the mortality rates of the hypomagnesemic medical ICU and non-ICU groups were approximately twice the rate of the normomagnesemic groups [74]. Of note, hypomagnesemia may actually be underreported since the serum magnesium level is not reported as part of the routine chemistry panel .
The incidence and severity of hypomagnesemia is particularly high among patients who receive treatment with cisplatin and monoclonal antibodies directed against the epithelial growth factor receptor (eEGFR) domain (cetuximab, panitumumab) . In a recent meta-analysis of the incidence of hypomagnesemia with cetuximab therapy, the incidence of all grade hypomagnesemia was 37 %. Panitumumab-induced hypomagnesemia was reported in 90 % of patients treated at one center [75]. Not infrequently, treatment with these agents has to be interrupted or stopped as a consequence of severe magnesium depletion. While patients receiving anti-EGFR therapy seem to respond to magnesium supplementation and maintain normal magnesium levels after the drug is stopped, a proportion of patients with ifosfamide- and cisplatin-related hypomagnesemia can have persistently low levels for years after cessation of drug treatment [76]. The incidence and severity of hypomagnesemia due to cisplatin and anti-EGFR therapy appear to be related to duration of exposure to these agents[77]. Other cancer drugs that can cause hypomagnesemia include cyclosporine, tacrolimus, pegylated liposomal doxorubicin, and interleukin-2. Other medications frequently given to cancer patients that can cause or potentiate hypomagnesemia include diuretics, aminoglycosides, amphotericin B, pentamidine, gentamicin, and proton pump inhibitors [69]. Proton pump inhibitors have been associated with hypomagnesemia (gastrointestinal loss of Mg) after prolonged use, usually more than a year [78–80].
Less than 2 % of the total body magnesium is present in the extracellular space, and only two third of this amount is unbound to albumin (free) and is active. The remainder of total body magnesium resides in bones and soft tissues, with the bones being the principal source of magnesium. The kidneys and the GI tract are the major organs of magnesium absorption and elimination from the body. Although magnesium depletion from GI losses can occur from upper or lower tract losses, lower tract secretions contain much larger magnesium content than upper tract secretions. (15 mEq/L in the lower tract versus 1 mEq/L in the upper tract) [81]. Thus, hypomagnesemia is more common with diarrhea, malabsorption, and short bowel resection compared with vomiting or nasogastric suction .
Magnesium elimination from the body occurs predominantly in the kidneys. In the setting of magnesium depletion, the kidneys fastidiously conserve magnesium, with the main site of magnesium reabsorption being the thick ascending limb of the loop of Henle. Magnesium handling in the kidney is slightly different than for other electrolytes in that the threshold for urinary excretion of magnesium is very close to the normal serum concentration of this electrolyte. Consequently, if a hypomagnesemic patient is receiving an IV bolus of magnesium and the serum magnesium rises abruptly, the kidneys quickly eliminate magnesium. For this reason, it is recommended that magnesium infusions should be given slowly to improve magnesium absorption and minimize renal elimination.
Hypokalemia and hypocalcemia can coexist with hypomagnesemia, so there is overlap between some of the clinical signs and symptoms of hypomagnesemia and deficiencies of these other electrolytes. Neuromuscular symptoms of magnesium deficiency include generalized weakness, tetany, seizures, delirium, and coma. Cardiac manifestations include EKG changes (widening of the QRS complex, flattening of the T wave) and ventricular and atrial arrhythmias .
The severity of the clinical manifestations of hypomagnesemia and the extent of the deficiency determines the appropriate dose and route for magnesium repletion. In the setting of ventricular arrhythmias or EKG abnormalities, IV magnesium sulfate 1–2 g can be given as a bolus followed by a slow infusion once hemodynamic stability is achieved. For severe asymptomatic hyponatremia (serum magnesium less than or equal to 1 mg/dl or 0.4 mmol/L, 4–8 g of magnesium sulfate can be infused slowly over 12–14 h. For less significant deficiencies (serum magnesium levels > 1.2 mg/dl or 0.5 mmol/L) oral magnesium can be used, preferably using a sustained release preparation .
Case #4 Follow Up and Discussion
Cetuximab is a monoclonal antibody directed against the epithelial growth factor receptor, and has been reported to cause hypomagnesemia in up to 37 % of patients. Concomitant use of proton pump inhibitors and diuretics may exacerbate the level of hypomagnesemia. When possible, these medications should be discontinued if the cetuximab needs to be continued. Given the presence of neuromuscular irritability, this patient will require intravenous therapy with magnesium sulfate. Magnesium is best absorbed when it is delivered by slow infusion. This patient can be given 6–8 g of magnesium sulfate over 6–8 h. In addition, oral magnesium therapy should be started. He should continue to receive additional doses of IV magnesium if he continues to have neuromuscular irritability. Often times, for patient on cetuximab, oral repletion does not adequately maintain normal serum magnesium levels, even after IV repletion. These patients may require IV magnesium repletion to be given on a regular basis (once or twice weekly) for the duration that they are on cetuximab, in order to maintain normal serum magnesium levels. The hypomagnesemia usually resolves several weeks after the treatment with cetuximab is completed. The correct answer is (b).
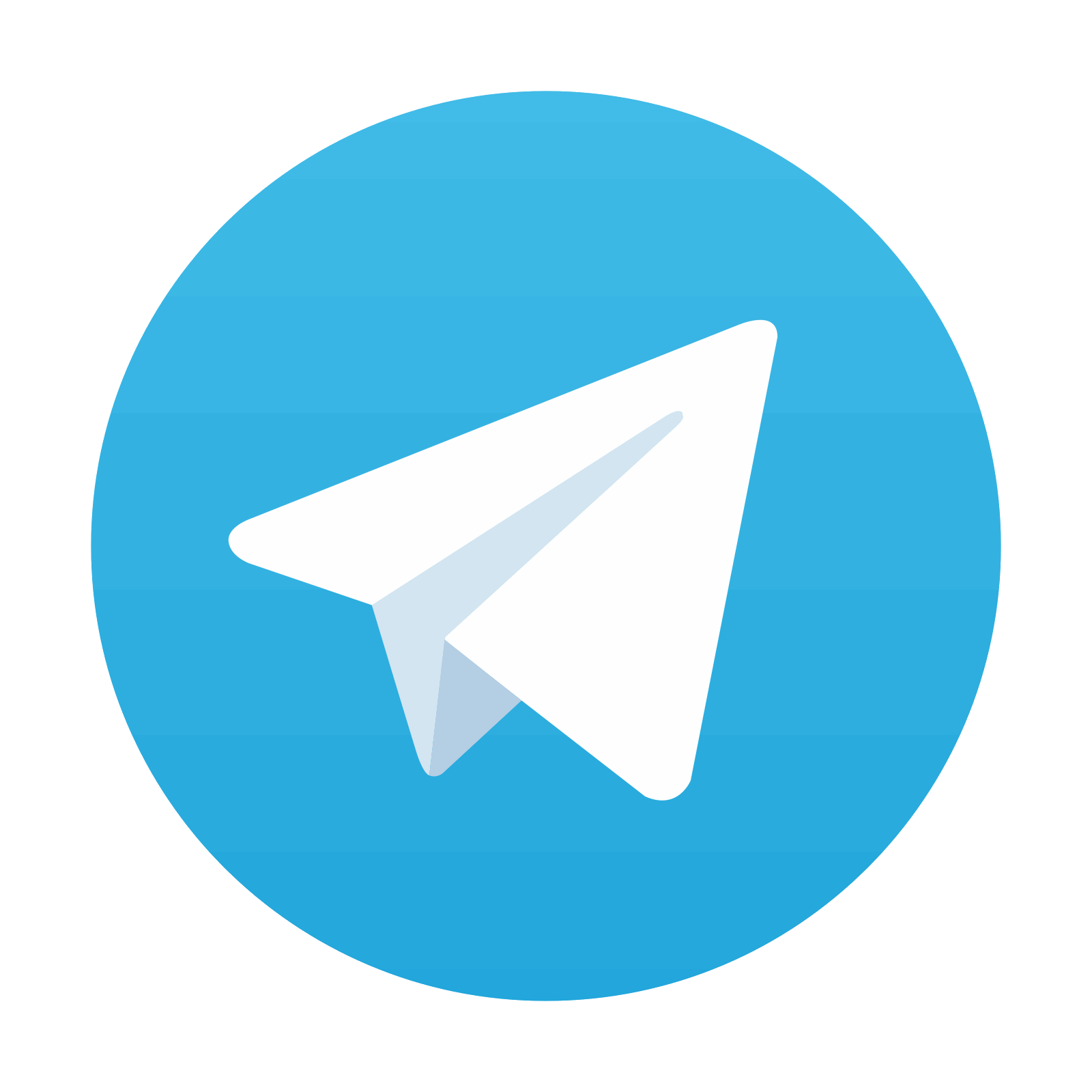
Stay updated, free articles. Join our Telegram channel

Full access? Get Clinical Tree
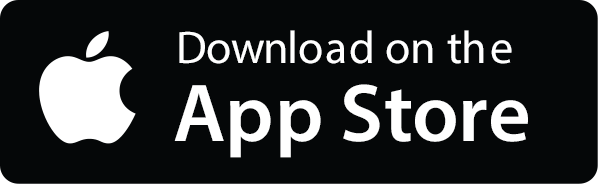
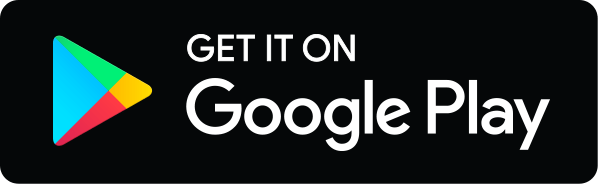