Class
Examples
Kidney toxicity
Antiangiogenic therapy
Bevacizumab, sunitinib, sorafenib, pazopanib, everolimus
Hypertension, proteinuria, TMA, AKI
EGFR inhibitors
Cetuximab, erlotinib, gefitinib, lapatinib, panitumumab
Hypomagnesemia, hypokalemia
Multifunctional TKIs
Imatinib, dasatinib, ponatinib, bosutinib
AKI, CKD, proteinuria, TMA
Case #1
A 58-year-old woman is referred to you for evaluation and management of hypertension. She was diagnosed with metastatic ovarian cancer 3 years ago and underwent de-bulking surgery followed by taxol and carboplatin. She recently progressed, and was started on bevacizumab monotherapy 4 months ago. She had a history of hypertension for 10 years, controlled with atenolol 100 mg per day. Since starting bevacizumab, she has experienced exacerbated hypertension, with values of 175/105 mm Hg, necessitating the addition of lisinopril 10 mg per day. However, she remains hypertensive (160/95 mm Hg) and is referred for further evaluation.
Although she complains of fatigue, she has tolerated the bevacizumab well, without headache, visual changes, or ankle swelling. Her exam is notable for a blood pressure (BP) of 158/97 mm Hg, normal fundoscopic exam, and no edema. Laboratories shows serum creatinine (1.0 mg/dL), hemoglobin (13.8 g/dL), and platelets (337,000 per/dL). Her haptoglobin is normal and there are no schistocytes on the smear. There is 1 + protein on the dipstick, and the sediment is bland. Urine protein to creatinine ratio is 0.6 g/g creatinine.
What is the mechanism of hypertension related to bevacizumab or other antivascular endothelial growth factor (VEGF) therapy (more than one possible answer)?
a.
Elevated renin production due to VEGF inhibition
b.
Reduced vasodilatory nitric oxide (NO) produced as a consequence of inhibition of the intrinsic tyrosine kinase activity of VEGFR2 that normally couples with endothelial nitric oxide synthase (eNOS)
c.
Microcapillary rarefaction
d.
Endothelin-1 (ET-1)-induced hypertension
Antiangiogenic Therapies
Antiangiogenic therapies target the VEGF molecule, its receptor, or downstream pathways. FDA-approved antiangiogenic agents include bevacizumab, a recombinant humanized monoclonal antibody that binds and sequesters the VEGF molecule, [4] and multi-targeted TKIs , small molecules that inhibit the vascular endothelial growth factor receptor (VEGFR) intracellular intrinsic kinase activity, such as sunitinib, sorafenib, axitinib, and pazopanib [5]. TKIs are not entirely specific for VEGFR2, they also have inhibitory activity against other receptor tyrosine kinases, such as the platelet-derived growth factor receptor or c-Kit. The use of these medications has since expanded to many different solid tumors, with numerous clinical trials of newer formulations of the medications underway. Antiangiogenic therapies are now the first-line therapies for cancers such as metastatic renal cell carcinoma, which accounts for 2.5 % of all new cancer diagnoses. With increased use of these medications and many highly potent formulations in development, there is an increasing clinical need to understand how to diagnose and manage VEGF inhibitor toxicities.
Hypertension Caused by Antiangiogenic Therapies
Hypertension is a very common toxicity of antiangiogenic therapy. Hypertension occurs in 19–24 % of patients receiving FDA-approved therapies [6, 7], but it can occur in up to 80 % of patients on the newer experimental forms of these medications [8]. Nearly all patients taking these drugs experience a rise in BP, even if not to hypertensive levels. With high-potency TKIs, BP rise can be rapid—within days. On the other hand, with biologic therapies such as bevacizumab, the rise can be slower, over weeks or months, as in the patient in Case #1. In one study examining sorafenib, among 54 patients initiating therapy, 93 % had a rise in BP by day 6, and most experienced a rise in BP over the first 24 h of therapy, as assessed by ambulatory blood pressure monitoring (ABPM) [9, 10]. BP typically falls when treatment is interrupted. We reported a very rapid rise in BP among women initiating cediranib therapy, a highpotency small-molecule VEGF-targeted therapy , with 67 % of patients developing hypertension over the first 3 days of therapy, and 87 % by the end of the study [8] .
The binding of VEGF to its receptor VEGFR2 activates the intrinsic tyrosine kinase activity of VEGFR2, which normally couples with eNOS and increases synthesis of vasodilatory NO [11]. Based on this, inhibition of VEGF signaling decreases NO bioavailability, causing vasoconstriction. Indeed, VEGF inhibition is associated with decreased urinary nitrite/nitrate excretion and decreased serum levels of NO metabolites in humans [12, 13], although no difference in flow-mediated dilation, a surrogate for NO bioavailability, has also been reported [13]. Treating mice with an anti-VEGFR2 antibody causes a rise in BP and reduces kidney eNOS and nNOS [14]. On the other hand, in a swine model of sunitinib-induced hypertension, NO bioavailability does not appear to contribute to sunitinib-induced hypertension [15]. Despite some conflicting evidence in the literature, most evidence to date does implicate increased peripheral vascular resistance in the pathophysiology of antiangiogenic therapy-induced hypertension .
Another important factor underlying antiangiogenic therapy-induced hypertension is ET-1, a potent vasoconstrictor. Sunitinib induces a rise in circulating levels of ET-1 in rodents, as well as in humans [16]. ET-1 levels rise rapidly after starting regorafenib, a TKI, and they normalize just as rapidly after discontinuation [17]. Pig models suggest that the rise in BP induced by antiangiogenic therapy can be prevented ET receptor antagonists [15, 18]. Since antiangiogenic therapies induce generalized endothelial dysfunction, which itself is a known trigger of ET-1 secretion [19], it is likely that this also plays a role in the underlying pathophysiology.
A third factor in antiangiogenic therapy-induced hypertension is microcapillary rarefaction. Although the data supporting a role for this are less strong, several observations suggest it may be playing a role. Rodents treated with VEGF inhibitors experience regression of tracheal capillary networks by up to 30 % at 21 days of therapy, and this process reverses with antiangiogenic therapy discontinuation [20]. In humans, capillary density reduction has been measured in patients taking either bevacizumab or TKIs, of the order of 10–20 % reduction [21, 22]. It is important to note, however, that increasing peripheral vascular resistance by 5 % requires rarefaction of 40 % of the microcapillary bed—more than what has been observed in humans [16, 23]. At the present time, more research is needed to better define the role of capillary rarefaction in antiangiogenic therapy-induced hypertension .
Other pathways may also be playing roles. A shift in the pressure–natriuresis curve, causing volume overload, has been reported by Facemire and colleagues [14]. Macrophage-derived VEGF-C has been proposed to regulate lymphangiogenesis and extracellular fluid buffering, and anti-VEGF therapies may interrupt this capacity [24]. The degree to which these pathways and others, such as prostaglandins or reactive oxygen species might contribute remains unclear [25]. Figure 5.1 summarizes these pathways .


Fig. 5.1
Multiple mechanisms by which VEGF blockade induces hypertension. VEGF signaling blockade inhibits NO production, enhances endothelin-1 secretion, and causes capillary rarefaction. All of these effects cause increased afterload and consequent increased blood pressure. In addition, VEGF blockade shifts the pressure–natriuresis curve, and decreases lymphangiogenesis and both of these effects contribute to volume overload and hypertension
Case #1 Follow Up and Discussion
Choices b, c, and d are correct. Reduced vasodilatory NO produced as a consequence of inhibition of the intrinsic tyrosine kinase activity of VEGFR2, which normally couples with eNOS, microcapillary rarefaction, and ET-1-related effects are important mechanisms. Renin is not a major described mechanism in these agents.
Case #2
A 78-year-old woman with renal cell carcinoma is referred to you for proteinuria. She has a history of hypertension controlled with hydrochlorothiazide and diabetes on metformin. She started sunitinib 11 months ago, and had no proteinuria by urinalysis at that time. She developed ankle edema 5 months ago, and a 24-h urine collection was performed that showed 7.2 g of proteinuria. Her hydrochlorothiazide dose was increased. Last month her edema worsened, and she was referred to you.
She complains of significant ankle swelling. Her exam is notable for a BP of 152/93 mm Hg and 2+ pitting edema to her mid-thighs bilaterally. Serum creatinine is 1.4 mg/dl and her urine protein to creatinine ratio is 10.9 g/g creatinine. Urinalysis shows 1+ hematuria with 3+ protein. What is the most likely mechanism of her proteinuria?
a.
Minimal change disease
b.
Membranous nephropathy
c.
Hyperfiltration-related focal and segmental glomerulosclerosis
d.
Thrombotic microangiopathy (TMA)
Proteinuria After Antiangiogenic Therapies
Proteinuria after antiangiogenic therapy is most commonly the consequence of renal TMA .
Case #2 Follow-Up and Discussion
Proteinuria on the administration of antiangiogenic therapy is most commonly the consequence of renal TMA. The clinical syndrome is characterized by hypertension, microangiopathic hemolysis, renal failure, sub-nephrotic proteinuria, and microscopic hematuria. Complement levels are typically normal. Haptoglobin levels may be low, and schistocytes can be present on smear, reflecting renal-limited microangiopathic hemolysis. On renal biopsy, endotheliosis is the most prominent feature, and endothelial injury is reflected by thickened basement membranes with double contours. Answer d is correct.
In one study of 1850 patients receiving bevacizumab, the incidence of new proteinuria ascribable to bevacizumab was about 5–10 % and the risk was dose-dependent (RR, 1.4 with low-dose bevacizumab; 95 % confidence interval, 1.1–1.7; RR, 2.2 with high dose; 95 % CI, 1.6–2.9) [6]. This proteinuria probably reflects renal TMA . In a larger meta-analysis (12,268 patients), the incidence of any grade proteinuria associated with bevacizumab was found to be 13.3 % (95 % confidence interval, 7.7–22.1 %) [26]. The incidence of high-grade proteinuria (greater than 3.5 g per day) was 2.2 % (95 % CI 1.2–4.3 %), and the incidence of nephrotic syndrome was 0.8 % (95 % CI 0.4–1.8 %) with a relative risk of 7.78 (95 % CI: 1.80–33.62; P = 0.006) .
The kidney histology in patients who develop proteinuria after starting antiangiogenic therapy includes endotheliosis, focal foot process effacement, evidence of glomerular basement membrane damage, including double contours and mesangiolysis. In severe cases fibrin deposition and red blood cell entrapment is also seen. These are the histologic characteristics of renal TMA.
The pathophysiology of this syndrome involves VEGF expressed by podocytes and implicates podocyte–EC crosstalk [27]. Eremina et al. investigated the role of podocyte-derived VEGF in the maintenance of the glomerular filtration barrier. Using conditional mouse genetic models, they specifically ablated VEGF from podocytes alone, and showed that this was sufficient to cause renal TMA [28]. Thus, antiangiogenic therapies act by blocking the effect of podocyte-derived VEGF on adjacent glomerular EC, resulting in disruption of the glomerular filtration barrier with proteinuria, as well as enhanced clotting [29]. Numerous reports have now documented that renal TMA lesions are the most common pattern of glomerular injury found in humans receiving antiangiogenic therapy [28, 30–33]. However, other biopsy findings have also been reported, including immune complex glomerulonephritis [31, 34, 35] and allergic interstitial nephritis [36–38] .
Case #3
You are referred a patient for evaluation of hypomagnesemia on cetuximab therapy. The patient is a 59-year-old male with metastatic colon cancer previously treated with the FOLFOX regimen. He had progression and was changed to cetuximab infusions weekly. Electrolytes and renal function were all normal at the start of the therapy. Four weeks later the patient complained of generalized fatigue and muscle weakness. Chemistries revealed a potassium level of 3.2 mg/dL and magnesium of 0.9 mg/dL. You order a fractional excretion of magnesium, which revealed high urinary magnesium levels despite low circulating magnesium. He received IV repletion andwas started on oral magnesium oxide, titrated up to maintain magnesium level higher than 1.2 mg/dL; despite high oral magnesium doses his magnesium levels do not normalize while on cetuximab, however.
What are the risk factors for the development of hypomagnesemia with cetuximab therapy?
a.
Duration of therapy
b.
Race (African Americans)
c.
Elderly
d.
CKD
Toxicities Associated with EGFR Inhibition
Inhibition of the EGFR is a recognized cause of both hypomagnesemia and secondary hypokalemia. Monoclonal antibody inhibitors of the EGFR include cetuximab and panitumumab. Small-molecule EGFR inhibitors include gefitinib and erlotinib. The overall incidence of hypomagnesemia in patients treated with these drugs is 17 %. Compared to controls not receiving anti-EGFR therapy, the relative risk of hypomagnesemia for cetuximab was 3.87, and the relative risk for panitumumab was 12.55 [39]. Because renal magnesium handling is dependent on normal EGFR signaling, hypomagnesemia reflects adequate EGFR inhibition in vivo and may therefore serve as a surrogate of anticancer efficacy. Indeed, early hypomagnesemia could be a biomarker for superior cancer response. In a study of KRAS wild-type colorectal cancer patients, those that developed an early fall in magnesium > 50 % by day 28 had a higher tumor response rate (55.8 vs. 16.7 %, P < 0.0001) and a longer overall survival (11.0 vs. 8.1 months, P = 0.002) [40].
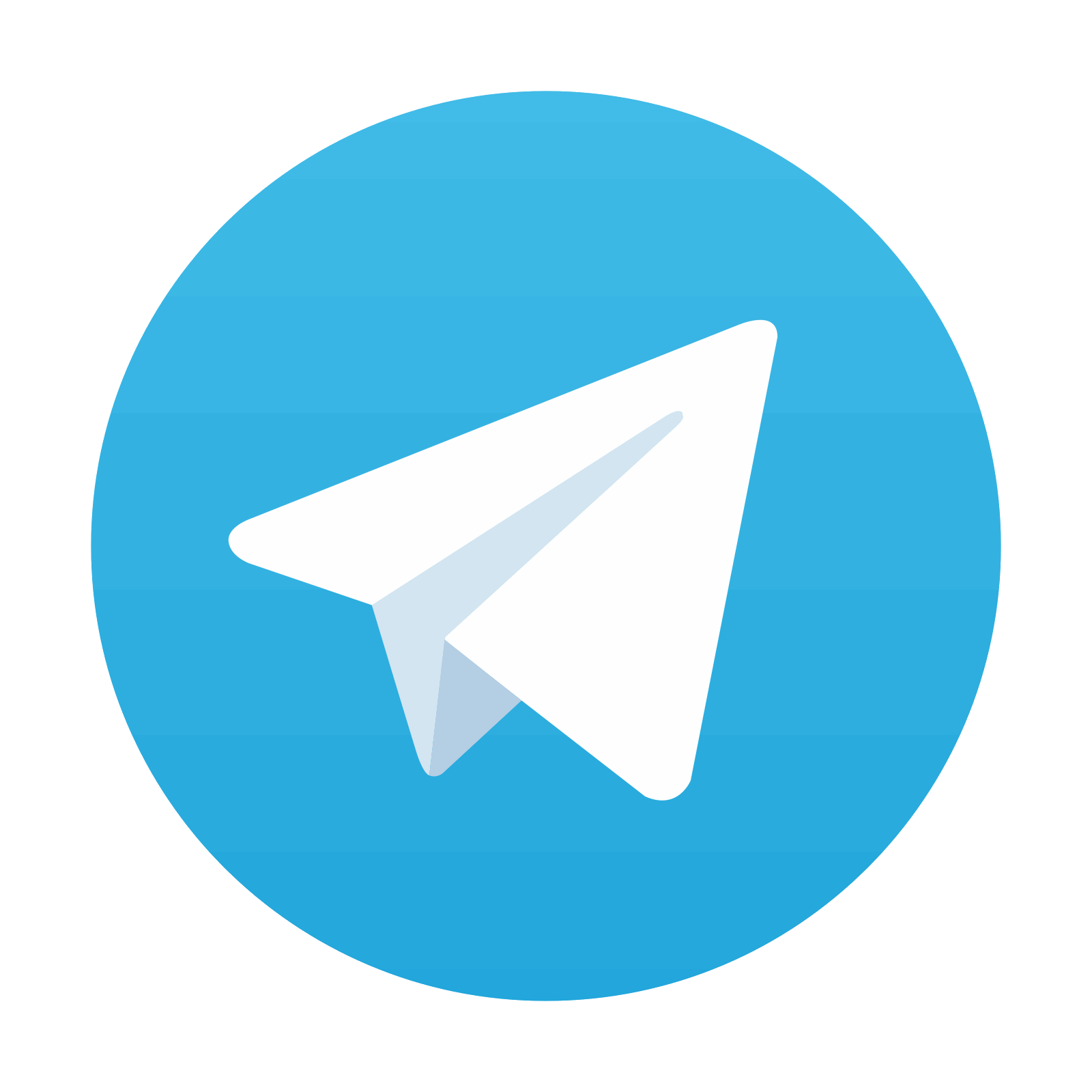
Stay updated, free articles. Join our Telegram channel

Full access? Get Clinical Tree
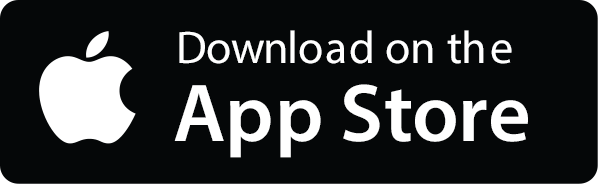
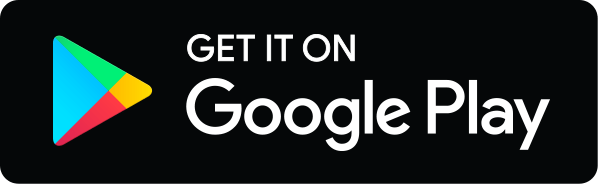