Since 1976, echocardiography (echo) has been used to evaluate cardiac structure and function. Echo uses sound in the high-frequency range (2–10 MHz). Frequency ranges between 2 and 5 MHz are typically used for imaging adults, while frequencies of 7.5 to 10 MHz are used for children and specialized adult applications. The transducer contains a piezoelectric crystal that converts electrical to sound energy, producing sound waves that are transmitted in the form of a beam. A complete transthoracic echocardiogram (TTE) consists of a group of interrelated applications including two-dimensional (2D) anatomic imaging, M-mode, and three Doppler techniques: pulsed-wave (PW), continuous-wave (CW), and color-flow (CF) imaging.1–7 In addition, the quantification of cardiac chamber dimensions, areas, and volumes is an important aspect of a complete examination. Using a combination of these ultrasound techniques, one can assess the anatomy and function of the cardiac valves, myocardium, and pericardium.
Standard views are obtained along three orthogonal planes of the left ventricle (LV): long-axis, short-axis, and the four-chamber plane (Fig. 56-1A–C). The long axis is parallel to the long axis of the LV. The short axis cuts the LV cross-sectionally, similar to slices of bread in a loaf, and is orthogonal to the long axis. Four standard transducer locations are used to obtain complete visualization of the entire heart: parasternal, apical, subcostal, and suprasternal.
Figure 56-1
Standard 2D TTE (A–C) and M-mode (panel D) views in a normal individual. A. Parasternal long-axis view of the right ventricle (RV), interventricular septum, left ventricle (LV) cavity and posterior wall, and both mitral and aortic valves. Since the RV is the most anterior structure, it will be located closest to the transducer beam and is seen at the top of the image display, while the LV and LA (both posterior structures) are farther away from the transducer and are seen at the bottom of the image. B. Parasternal short-axis view of the ventricles at the papillary muscle midventricular level obtained by rotating the transducer 90 degrees. C. Apical four-chamber view, important for evaluation of ventricular function, apical thrombus, mitral and tricuspid valve function. D. M-mode view through the RV and mitral leaflets. This view is useful for detecting RV diastolic collapse in tamponade.

M-mode is a one-dimensional “ice pick” view of the heart (Fig. 56-1D) and is often used for measuring LV systolic and diastolic chamber dimensions and wall thickness.
The Doppler effect is the phenomenon whereby the frequency of sound waves increases or decreases as the sound source moves toward or away from the observer. The resultant Doppler frequency shift can be detected and translated into blood-flow velocity. The velocity of blood can then be used to calculate valvular gradients and areas, intracardiac pressures, and volumetric flow.
Pulsed-wave (PW) Doppler is obtained when a pulse of ultrasound is transmitted intermittently, allowing for estimation of blood-flow velocity at a specific region of interest. It is thus “site-specific.” Its disadvantage is aliasing (or wraparound) of the signal at velocities that are one-half of the pulse repetition frequency (Nyquist limit). This property limits the maximal velocity that can be accurately measured with PW.
Continuous-wave (CW) Doppler records all the velocities along the path of the ultrasound beam. Using CW, the magnitude of the blood-flow velocity and its direction can be accurately recorded. Its disadvantage is that it does not allow localization of the specific site of the velocity obtained along the beam path. CW is used to measure high-velocity jets associated with valvular dysfunction as well as intracardiac pressure gradients. The simplified Bernoulli equation can then be used to convert velocities into pressure gradients (ΔP = 4V2).
Color-flow (CF) Doppler transforms Doppler velocity information into a color-coded scheme. Red denotes flow toward the transducer, while blue represents flow away from the transducer. The relative velocity of blood flow is also depicted, with brighter shades of blue or red representing higher velocities. Turbulent flow is seen as multicolored jets (e.g., due to valvular disease or intracardiac shunts).
Transesophageal echocardiography (TEE) was first introduced clinically in the United States in the 1980s. By placing an echocardiographic probe in the esophagus, the distance between the transducer and the structures of interest is decreased. This allows the use of higher frequency (5- and 7-MHz) transducers that further enhances image quality39. The development of transducer crystals that rotate from 0 to 180 degrees also allows for examination of each cardiac structure from different planes and angles. Acquisition of images is from two basic locations: midesophageal (30–35 cm from the incisors) and midgastric (40–45 cm from the incisors).
Typically, a TEE examination provides additional information but does not replace a transthoracic examination. In the evaluation of the anterior structures, a TTE may in fact provide better image quality. A TEE is useful for the evaluation of patients with limiting body habitus, such as obesity or emphysema, who are not optimally imaged by the transthoracic approach. In addition, certain structures that are not well visualized by TTE [such as the left atrial (LA) appendage, thoracic aorta, and prosthetic valves] can be assessed by the transesophageal approach. A third common indication is to guide intraoperative management during cardiac surgery. Class I indications for perioperative TEE (conditions for which there is evidence and/or general agreement that TEE is useful and effective) are listed in Table 56-1.8 There are other situations in which TEE may also be useful but is not required (i.e., class II indications: conditions in which there is a divergence of opinion about the usefulness/efficacy of a procedure but in which the weight of opinion is in favor of usefulness/efficacy). These include ongoing surgical procedures in patients at increased risk of myocardial ischemia or hemodynamic instability, minimally invasive surgery, or intracardiac mass resection. Examples include, the Cox-Maze procedure, cardiac tumor resection, aneurysmorrhaphy, intracardiac thrombectomy, and pulmonary embolectomy. It is also particularly useful in detecting intracardiac air or aortic atheromatous disease and for selecting anastomotic sites during heart/lung transplantation.8 Antibiotic prophylaxis for infective endocarditis is usually unnecessary but is optional in the high-risk patient (e.g., complex congenital heart disease, prosthetic valve, poor dentition, or prior history of endocarditis).9
|
Relative contraindications for TEE include significant esophageal pathology (e.g., strictures, varices), history of radiation therapy to the mediastinum, and recent esophageal or gastric surgery. Serious complications of TEE are uncommon (<1 percent) but may include aspiration and other problems related to sedation, mucosal trauma, laryngospasm, esophageal or pharyngeal laceration or perforation, methemoglobinemia, and rarely death. Death occurs in less than 1 in 10,000 patients. The passage of the TEE probe is “blind” and is done without direct visualization. The severe complications of esophageal or pharyngeal trauma are signaled by patient complaints of severe pain and inability to swallow, which usually occur after the procedure has ended and sedation has worn off. Esophageal perforation during intraoperative TEE can go undetected for a period of time because of the use of anesthesia and paralytics that prevent patient feedback regarding pain during probe passage.
Three-dimensional (3D) echocardiography has been one of the most important developments in cardiac ultrasound imaging during the last decade. Cardiac structures are seen in relation to each other in all three spatial dimensions39. Acquisition of data includes volumetric ultrasound imaging. It also includes 2D imaging in multiple planes with a known 3D location; as long as the images are linked. 3D echocardiography has been shown to be more accurate in the measurement of LV volumes and ejection fraction (EF) and has been validated against accepted reference techniques such as cardiac magnetic resonance40. 3D echocardiography is not widely available yet and it not part of the routine clinical examination.
Routinely, a qualitative visual estimate of global LV systolic function is obtained by examining LV wall thickening and motion in multiple topographic planes. It is imperative that the endocardium is visualized to make this assessment. In addition, LV systolic function can also be assessed semiquantitatively by using a grading scale (wall motion score). On TEE, images obtained from the esophagus and transgastric orientations are used to assess LV systolic function.
EF is often reported qualitatively as increased (hyperdynamic), normal, mildly, moderately, or severely reduced.8 In addition, the reader often assigns an estimated value to the qualitative assessment. Normal LV EF is 61 ± 10 percent.10
Visual estimation of EF by experienced readers is generally reliable but is limited by reader variability and depends on optimal echocardiographic delineation of the endocardium.
EF can also be quantified from the equation below after determining end-diastolic volume (EDV) and end-systolic volume (ESV):
EF = SV/EDV = (EDV − ESV)/EDV
where SV, stroke volume; EDV, end-diastolic volume; and ESV, end-systolic volume.
Percent FS is another method for estimating LV systolic function and reflects a percent change in LV dimension with systolic contraction. It is calculated from LV end-diastolic and end-systolic dimensions measured by M-mode. The normal range for FS is between 25 and 45 percent. For ventricles that are roughly symmetrical without regional wall motion abnormalities, EF is approximately 2 (percent FS).
Percent FS = (EDD − ESD) / EDD × 100
where EDD, end-diastolic dimension and ESD, end-systolic dimension.
For the assessment of LV regional wall motion, the LV is divided into 16 segments (Fig. 56-2), each of which is given a score from 1 to 5 (1, normal or hyperkinetic; 2, hypokinetic; 3, akinetic; 4, dyskinetic; 5, aneurysmal or diastolically deformed). This grading system differs from the 1-to-5 grading system of TEE often used by cardiac anesthesiologists [1, normal (>30 percent thickening); 2, mildly hypokinetic (10–30 percent thickening); 3, severely hypokinetic (<10 percent thickening); 4, akinetic (no thickening); 5, dyskinetic (paradoxical systolic motion)].12 The wall motion score index is calculated as the sum of segmental wall motion scores divided by the number of segments seen. A score of 1 is normal, and higher wall motion scores indicate more extensive ventricular dysfunction. However, this grading system is more useful for research databases than for clinical use.
Figure 56-2
Sixteen-segment model of the left ventricle. According to guidelines of the American Society of Echocardiography and the Society of Cardiac Anesthesia, the left ventricle is divided into 16 segments (6 basal, 6 midventricular, and 4 apical segments) for evaluation of wall motion and calculation of the wall motion score. (From Shanewise JS, Cheung AT, Aronson S, et al. ASE/SCA guidelines for performing a comprehensive intraoperative multiplane transesophageal echocardiography examination: Recommendations of the American Society of Echocardiography Council for Intraoperative Echocardiography and the Society of Cardiovascular Anesthesiologists Task Force for Certification in Perioperative Transesophageal Echocardiography. J Am Soc Echocardiogr 1999;12:884–900. With permission. Copyright Elsevier.)

LV preload is the LV volume at end-diastole. Normal LV end-diastolic size is 3.5 to 5.7 cm in the parasternal long-axis view. The size of the ventricles and atria may be used for the qualitative evaluation of filling pressures and assessment of hyper- or hypovolemia, particularly intraoperatively with TEE.13 Small LA size and near cavity obliteration of the LV can indicate hypovolemia.14 On the other hand, ventricular and atrial enlargement may indicate hypervolemia or an underlying cardiomyopathy. Doming of the interatrial septum toward the right suggests elevated LA pressure and increased LV preload. One limitation to using TEE for indirect measurement of volume status is that the LV may be foreshortened; it is therefore recommended that the LV be imaged from several different planes to get a more accurate estimate of LV volume.14
Doppler echo is the most common diagnostic tool for assessing diastolic function. Indices of transmitral and pulmonary venous Doppler flows are commonly used to identify patterns of diastolic dysfunction.11
When sinus rhythm is present, PW Doppler at the level of the mitral valve (MV) leaflets records two velocities separated by a period of diastasis (no flow), as shown in Fig. 56-3. After the MV opens, early rapid diastolic filling of the LV occurs (E wave), followed by a period of diastasis, after which late filling occurs due to atrial contraction (A wave). Normally, in individuals less than 60 years old, the peak E:A wave velocity ratio is greater than 1. When there is impaired relaxation without elevated filling pressures, the peak E decreases; hence, the E:A ratio becomes less than 1. In contrast, a “restrictive” filling pattern is seen when both impaired relaxation and elevated filling pressures are present, resulting in a smaller contribution of atrial contraction and an increased E:A ratio (>1.5–2).
Figure 56-3
Pulsed-wave (PW) Doppler of the mitral valve inflow. This figure shows a normal pattern of blood flow through the mitral valve in diastole. The two peaks indicate early (E wave) and late (A wave) diastolic filling separated by a brief period of diastasis (no flow). In individuals less than 65 years old, a normal E:A ratio is 2:1. Deceleration time is the time interval from peak E velocity to baseline.

Four velocities are seen in PW Doppler of the pulmonary veins (Fig. 56-4). Occurring in early systole, the first systolic forward flow velocity (PVS1) is due to the relaxation of the LA, which promotes pulmonary venous flow into the LA. In mid-to-late systole, a second systolic forward flow (PVS2) occurs that is produced by the increase in pulmonary venous pressure occurring after right ventricular (RV) systole. In diastole, a third pulmonary vein velocity is seen (PVD). This is related to the decrease in LA pressure seen after MV opening. The fourth velocity is the atrial flow reversal velocity (PVa) that is related to LA contraction. A pulmonary vein systolic velocity peak less than the diastolic velocity peak is suggestive of elevated LA pressures. Restrictive filling indicative of very high filling pressures is seen when PV S2 is much less than PVD. When higher filling pressures are present, both the duration and peak velocity of PVa are increased. Another sign of elevated LA pressure is when the duration of PVa is longer than the duration of the mitral inflow A wave (by >0.03 s).
Figure 56-4
Pulsed-wave (PW) Doppler of the pulmonary vein. This figure shows a normal pattern of blood flow through the pulmonary veins with four velocities seen (PVS1, PVS2, PVD, PVa). Duration of peak reverse flow velocity during atrial contraction (PVa dur) is also measured. (From Oh JK, Appleton CP, Hatle LK, et al. The noninvasive assessment of left ventricular diastolic function with two-dimensional and Doppler echocardiography. J Am Soc Echocardiogr 1997;10:246–270. With permission. Copyright Elsevier.)

Qualitative evaluation of RV size, wall thickening, and motion is done visually. The subcostal and apical four-chamber views provide the most accurate assessment of RV size. Normal RV size is less than two-thirds of LV size (see Fig. 56-1) and wraps around the LV in a U-shaped manner. The RV size is described as normal if it smaller than the LV and its apex is more basally located than the LV apex; mildly dilated if it is enlarged but to a lesser extent than the LV area; moderately dilated if the RV area is the same as the LV area; and severely dilated if the RV area is larger than the LV. RV hypertrophy is present when the RV free wall thickness is >0.5 cm.
Assessment of RV function is also done visually as is estimation of LV function; however, it is much more difficult to estimate RV function. The function is compared to the function of the LV and is described as normal, mildy reduced, moderately reduced, and severely reduced. It can also be assessed by the amount of displacement of the tricuspid valve annulus toward the apex in systole. A reduction of motion of less than 1.5 cm indicates a significant reduction in RV function. Normal tricuspid annular motion is 1.5 to 2.0 cm.
Septal wall flattening (resulting in a D-shaped LV in the short-axis view) or septal deviation into the LV can be seen in both pressure- and volume-overload conditions of the RV.12 With elevated RV pressure, the septum moves toward the LV with maximum reversed curvature in midsystole. In RV volume overload, the reversed curvature of the septum is seen in middiastole with normalization during systole.
When tricuspid regurgitation (TR) is present, the RV systolic pressure (RVSP) can be estimated (see also “Pulmonary Hypertension,” below). TTE is preferred to TEE for measuring RVSP because of better alignment of the transducer beam with the direction of the regurgitant jet.
Examination of the size and respiratory changes of the venae cavae and hepatic veins can give helpful clues to the patient’s volume status and is also used in the evaluation of pericardial disease. Although TTE is preferred for examination of the respiratory variation of the inferior vena cava (IVC), TEE provides better visualization of the superior vena cava. Dilatation of the superior vena cava (greater than one-half the aortic dimension in the short-axis view) is suggestive of hypervolemia.14
Normal MV function depends on the normal function of all its component parts, including the mitral leaflets, annulus, chordae tendineae, and papillary muscles. Not only is LV function important for normal MV function but also the cavity size, since LV dilation will prevent or limit leaflet coaptation in systole, causing mitral regurgitation (MR). The rectangular posterior leaflet is composed of three scallops (P1, P2, P3), while the semicircular anterior leaflet is divided into thirds for descriptive purposes (Fig. 56-5). Normal MV area is 4 to 6 cm2.
Figure 56-5
Mitral valve structure. The mitral valve consists of two leaflets, the anterior and posterior leaflets, which are separated at the annulus by the posteromedial and anterolateral commissures. The posterior leaflet is rectangular and composed of three scallops (P1, P2, P3). The anterior leaflet is semicircular and is divided into three segments for descriptive purposes (A1, A2, A3). (From Shanewise JS, Cheung AT, Aronson S, et al. ASE/SCA guidelines for performing a comprehensive intraoperative multiplane transesophageal echocardiography examination: Recommendations of the American Society of Echocardiography Council for Intraoperative Echocardiography and the Society of Cardiovascular Anesthesiologists Task Force for Certification in Perioperative Transesophageal Echocardiography. J Am Soc Echocardiogr 1999;12:884–900. With permission. Copyright Elsevier.)

Rheumatic heart disease is the most common cause of mitral stenotic lesions (Fig. 56-6), with leaflet thickening and fusion of the commissures. Subvalvular involvement is also common, which causes chordal thickening, shortening, calcification, and fibrosis. This creates the characteristic fish-mouth valve that is best seen in the short-axis view. Commissural fusion results in bowing of the leaflets in diastole causing a hockey-stick appearance in the long-axis view.
Figure 56-6
Rheumatic mitral stenosis. A. Transesophageal transgastric long-axis view of the left ventricle, the anterolateral papillary muscle (ALPM), the posteromedial papillary muscle (PMPM), mitral valve (MV) leaflets, and left atrium (LA). Note the thickened and partially calcified subvalvular and valvular structures (short arrow). The patient had severe three-vessel coronary artery disease in addition to rheumatic aortic stenosis and atrial fibrillation and underwent successful mitral and aortic valve replacements and coronary artery bypass grafting. B. Continuous-wave (CW) Doppler of the mitral valve intraoperatively. MR, mitral regurgitation; MS, mitral stenosis.

2D echo is the “gold standard” for evaluating mitral stenosis (MS) and allows assessment of the structure and function of the mitral annulus, leaflets, chordae, papillary muscles, LV size and function, LA size, and pulmonary hypertension (RVSP). 2D short axis imaging during diastole allows assessment of the valve area that has been well validated with measurements obtained intraoperatively. The Wilkins echo score15 for rheumatic MS is based on four variables (leaflet mobility, leaflet thickening, subvalvular thickening, and calcifications). Each variable receives a score of 1 to 4 and the individual scores are summed up. A total score equal to or greater than 8 is associated with better outcomes for mitral balloon valvuloplasty. For valves with less favorable scores (>8), cardiac surgery with valve replacement may be the preferred treatment option.
As mentioned above, the 2D short axis imaging on TTE allows direct planimetry of valve area. With this approach, it is important to scan completely above and below the MV plane in order to identify the smallest orifice. Direct planimetry is difficult if image quality is poor.
Calculation of MV area can be done by using the pressure half-time (PHT) method. PHT is the time (milliseconds) for the maximal pressure gradient to decrease by half and is usually equal to the deceleration time multiplied by 0.29.
MV area (cm2) = 220/PHT (ms)
Accurate assessment of MV area requires a near-parallel orientation between directions of blood flow and the Doppler beam. The PHT method has several important shortcomings. It is affected by concomitant aortic regurgitation (AR) or decreased LV compliance.4 Rapid increase in LV diastolic pressure associated with either of these conditions may shorten the PHT and underestimate the extent of stenosis. Severe AR can actually lead to restricted opening of MV leaflet causing functional MS and lengthening of the PHT measurement in addition to anatomic stenosis. Another technique to estimate valve area includes the continuity equation.1,4 The latter approach is also less reliable in the presence of significant aortic and MR. Details of this approach are outlined in references 1 through 11.
As the degree of obstruction to blood flow caused by a stenotic valve increases, the velocity of the blood flow also increases in order to maintain constant flow (conservation of mass or flow). Velocities can then be transformed into pressures with the simplified Bernoulli equation:
ΔP = 4V2
where ΔP, MV pressure gradient (mm Hg) and V, velocity of blood flow across the MV (ms).
The mean MV pressure gradient and MV area are used to estimate the severity of MS and the need for intervention (Table 56-2).
Severity | Mitral Valve Area (cm2) | Mean Gradienta (mm Hg) |
---|---|---|
None | 4–6 | – |
Mild | 1.6–2.0 | <5 |
Moderate | 1.1–1.5 | 5–10 |
Severe | <1 | >10 |
There are three basic mechanisms of MR: primary abnormalities of the mitral leaflets, commissures, or annulus; malfunctioning of the subvalvular structures (chordae tendineae and papillary muscles); and alterations in LV and LA dimensions and function.
The most common cause of isolated severe MR is myxomatous degeneration (Fig. 56-7). The valve itself can also be deformed from rheumatic fever, mitral annular calcification, infective endocarditis, and congenital lesions (cleft MV). Myxomatous degeneration is appreciated by thickened, redundant leaflets and chordae that exhibit excessive motion. The leaflets prolapse into the left atrium in systole. The severity ranges from mild prolapse to severe prolapse or a flail leaflet. Mitral annular calcification is seen more commonly in elderly patients and in patients with renal disease and more commonly affects the posterior annulus. It is exhibited by increased echogenicity and subsequent acoustic shadowing.
Figure 56-7
Myxomatous mitral valve prolapse and mitral regurgitation. A. Transthoracic parasternal long-axis view of the mitral valve with a greater than 2-mm prolapse of the posterior leaflet (MVP) beyond the plane of the annulus. B. Apical four-chamber view shows biatrial enlargement. C. Color-flow Doppler shows severe mitral regurgitation (MR) with an eccentric jet that is “wall hugging” and anteriorly directed (opposite the side of leaflet prolapse).

Other less common causes of leaflet abnormalities include endomyocardial fibrosis, carcinoid disease, drugs (e.g., fenfluramine hydrochloride and phentermine, or Fen-Phen), radiation therapy, trauma, Marfan disease and collagen vascular disease.
Abnormalities in the subvalvular structures include dysfunctional and/or ruptured chordae tendineae and papillary muscles. Ruptured chordae tendineae accounts for a large proportion of MR lesions. Etiologies include idiopathic causes, MV prolapse, infective endocarditis, and thoracic trauma. Papillary muscle dysfunction (with or without frank rupture) is most often seen in the setting of myocardial ischemia or infarction. The posteromedial head of the papillary muscle is most vulnerable to ischemia because of its end-artery vascular supply. Ischemic MR causes restricted leaflet motion resulting in the tenting of the MV in systole. Other causes of dysfunction include dilated cardiomyopathy, myocarditis, hypertension, and chest trauma. Papillary muscle partial or complete rupture is a known potential complication of a myocardial infarction. The ruptured papillary muscle head is visualized in the left atrium in systole and in the LV in diastole.40
Global or regional LV enlargement may dilate the mitral annulus and change the position and axis of contraction of the papillary muscles, leading to dysfunction. This is often called functional MR. Progressive LA and LV enlargement associated with chronic MR further exacerbate the extent of MR because of continued changes in chamber geometry.
Unlike the case in MS, where echo is the accepted gold standard for diagnosis, there is no gold standard for the assessment of MR; therefore, multiple parameters are used in estimating the severity of MR.16 The purpose of echo in MR is to determine the etiology, mechanism, and severity of regurgitation; determine the need and type of surgery; and evaluate other valves, RV and LV function, and the presence of pulmonary hypertension. The most important aspect is to determine the hemodynamic significance of the regurgitation based on LV size (particularly end-systolic dimension), LV systolic function (EF), and the presence and degree of LA enlargement and pulmonary hypertension (RVSP). Regurgitant jet size and area may contribute to the assessment of MR but often correlate poorly with MR severity.16 The degree of MR intraoperatively may appear less or more severe compared to the degree preoperatively, because of different hemodynamic and ischemic conditions (e.g., lower blood pressure or relief of ischemia will reduce the amount of regurgitation).17
MR results in LV volume overload. Acutely, the LV is able to empty more efficiently, such that forward cardiac output is maintained. Chronic MR leads to LV dilation and eventual decrease in contractility. The LA also increases in size to accommodate the regurgitant volume. Pulmonary artery pressures increase both in acute MR as well as in chronic MR due to elevated LA pressures. This can subsequently lead to pulmonary hypertension. The direction and shape of the regurgitant jet may aid in assessment of valve pathology and severity. Anterior leaflet pathology produces a posteriorly directed jet and posterior leaflet pathology causes an anterior directed jet. A central jet is often seen in dilated cardiomyopathy as mentioned earlier due to a dilated LV and/or valve annulus.40
Large regurgitant jet: >40 percent of LA area.
Wide vena contracta (the narrowest part of the regurgitant color jet at its origin): ≥7 mm.
Eccentric wall-impinging regurgitant jet (Fig. 56-7B).
Dilated LV: end-systolic dimension ≥45 mm or end-diastolic dimension ≥70 mm.
LV EF <55 to 60 percent.
Dilated LA (≥5.5 cm), although the LA may not be dilated in acute MR.
Pulmonary hypertension: resting RVSP >50 mm Hg.
Restrictive mitral filling pattern: high peak early transmitral velocity (E wave) >1.5 ms.
Pulmonary vein systolic flow reversal: A normal pulmonary vein Doppler pattern has a systolic (S) wave larger than the diastolic (D) wave. As the MR progresses, the S wave decreases until it may become reversed. While a reduced S wave is a nonspecific finding,18 the presence of a reversed S wave has high specificity for significant MR. The absence of a reversed S wave does not exclude significant MR.
Effective orifice area (ERO) ≥0.4 cm2.
Table 56-3 summarizes the important echo features of MV stenosis and regurgitation.
Morphology | Mitral annulus, leaflets, chordae, papillary muscles, LV size and function, LA size, pulmonary hypertension (RVSP) |
Stenosis |
|
Regurgitation |
|
Mitral valve prolapse (MVP) is the most common cause of MR in patients undergoing cardiac surgery in the United States.19 MVP is the systolic billowing or displacement of at least 2 mm of either mitral leaflet into the LA beyond the plane of the mitral annulus in the parasternal or apical long-axis views (see Fig. 56-7). Recent criteria for echo diagnosis of MVP have become more stringent,20 leading to increased specificity of the criteria for MVP while at the same time preserving sensitivity for the detection of MVP complications. Classic MVP is defined as equal to or greater than 5 mm of leaflet thickening (myxomatous changes) of the prolapsing leaflet, while nonclassic prolapse is leaflet thickening less than 5 mm. Compared to nonclassic MVP, classic MVP has a worse prognosis, with most complications of MVP (significant MR and congestive heart failure, MV surgery, infectious endocarditis) arising in patients with classic MVP.19 Asymmetrical prolapse of the leaflets is associated with progression to more significant disease (flail leaflet and severe MR).
Determining which segment of the mitral leaflet tissue is prolapsing is essential for proper MV repair. MR associated with MVP often has an eccentric jet directed opposite to the prolapsing leaflet (e.g., anteriorly directed jet if posterior leaflet prolapse is present). In contrast, in MR associated with rheumatic MS, the regurgitant jet is directed toward the affected leaflet due to restricted leaflet motion (e.g., anteriorly directed jet if the anterior leaflet is calcified). MR associated with MVP may be due to flail leaflet or chordal rupture resulting from myxomatous involvement of the chordae or leaflet. Chordal rupture is diagnosed when the chords are seen as mobile echodensities attached to a flail or partially flail leaflet (Fig. 56-8).21 Chordal rupture may be difficult to distinguish from vegetations of infective endocarditis. Mitral annulus calcification (MAC) may also be seen in patients who have MVP.
Figure 56-8
Ruptured chord attached to a flail segment of the posterior mitral leaflet due to myxomatous disease. Intraoperative transesophageal echocardiogram showing the ruptured chord as an echodense linear filament attached to the flail leaflet, prolapsing into the left atrium during systole. LA, left atrium; LV, left ventricle.
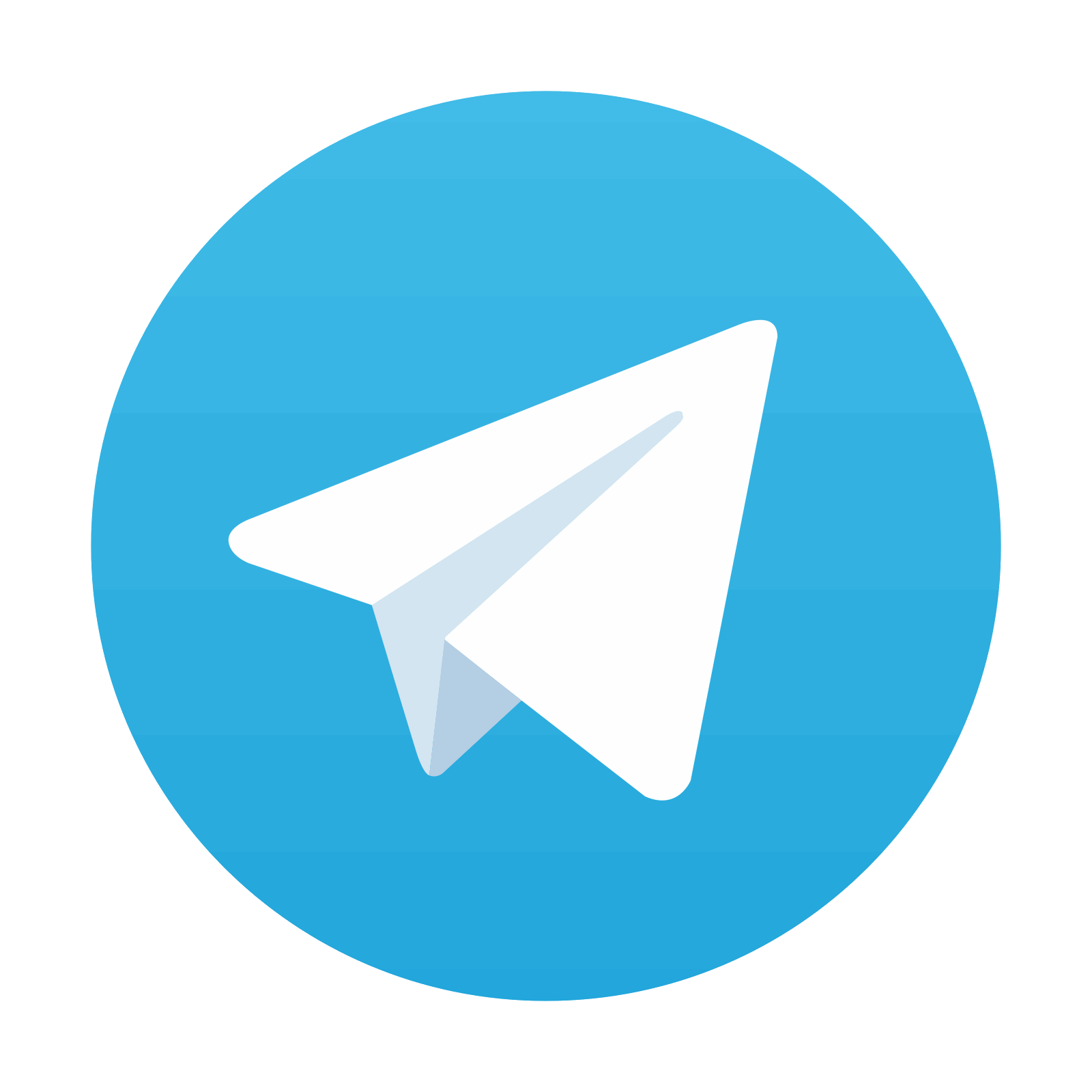
Stay updated, free articles. Join our Telegram channel

Full access? Get Clinical Tree
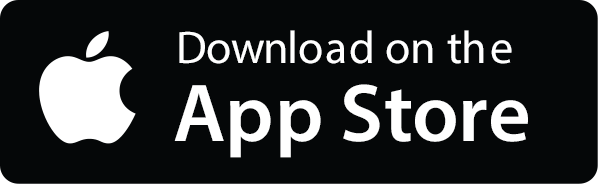
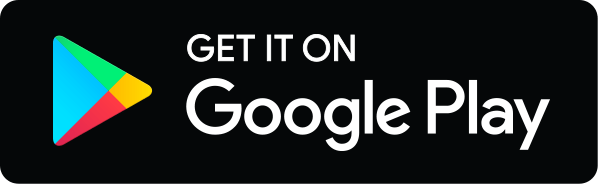
