1. What are dysnatremias?
The term dysnatremia applies when an aberration in plasma sodium concentration is present. Changes in plasma sodium concentration can result in fluid shifts between the intra- and extracellular compartments of the body. In the healthy state, the body’s osmoregulatory system maintains the plasma sodium concentration between 135 and 145 mEq/L. Failure of this system begets an imbalance of free water intake and excretion. When free water intake exceeds excretion, the plasma sodium concentration decreases below 135 mEq/L, a condition known as hyponatremia. In contrast, hypernatremia, defined as a serum sodium concentration >145 mEq/L, occurs when electrolyte-free water excretion exceeds intake. Less commonly, pure loss or addition of sodium without a primary disturbance in water balance may also lead to hypo- or hypernatremia.
2. How is plasma osmolality determined?
Plasma osmolality can be calculated with the following equation:
As the equation implies, serum sodium concentration is by far the main determinant of plasma osmolality. Plasma osmolality can be directly measured with an osmometer. An osmolar gap exists if there is greater than a 10 mOsm/kg H 2 O discrepancy between the calculated and measured osmolality, indicative of the presence of a solute not routinely measured in plasma.
3. Can osmolality be normal despite an abnormal serum sodium concentration?
Yes! Serum sodium concentration is usually measured using indirect ion-selective electrodes with specimen dilution and depends on the assumption that water comprises approximately 93% of plasma. The presence of high concentrations of plasma lipid or protein, however, will reduce the aqueous contribution to plasma volume. As a result, the measured serum sodium concentration will be falsely low. This condition is known as pseudohyponatremia. Ultracentrifugation and separation of the lipid layer can correct for lab artifact due to hyperlipidemia. Direct ion-selective electrodes are not confounded by hyperlipidemia or hyperproteinemia, but only about a third of chemical analyzers use this technique. Blood gas laboratories use ion-selective electrodes and thus are not susceptible to this artifact.
4. Is plasma osmolality the same as plasma tonicity?
No! While plasma hypertonicity implies hyperosmolality, hyperosmolar plasma is not necessarily hypertonic. The effective osmolality, often termed plasma tonicity, denotes the concentration of osmoles in plasma that do not move freely across the cell membrane. Such osmoles can generate concentration gradients across cell membranes and in turn drive shifts in water between the extra- and intracellular compartments. While sodium and glucose as effective osmoles contribute to tonicity, urea and ethanol are ineffective osmoles as they freely cross cell membranes. High concentrations of these latter molecules confer hyperosmolality without affecting tonicity. In the absence of ethanol or other unexpected solutes, the effective osmolality is simply the measured osmolality minus blood urea nitrogen (BUN) (mg/dL)/2.8.
6. In what hyponatremic states can a patient be hyperosmolar or hypertonic?
The two most clinically relevant occurrences of hyperosmolar hyponatremia are azotemia and hyperglycemia. Buildup of nitrogenous waste, that is, urea, in the setting of impaired kidney function increases plasma osmolality. If present alone, kidney dysfunction does not lead to a change in serum sodium concentration. However, if a simultaneous disorder in water balance leading to hyponatremia is present, hyponatremia with normal or elevated serum osmolality may result. Such patients are truly hypotonic , because the decreased sodium, an effective osmole, leads to a decrease in tonicity while urea, an ineffective osmole, does not increase tonicity.
In hyperglycemia, increased serum glucose increases both the total and effective plasma osmolality, leading to a hypertonic state. The hypertonic plasma draws water from the cells into the extracellular compartment, lowering the serum sodium concentration. For every 100 mg/dL increase in glucose above normal, the serum sodium falls by approximately 1.6 mEq/L even as tonicity rises due to the hyperglycemia. In cases of more severe hyperglycemia (i.e., serum glucose >400 mg/dL), the ratio approximates a 2.4 mEq/L drop in serum sodium for every 100 mg/dL rise in serum glucose. A similar process occurs with administration of mannitol. Any such correction factor is an approximation, and osmolality should be measured directly when clinically relevant.
7. What causes hypotonic hyponatremia?
While there are many discrete etiologies of hypotonic hyponatremia, there are three general processes:
- 1.
Inadequate solute intake
- 2.
Excess electrolyte-free water intake
- 3.
Retention of electrolyte-free water
Not infrequently, the latter two are present simultaneously.
8. What are the clinical manifestations of hyponatremia?
Not all patients with hyponatremia will be symptomatic. While neurons are especially sensitive to osmotic stress, astrocyte-mediated expulsion of electrolytes into the extracellular fluid (ECF) over several hours followed by organic osmolytes (e.g., taurine and glutamate) over the ensuing 24 to 48 hours prevents intracellular swelling. Thus, patients who develop a serum sodium concentration between 125 and 135 mEq/L over greater than 48 hours will often have minimal or no symptoms. Observational studies, however, have demonstrated an association between even mild hyponatremia (serum sodium concentration 130 to 134 mEq/L) and increased in-hospital mortality, falls, and reduced bone density. Other studies have noted impaired motor function and gait even with modest reductions in serum sodium concentration. The combination of cognitive impairment, unsteady gait, increased falls, and osteoporosis constitute a “perfect storm” for development of bone fractures. An increase in fracture risk has indeed been documented. Rapid decreases in plasma sodium concentration (from >134 to <125 mEq/L within 48 hours) are faster than compensatory loss of intracellular solute, so the relatively hypertonic intracellular compartment osmotically absorbs water. This cellular swelling particularly affects the brain given the limit to expansion within a tightly encasing cranium. Thus, the manifestations of hyponatremia are predominantly neurologic and include nausea, vomiting, malaise, headache, lethargy, confusion, and muscle cramps. In severe cases, seizures, coma, tentorial herniation, and neurogenic pulmonary edema can occur.
9. What is the initial diagnostic approach to hypotonic hyponatremia?
Once hypotonicity is confirmed with an effective osmolality <275 mOsm/kg H 2 O, the urine osmolality is instrumental is assessing whether or not the kidneys are reabsorbing electrolyte-free water. In the absence of chronic kidney disease, a urine osmolality <100 mOsm/kg indicates an intact diluting mechanism and a state of maximal electrolyte-free water excretion. Conversely, a urine osmolality >100 mOsm/kg suggests that electrolyte-free water reabsorption is occurring, which would be inappropriate from the point of view of tonicity alone. Patients with chronic kidney disease may have an impairment in maximal ability to dilute the urine. In such cases a urine osmolality of <200 mOsm/kg reflects maximal free-water excretion. The diagnostic approach to hyponatremia is further outlined the Fig. 73.1 .

10. How is hypotonic hyponatremia with dilute urine further assessed?
If the urine is maximally dilute, the pituitary-kidney axis is doing what it is supposed to be doing, and hypotonic hyponatremia is arising from electrolyte-free water ingestion in excess of what the kidneys can excrete. In the typical Western diet, approximately 800 mOsm of solute derived from ingested electrolytes and protein is consumed or generated and excreted in the urine on a daily basis. Assuming a maximal urine diluting capacity of 50 mOsm/kg H 2 O, such a solute load is sufficient to permit excretion of up to 16 L of urine (800 mOsm solute/50 mOsm/kg = 16 L). If electrolyte-free water intake exceeds this limit, the kidneys’ electrolyte-free water excretory capacity is overwhelmed, and hypotonic hyponatremia ensues. This condition, known as primary polydipsia, typically occurs among psychiatric patients. Inadequate solute intake impairs free water clearance. If solute intake decreases to 200 mOsm/day and intake of protein that would lead to urea production is reduced—as occurs with a “tea and toast diet” or beer potomania—the volume of maximally dilute urine the kidneys can excrete may be as little as 4 L. Water ingestion above this amount can more easily be achieved and will also result in hyponatremia.
11. What causes hyponatremia with concentrated (less than maximally dilute) urine?
Electrolyte-free water reabsorption. Water reabsorption is mediated by binding of vasopressin, or antidiuretic hormone (ADH—same stuff, 2 names), to vasopressin 2 (V2) receptors on the basolateral membranes of principal cells in the collecting ducts of nephrons. Vasopressin binding in turn stimulates insertion of aquaporin water channels into the luminal membrane. Water then flows through these aquaporin channels into cells and onward to the hypertonic medullary interstitium via constitutively active basolateral aquaporin channels. ADH release is regulated by osmoreceptors in the hypothalamus. Normally, vasopressin release is inhibited when the serum sodium concentration falls below 135 mEq/L. However, poor effective circulating volume—as sensed by carotid baroreceptors as a decrease in arterial pressure—serves as a potent stimulus for ADH secretion despite osmoregulatory inhibition. Hyponatremia in the setting of hypovolemia arises from hemodynamically driven ADH release and should improve with volume repletion. ADH secretion in the setting of arterial underfilling, due either to a low output state in the case of heart failure or to splanchnic vasodilation in cirrhosis, occurs by the same hemodynamic mechanism. These patients, conversely, will appear hypervolemic on exam. They are hyponatremic and hypotonic despite a clear excess in total body sodium content.
12. How is hypervolemic hyponatremia managed?
Unlike hypovolemic hyponatremia, the mainstay of therapy for hypervolemic hyponatremia arising from either heart failure or cirrhosis is water restriction. Loop diuretics can also be effective in hyponatremia associated with heart failure. By inhibiting sodium reabsorption in the ascending limb of the Loop of Henle, loop diuretics decrease the tonicity of the medulla and thereby diminish the driving force for ADH-mediated water reabsorption in the collecting duct. (By acting in the distal tubule of the cortex, thiazide diuretics block sodium reabsorption only at a site where sodium is reabsorbed without water. Moreover, generation of a hypertonic medullary interstitium is unaffected, leaving conditions persistently favorable for electrolyte-free water reabsorption. Thus, thiazide diuretics tend to induce hyponatremia .) When water restriction and loop diuretics fail, a vasopressin receptor antagonist (vaptan) may be useful. Conivaptan (a non-selective V1/V2 antagonist) and tolvaptan (a pure V2 antagonist) can be used to promote aquaresis in volume-overloaded heart failure patients with mild-to-moderate symptomatic hyponatremia. Conivaptan should be used with caution in patients with cirrhosis due to a theoretically increased risk for hypotension or variceal bleeding via antagonism of the V1 pressor receptor. Tolvaptan is relatively contraindicated in patients with cirrhosis as well due to the possibility of hepatotoxicity. More common side effects associated with these medications include thirst and dry mouth.
13. What etiologies give rise to euvolemic hyponatremia with less than maximally dilute urine?
Most commonly, euvolemic hyponatremia with less than maximally dilute urine arises from the syndrome of inappropriate antidiuretic hormone secretion (SIADH, discussed below). Other potential causes include exercise-associated hyponatremia (EAH), methylenedioxy- N -methamphetamine (MDMA or ecstasy) ingestion, hypothyroidism, and glucocorticoid insufficiency. EAH is classically observed among marathon runners, who become overhydrated in the face of excessive water ingestion coupled with impaired water excretion from non-osmotically driven ADH release. By stimulating both ADH secretion and polydipsia via activation of serotonergic pathways, MDMA ingestion can lead to acute, severe hyponatremia and death if not promptly addressed. Although the mechanism is not definitively clear, the negative systemic effects of profound thyroid hormone deficiency on cardiac output and peripheral vascular resistance appear to trigger nonosmotic ADH secretion and hyponatremia. Since cortisol suppresses ADH release, isolated glucocorticoid deficiency can result in hyponatremia from uninhibited vasopressin secretion. This entity is distinct from Addison disease or other causes of adrenal gland destruction where mineralocorticoid deficiency and consequent volume depletion are present along with glucocorticoid lack.
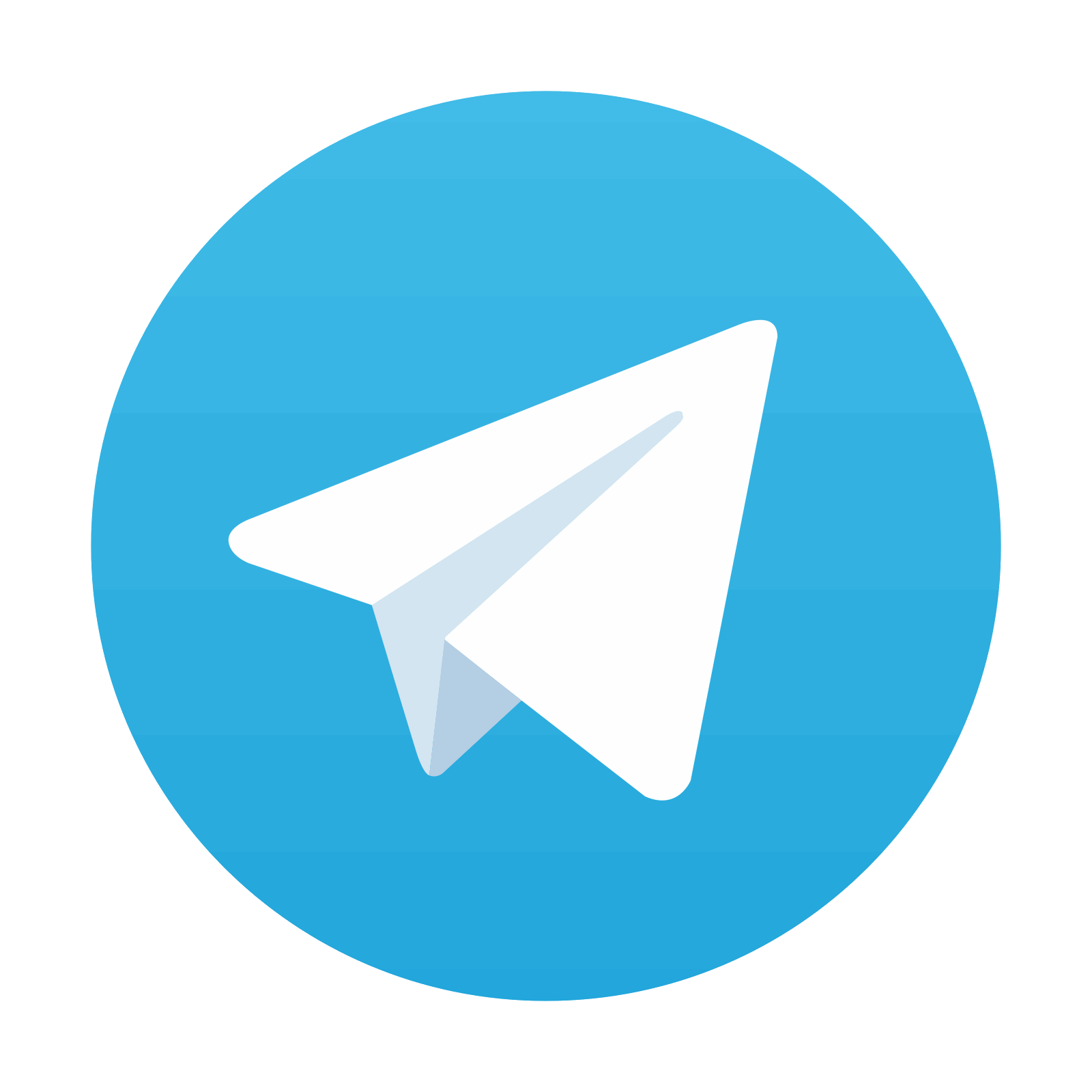
Stay updated, free articles. Join our Telegram channel

Full access? Get Clinical Tree
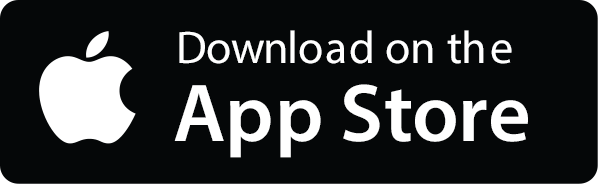
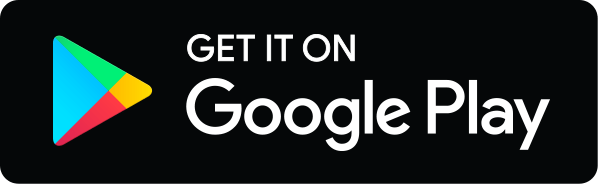