Fig. 7.1
Basic structure of triglycerides
3 Triglyceride Digestion
3.1 Intragastric Events
Several important steps in triglyceride digestion occur within the stomach . Lipase activity is readily detected in the gastric fluid, and in normal adult humans appears to account for 10–30 % of triglyceride hydrolysis. Lipase is produced in serous glands at the base of the tongue or in acinar cells in pharyngeal tissue, and the lipase is then swallowed along with the food bolus. Lingual lipase generating free fatty acids in the mouth and pharynx may play a role in gustatory fatty acid signaling (fatty acid taste). In humans, the lipase found in gastric fluid appears to originate mainly within chief cells of the gastric mucosa . Secretion of gastric lipase can be stimulated by cholinergic agents, gastrin , and possibly other gastrointestinal hormones such as cholecystokinin . Gastric lipase has a broad pH optimum (pH 2–6) and is resistant to pepsin and therefore active within the environment of the stomach. Gastric lipase emptied into the duodenum continues to be active within the environment of the small intestine , although pancreatic lipase is the enzyme responsible for most of the triglyceride hydrolysis (see below). Gastric lipase preferentially hydrolyzes the ester bond in the 3 position of triacylglycerol, generating mainly fatty acids and diglycerides.
Within the stomach , there is acid-peptic digestion of the protein component of food lipoproteins and liberation of oil droplets. The churning action of gastric contractions against the closed pylorus results in the formation of an emulsion containing small lipid particles (less than 2 nm) that can then be emptied from the stomach into the duodenum. These small oil droplets with a large surface-to-volume ratio are the preferred substrate for the colipase –pancreatic lipase enzyme system that is responsible for the majority of triglyceride digestion in adults (see below).
3.2 Hormonal Regulation of Biliary and Pancreatic Secretion
As the gastric fluid empties into the duodenum, there is initiation of a coordinated series of events designed to properly deliver biliary and pancreatic secretions into the small intestine to continue the process of triglyceride digestion. Exposure of the duodenal and upper jejunal mucosa to gastric acid stimulates secretion of the peptide hormone secretin from neuroendocrine cells in the mucosa into the portal circulation. Secretin , in turn, stimulates production of a bicarbonate–rich fluid by the pancreatic ductular cells. Delivery of this alkaline pancreatic fluid into the duodenum partially neutralizes the gastric acid secretion. The pH of the upper small intestine is therefore adjusted to approximately pH 6.5, which is optimal for the activity of the colipase -pancreatic lipase enzyme system. Fatty acids and certain amino acids in the intestinal fluid stimulate the release of a second peptide hormone, cholecystokinin (CCK) (also called pancreozymin ) from the mucosa into the portal circulation. CCK induces digestive enzyme secretion by the pancreatic acinar cells , including the secretion of pancreatic lipase and colipase. In addition, CCK also stimulates contraction of the gallbladder and relaxation of Oddi’s sphincter , resulting in the delivery of concentrated bile into the small intestine. Secretin, CCK, and other hormones produced in the intestinal mucosa also regulate gastric motility , preventing excessively rapid delivery of the food bolus into the intestine and overwhelming of the digestive processes. For a detailed discussion of the regulation of gastric emptying, pancreatic, and biliary secretion, see Chaps. 3, 4 and 12.
3.3 Pancreatic Lipase
The enzyme responsible for most of the triglyceride digestion in adult humans is pancreatic lipase . This 449-amino-acid glycoprotein is secreted from the pancreas in its active form. Pure pancreatic lipase has a pH optimum of pH 8–9, but in the presence of bile salts the pH optimum is reduced to pH 6–7, the typical pH of the upper small intestine . At acid pH (less than pH 4.0), pancreatic lipase is irreversibly inactivated; therefore, neutralization of gastric acid by bicarbonate in pancreatic juice is essential for preserving enzyme activity. Pancreatic lipase is an interfacial enzyme that is most active at an oil-water interface and thus efficiently hydrolyzes triglyceride present in the small lipid droplets that are emptied from the stomach into the small intestine. In the small intestine, the oil droplets are coated on their surfaces with bile salts, phospholipids and other compounds that inhibit the binding of pure pancreatic lipase (Fig. 7.2). In order to achieve effective association of pancreatic lipase with the surface of these oil droplets, a protein cofactor known as colipase is required which forms a complex with pancreatic lipase and bile components that associate with lipid droplets. Colipase is secreted into pancreatic fluid as a proprotein, and an amino-terminal pentapeptide is cleaved by trypsin in the small intestinal fluid to produce the 96-amino-acid form that can associate with the lipid droplet. In addition, fatty acids also appear to facilitate association with pancreatic lipase and the oil-water interface; therefore partial triglyceride hydrolysis by gastric lipase may be an important mechanism to enhance the efficiency of the colipase-pancreatic lipase enzyme system. The amounts of both pancreatic lipase and pro-colipase in pancreatic fluid are increased by dietary fat and secretin , and fat feeding increases the mRNA abundances for both pancreatic lipase and pro-colipase in the pancreatic acinar cells .
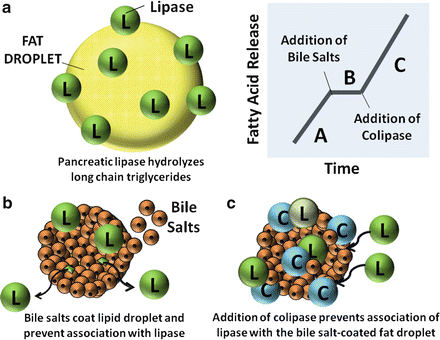
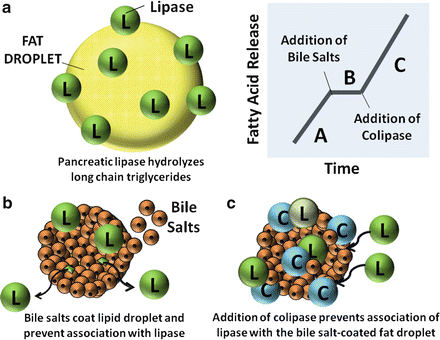
Fig. 7.2
Actions of pancreatic lipase , bile salts and colipase in fat digestion. (a) Pancreatic lipases break down triglycerides. (b) Bile salts coat lipid droplet and displace lipases. (c) Addition of colipases anchor lipases to bile salt-coated fat droplet (Adapted from American Gastroenterological Association Teaching Slide Collection 19 ©, Bethesda, Maryland, slide 29; Used with permission)
Pancreatic lipase preferentially hydrolyzes the first and third ester bonds in triglyceride, forming fatty acids and 2– or β–monoglycerides (Fig. 7.3). The colipase pancreatic lipase system is highly efficient, and triglyceride digestion is nearly complete within the first 100 cm of the proximal jejunum . There is a great excess of pancreatic lipase in pancreatic secretions, and lipase activity must be less than 10 % of normal for triglyceride absorption to be impaired and for steatorrhea to occur.
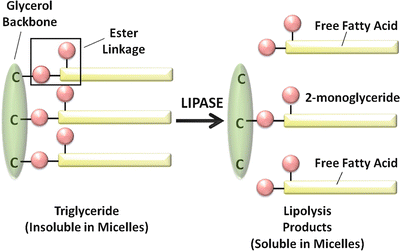
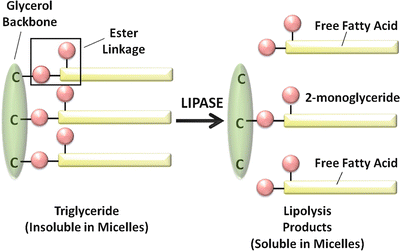
Fig. 7.3
Digestion of triglyceride by pancreatic lipase (Adapted from American Gastroenterological Association Teaching Slide Collection 5 ©, Bethesda, Maryland, slide 10; Used with permission)
3.4 Other Lipases
In addition to pancreatic lipase , pancreatic secretions contain another lipase that requires bile salts for activity. In contrast to pancreatic lipase, which has a high degree of substrate specificity for triacylglycerol, bile salt–activated lipase will catalyze the hydrolysis of carboxyl ester bonds not only in acylglycerols, but also in other dietary fats such as cholesteryl esters, fat-soluble vitamin esters, and phospholipids. Human bile salt-activated lipase, which has a molecular weight of approximately 100 kd, represents about 4 % of total pancreatic juice protein. Bile salts likely induce conformational changes in the enzyme that enhance access of the active site to bulky lipid substrates and provide additional lipid-binding capability. Trihydroxylated bile salts , such as cholate, taurocholate , or glycocholate, are more potent activators than dihydroxylated bile salts , such as taurochenodeoxycholate; and the 7a-hydroxyl group of bile salts is extremely important for activation. Bile salt-activated lipase catalyzes the complete hydrolysis of triglyceride to fatty acids and glycerol. Patients with congenital deficiency of pancreatic lipase or colipase have been described, and although they have steatorrhea , some fat digestion and absorption continue due to the lipolytic activities of the gastric and bile salt-activated lipases.
Human milk contains a bile salt-activated lipase that is essentially identical to the pancreatic enzyme, differing principally in the glycosylation pattern. The concentration of bile salt-activate lipase in milk is approximately 100 mg/L. Because of the enzyme’s bile-salt requirement, the enzyme will not catalyze hydrolysis of lipids in breast tissue or milk until it reaches the duodenum and is exposed to bile.
In adults, pancreatic lipase has 10–60 times the lipolytic activity of bile salt-activated lipase and is responsible for the majority of triglyceride digestion. In the neonate and especially in premature infants, however, pancreatic function is immature, and secretion of pancreatic lipase is inadequate to support efficient triglyceride hydrolysis. In addition, the colipase -pancreatic lipase system is relatively inefficient in digesting milk-fat globules. Milk bile salt–activated lipase and gastric lipase (which is present in neonates at about the same level as adults), therefore, play important roles in ensuring adequate triglyceride digestion during early development. Other pancreatic lipase–related proteins (PLRP1 and 2) in pancreatic juice may also play a role in neonatal triglyceride digestion. As noted above, the generation of some fatty acids by the actions of milk bile salt-activated lipase and gastric lipase on milk triglycerides will increase colipase binding to the lipid droplet and to pancreatic lipase, thereby enhancing pancreatic lipase activity.
3.5 Phase Contrast Studies
The process of triglyceride digestion has been studied in vitro using phase contrast microscopy (Fig. 7.4). Small oil droplets are suspended in buffer containing concentrations of bile salts and phospholipids similar to those in intestinal fluid, and hydrolysis is initiated by addition of pancreatic juice. Initially, one observes the formation of calcium fatty acid soaps on the surface of the lipid droplet, which, if unchecked, would limit access of colipase and lipases to the surface of the droplet and limit further lipolysis. Formation of calcium fatty acid soaps, however, is inhibited by β-monoglycerides . This has been offered as a potential teleologic explanation for the structural specificity of pancreatic lipase , as the partial breakdown of triglyceride to fatty acids and β-monoglyceride would limit production of fatty acid soaps and favor continued digestion of the lipid droplet.
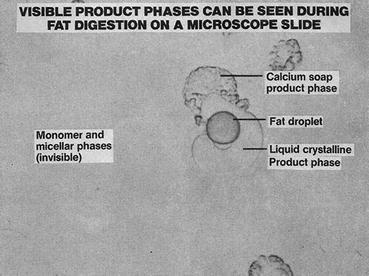
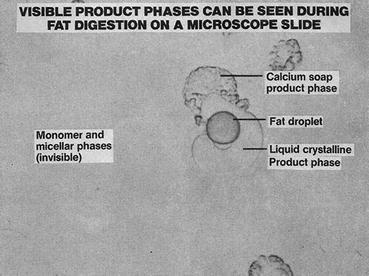
Fig. 7.4
Product phases of fat digestion seen by light microscopy (Adapted from American Gastroenterological Association Teaching Slide Collection 19 ©, Bethesda, Maryland, slide 31; Used with permission)
The second, or viscous isotropic, phase involves the formation of liquid crystals containing protonated fatty acids and monoglycerides. Continued accumulation of fatty acids and monoglycerides on the surface of the lipid droplet, however, would result in product inhibition of the lipases and would limit lipolysis. The products of triglyceride digestion, however, are removed from the droplet surface by formation of vesicles and mixed micelles (see below).
4 Triglyceride Absorption
4.1 Bile Salts and Micelle Formation
Bile salts are a family of amphiphilic compounds that are important for the efficient absorption of the products of triglyceride hydrolysis. At concentrations of approximately l–5 mmol/L, bile salts form micelles, macromolecular aggregates containing up to 20 or more bile salt molecules. These disc or spherical shaped structures have the polar aspect of the bile salts facing the outside of the structure and the hydrophobic portion pointing toward the interior of the micelle. Fatty acids and other lipids are incorporated between the bile salts and in the interior of these structures, forming mixed micelles (Fig. 7.5). This results in a remarkable increase in the solubilization of fatty acids, monoglycerides, and other lipids in the aqueous environment of the luminal contents (Fig. 7.6). It should be noted, however, than even in the complete absence of luminal bile salts, some fatty acid absorption (approximately 50–75 %) still occurs. Some data indicate that unilamellar vesicles formed in the intestinal contents at low bile salt concentrations may be a vehicle for some fat absorption. Adequate luminal bile salt concentration for micelle formation, however, is required for the normal highly efficient (95 % or greater) absorption of dietary triglycerides.
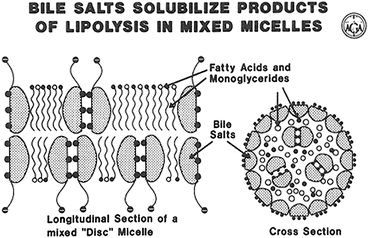
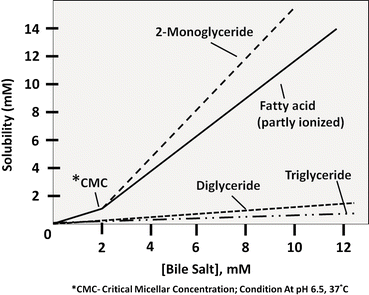
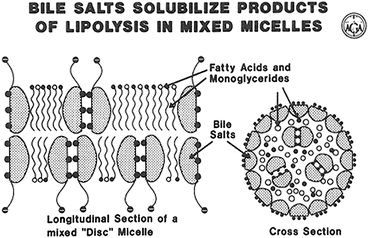
Fig. 7.5
Structure of a mixed disc micelle (Adapted from American Gastroenterological Association Teaching Slide Collection 19 ©, Bethesda, Maryland, slide 35; Used with permission)
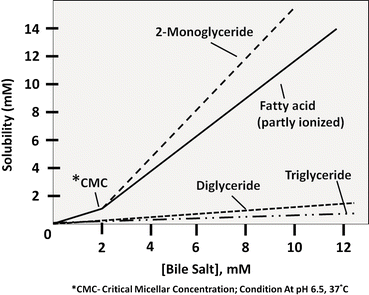
Fig. 7.6
Solubility of fat digestion products in bile salt solution (Adapted from American Gastroenterological Association Teaching Slide Collection 19 ©, Bethesda, Maryland, slide 39; Used with permission)
4.2 Uptake of Fatty Acids and β-Monoglycerides into the Enterocyte
Along the apical brush-border membrane surface of the enterocytes is a layer of structured water known as the unstirred water layer. Because of hydrophobic nature of fatty acids and β-monoglycerides , the unstirred water layer has been considered to represent the major permeability barrier limiting the rate of uptake of the products of triglyceride hydrolysis into the intestinal cells. The rate of flux of a lipid across the unstirred water layer will be determined by the product of a diffusion rate constant and the concentration gradient (Table 7.1). Because of their large size, the diffusion constant of mixed micelles is somewhat smaller than that of monomeric fatty acids in solution. This, however, is more than offset by the marked increase in the concentration of fatty acids achieved in mixed micelles compared with that possible with monomeric fatty acids in aqueous solution. The flux of fatty acids across the unstirred water layer in mixed micelles is therefore estimated to be more than 100 times greater than that achieved with fatty acid monomers. The fate of the mixed micelles at the apical brush-border membrane surface is still somewhat unclear. The most accepted theory is that the micellar fatty acids are in equilibrium with monomeric fatty acids in the unstirred water layer and that the monomeric fatty acids then cross the brush-border membrane.
Table 7.1
Effect of micellar solubilization of fatty acid on diffusive flux through unstirred water layera
From | Fatty acid concentration (mmol/L) | D × 104 (cm2/s) | J × 104 (mmol/L/cm-s) | Relative flux |
---|---|---|---|---|
Molecules | 0.01 | 7 | 0.07 | 1 |
Micelles | 10 | 1 | 10 | 142 |
The uptake of fatty acids across the enterocyte brush-border membrane occurs by both passive diffusion and by protein–mediated mechanisms. Protonate long chain fatty acids diffuse across the brush-border membrane by “flip flopping” through the membrane lipid bilayer down a steep concentration gradient. Several proteins have been identified that potentially play roles in fatty acid uptake. CD36 is a 472 amino acid heavily glycosylated transmembrane protein that appears to be involved in fatty acid uptake in many tissues. In the small intestine , it is mostly expressed on the brush-border membranes of the duodenum and jejunum, with little expression in the ileum or colon. Enterocytes prepared from the jejunum of CD36 knockout mice demonstrate reduced fatty acid uptake compared with wild-type controls. CD36 knockout mice, however, do not have significant fat malabsorption , although fat absorption is shifted to the more distal small intestine. Current observations indicate that CD36 mainly plays a regulatory role in intestinal triglyceride synthesis and chylomicron production (see below). CD36 is also expressed in lingual taste buds where is mediates perception of fatty acids, and may be involved in satiety signaling. FATP4 is high expressed in the small intestine, but is localized to the endoplasmic reticulum and subapical membranes. It, therefore, does not function as a true membrane transporter, but facilitates fatty acid uptake by virtue of its acyl CoA synthase activity. This results in metabolic trapping of fatty acids via conversion to fatty acyl CoA products that undergo rapid conversion to complex lipids, maintaining a concentration gradient for fatty acid uptake. Other candidate proteins involved in enterocyte fatty acid uptake (FABPpm, etc.) have been identified, but their role has not been completely elucidated. Further research is needed to define the relative contributions of diffusion and protein-mediated transport to enterocyte fatty acid uptake.
< div class='tao-gold-member'>
Only gold members can continue reading. Log In or Register a > to continue
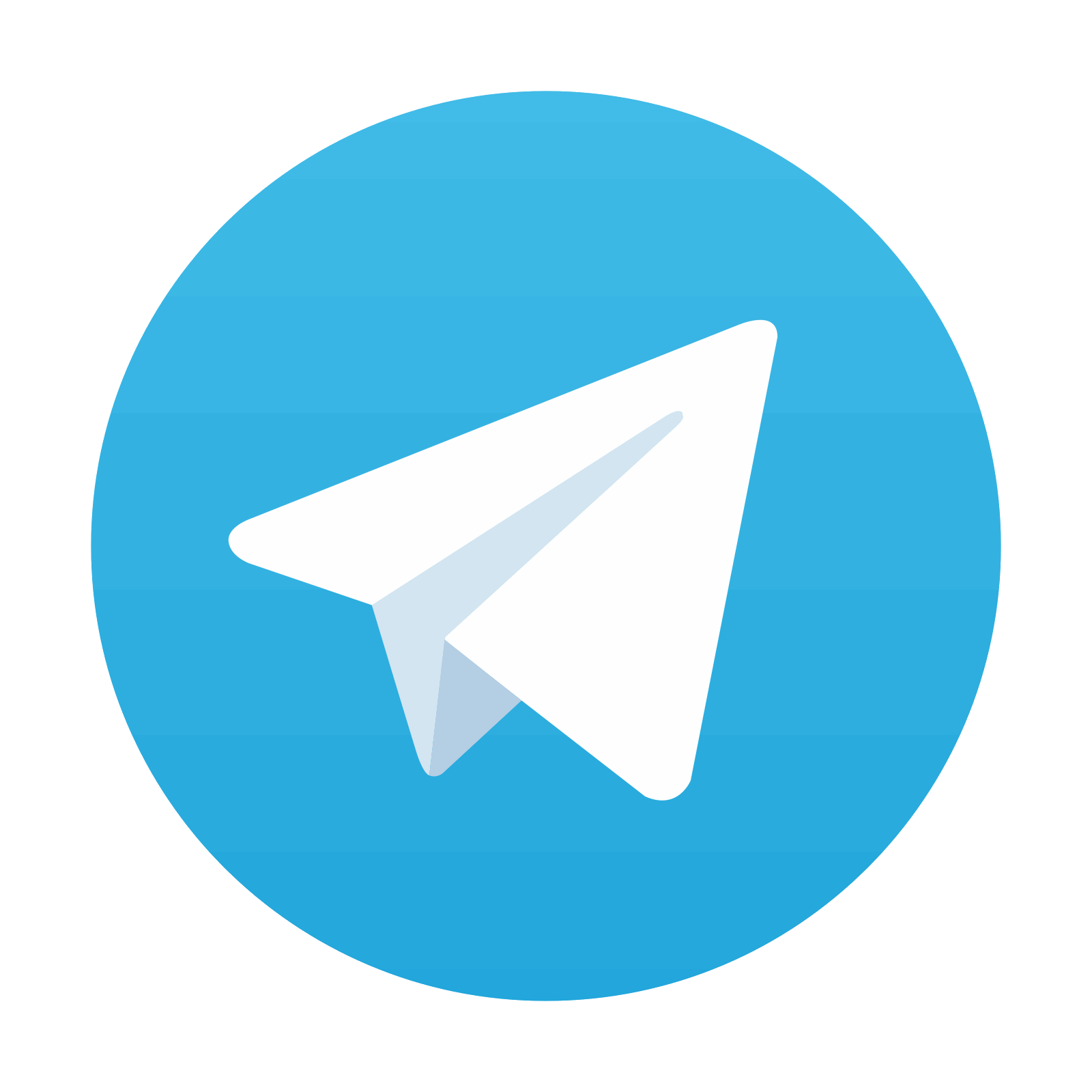
Stay updated, free articles. Join our Telegram channel

Full access? Get Clinical Tree
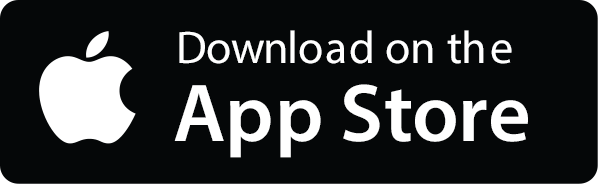
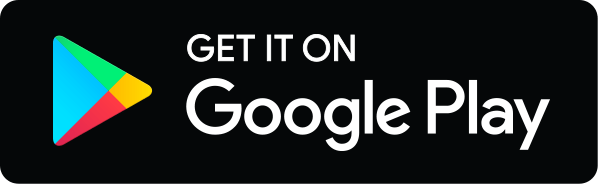