Diabetic Kidney Disease
Radica Z. Alicic
Joshua J. Neumiller
Katherine R. Tuttle
INTRODUCTION
Diabetes mellitus (DM), owing in part to the classic presenting symptom of polyuria, has long been associated with abnormalities in kidney function.1 Diabetic kidney disease (DKD), chronic kidney disease (CKD) caused by diabetes without other known causes, is defined as a sustained elevation of urinary albumin excretion (urine albumin-to-creatinine ratio [UACR] ≥30 mg/g) and/or a reduction in estimated glomerular filtration rate (eGFR) to less than 60 mL/min/1.73 m2.2 A diagnosis of DKD clearly increases the risk for progression to kidney failure, cardiovascular (CV) and cerebrovascular events, hospitalization, and death (Visual Abstract 10.1).3,4,5,6 Although less than 5% of cases of patients transitioning to kidney replacement therapy (KRT) were attributed to DM as recent as the 1970s, DKD is now the leading cause of kidney failure and the need for KRT, accounting for half of all cases worldwide.1,7 With the number of people in the United States living with DM continuing to rise at an unprecedented rate, the prevalence of DKD has increased in lockstep.8,9 The great majority (90%-95%) of more than 37 million Americans who are diagnosed with DM have type 2 diabetes mellitus (T2D).10 Approximately 40% of patients with T2D and 30% of those with type 1 diabetes mellitus (T1D) develop DKD.7,11,12 Efforts to improve the prevention, diagnosis, and effective management of DKD are critical, given the steadily increasing number of cases and resulting complications and mortality. This chapter will succinctly discuss the pathogenesis and clinical presentation of DKD, as well as current evidence-based recommendations for DKD prevention and treatment.
PATHOGENESIS OF DIABETIC KIDNEY DISEASE
The kidneys are central to glucose homeostasis. Under normal physiologic conditions, kidneys play a major role in gluconeogenesis as well as glucose reclamation from the glomerular filtrate.13 The majority (˜90%) of glucose reabsorption occurs in the early segment of the proximal tubule via sodium-glucose cotransporter-2 (SGLT2) and facilitative glucose transporter 2 (GLUT2). Only about 10% of reabsorption takes place in the S3 segment of the proximal tubule via sodium-glucose cotransporter-1 (SGLT1) and glucose transporter 1 (GLUT1) (see Figure 10.1).13,14,15
DKD results from the interaction of multiple, self-perpetuating processes activated by the metabolic abnormalities of diabetes. The pathophysiologic consequences of persistent hyperglycemia are numerous and include nonenzymatic modifications of proteins and lipids, formation of advanced glycation end products (AGE), and activation of various pathways linked to the pathogenesis of DKD (see Figure 10.2).6,16,17 Metabolic, hemodynamic, inflammatory, and fibrotic changes together result in cellular and structural abnormalities in all compartments of the kidney.
Increased Glucose Reabsorption in Kidney
In diabetes, glucose reabsorption in the proximal tubule is increased via enhanced reabsorption transport capacity of SGLT and GLUT transporters. This change is driven by augmented gene expression with translation to active proteins and helps reabsorb the increased amount of glucose present within the glomerular filtrate (Figure 10.1).18,19,20 Increased glucose reabsorption is an energy-consuming process, leading to greater oxygen demand in the cortex and medulla with subsequent expression of cellular stress markers such as neutrophil gelatinase-associated lipocalin (NGAL) and kidney injury molecule 1 (KIM1).21,22
An additional consequence of increased glucose and sodium uptake in the proximal tubule is a reduction of solute delivery to the macula densa. Reduced solute delivery to the macula densa is considered the primary contributor to the imbalance in afferent and efferent arteriole vascular tone and associated hemodynamic abnormalities (Figure 10.1).13,23
An additional consequence of increased glucose and sodium uptake in the proximal tubule is a reduction of solute delivery to the macula densa. Reduced solute delivery to the macula densa is considered the primary contributor to the imbalance in afferent and efferent arteriole vascular tone and associated hemodynamic abnormalities (Figure 10.1).13,23
Glomerular Hemodynamic Abnormalities in Diabetes
Glomerular hyperfiltration is an early hemodynamic change observed in approximately 75% of patients with T1D and at least 40% of those with T2D.24 The net effect of the imbalance in pre- and postglomerular vascular resistance (vasodilation of afferent arterioles and vasoconstriction of the efferent arterioles) is glomerular hypertension with increased glomerular capillary pressure and hyperfiltration.23,24,25 The exact mechanism(s) driving glomerular hyperfiltration remain under active investigation, but hyperglycemia along with high levels of insulin and amino acids are known to be important mediators.26 Afferent arteriolar vasodilation is driven by inhibition of tubuloglomerular feedback (TGF) (see Figure 10.1). TGF is an autoregulatory mechanism that adjusts the glomerular filtration rate in response to the concentration of solutes, sodium and chloride in particular, that are delivered to the distal convoluted tubule. In the diabetic kidney, increased glucose and sodium reabsorption in the proximal tubule reduces the delivery of solutes to the macula densa, a specialized segment of the distal convoluted tubule. Consequently, solute reabsorption via the Na-K-2Cl cotransporter (NKCC2) is decreased, resulting in diminished adenosine production and as a result, reduced adenosine-mediated vasoconstriction of the afferent arteriole. Another contributor to high glomerular capillary pressure is vasoconstriction of efferent arteriole. Vasoconstriction is mediated by increased local angiotensin II production, as well as the production of reactive oxygen species, and endothelin I (see Figure 10.1).13,24,25
Mechanotransduction
As discussed previously, hyperfiltration increases the glomerular capillary hydrostatic pressure gradient, thus exerting mechanical stress on structures of the kidney. Podocytes with their actin cytoskeleton are found to be mechanosensitive, and mechanical stretch causes narrowing of their bodies, elongation of cytoplasmic processes, and cellular hypertrophy.27 The stress-induced expansion of the glomerular basement membrane (GBM) contributes to adaptive podocyte hypertrophy and foot process effacement leading to ultimate podocyte detachment. Biomechanical stress activates various signaling pathways including protein kinases, prostaglandins, angiotensin II, mTOR, and TGF-β.28,29,30 Increased shear stress on proximal tubule microvilli is transmitted to the brush border cytoskeleton, generating biochemical signaling that activates the luminal Na+/H+ exchanger type 3 (NHE3) transporter and the basolateral Na+/3HCO3 cotransporter. Consequently, increases in reabsorption of sodium in the proximal tubule also contribute to decreased delivery of solutes to the macula densa and perpetuation of glomerular hyperfiltration.31,32
Inflammation and Fibrosis
Inflammation is now recognized as a major mechanism for the development and progression of DKD. Toll-like receptors (TLRs) play a fundamental role in the innate immune system by triggering proinflammatory signaling pathways in response to chronic exposure to the diabetic milieu.33 TLR2 and TLR4 receptors play a particularly important role, expressed in both intrinsic kidney cells (glomerular podocytes, mesangial cells, endothelial cells, and epithelial tubular cells) and immune cells (kidney dendritic cells, part of the resident network of inflammatory cells referred to as a renal mononuclear phagocyte [MNP], lymphocytes, and macrophages).34,35,36,37,38,39 Activation of TLRs upregulates transcription of proinflammatory cytokines and chemokines.37,38 Proinflammatory cytokines in
turn recruit additional immune cells, predominantly macrophages, activated T cells, and neutrophils, thus perpetuating a vicious inflammatory cycle.40,41,42,43,44
turn recruit additional immune cells, predominantly macrophages, activated T cells, and neutrophils, thus perpetuating a vicious inflammatory cycle.40,41,42,43,44
Inflammation produces various structural and resulting functional changes in the kidney. The extent of glomerulosclerosis and tubulointerstitial inflammation, as well as the rate of eGFR decline, is correlated with macrophage infiltration.42,45 Increased oxidative stress, inflammatory cell infiltration of the tubulointerstitium, intraglomerular hemodynamic abnormalities, extracellular matrix dynamics, promotion of kidney hypertrophy, thickening of the GBM, and podocyte and endothelial abnormalities are all associated with proinflammatory cytokine levels (including interleukin 1 [IL-1], interleukin 6 [IL-6], interleukin 18 [IL-18], and tumor necrosis factor-alpha [TNF-α]).46,47,48,49,50,51 Furthermore, macrophage infiltration of the tubulointerstitium, podocytopenia, and damage to GBMs and slit membranes are associated with upregulation of monocyte-chemotactic protein-1 (MCP-1)/chemokine C-C motif-ligand 2 (CCL2), C-X3-C motif chemokine (CX3CL1), and C-C motif chemokine 5.52,53,54,55
Epigenetic Changes in the Diabetic Kidney
Exposure to chronic hyperglycemia induces epigenetic alternations, including DNA methylation, histone modifications, and expression of noncoding RNAs.56,57 Epigenetic changes are a plausible mechanism for “metabolic memory” or the “legacy effect” characterized by persistent and enduring effects of early intensive glycemic control on the future development of DKD.33,58 Furthermore, histone methylation and acetylation have been implicated in the induction and expression of potent profibrotic factors in DKD such as TGF-β1.59,60 Noncoding RNAs are correlated with podocyte apoptosis, mesangial cell proliferation, and upregulation of various proinflammatory and fibrotic cytokines in animal models and in vitro.61,62,63,64
Structural Changes of Kidney
Structural changes in DKD are similar in both T1D and T2D across multiple compartments in the kidney.26,65,66,67 Enlargement of the kidney owing to glomerular and tubular hypertrophy, driven by growth factors and cytokines, is an early hallmark of DKD. Another early change is decreased negative charge and irregular thickening of the GBM.68 The proposed underlying mechanism of this change is increased production and decreased degradation of extracellular matrix proteins and heparan sulfate proteoglycan due to exposure to glycated proteins and mechanical stress in the glomerulus (Figure 10.3).6,68,69,70 Another key feature of early DKD is expansion of the mesangium.71 In part due to an adaptive response to the thickened GBM, alterations in subcellular localization of nephrin, and disruption of the actin cytoskeleton, podocytes flatten and demonstrate foot process effacement contributing to the loss of slit diaphragm integrity.71 Finally, podocytes detach from the GBM, leaving large subepithelial surfaces of the GBM denuded.72 Thickening of Bowman capsule and glomerular mesangial expansion are primarily due to increases in matrix and cellularity.73 Plasma proteins or hyaline material often accumulates between the glomerular endothelium and the GBM (fibrin cup) and between the glomerular tuft and Bowman capsule (capsular drop) (Figure 10.4).6,74,75 With the progression of DKD, mesangial expansion often forms sites of nodular glomerulosclerosis known as Kimmelstiel-Wilson nodules. Kimmelstiel-Wilson nodules were originally described as a pathognomonic lesion of diabetic glomerulopathy but may be seen in other conditions. Differential diagnosis includes membranoproliferative glomerulonephritis, monoclonal immunoglobulin/light chain deposition disease,
cryoglobulinemic glomerulonephritis, amyloidosis, fibronectin glomerulopathy, immunotactoid, fibrillary glomerulonephritis, and idiopathic nodular glomerulosclerosis (ING). ING resembles diabetic nephropathy in all aspects, except that patients are not diabetic. It is associated with long-standing hypertension or smoking (Figure 10.4).6,75,76,77 Sustained biomechanical stress on the tubular epithelium causes expansion of the tubular urinary space.31 The interstitium characteristically shows areas of fibrosis and inflammation, thickening of the tubular basement membrane, and tubular cell hypertrophy followed by tubular atrophy in late-stage DKD.78 The blood vessels display changes of arteriosclerosis and hyalinosis, particularly at the vascular pole. In advanced DKD stages, global glomerular sclerosis with severe interstitial fibrosis and tubular atrophy predominates (Figure 10.5).6,75 The international Renal Pathology Society work group developed a histopathological classification system to describe and grade severity of glomerular, interstitial, and vascular lesions of DKD.79
cryoglobulinemic glomerulonephritis, amyloidosis, fibronectin glomerulopathy, immunotactoid, fibrillary glomerulonephritis, and idiopathic nodular glomerulosclerosis (ING). ING resembles diabetic nephropathy in all aspects, except that patients are not diabetic. It is associated with long-standing hypertension or smoking (Figure 10.4).6,75,76,77 Sustained biomechanical stress on the tubular epithelium causes expansion of the tubular urinary space.31 The interstitium characteristically shows areas of fibrosis and inflammation, thickening of the tubular basement membrane, and tubular cell hypertrophy followed by tubular atrophy in late-stage DKD.78 The blood vessels display changes of arteriosclerosis and hyalinosis, particularly at the vascular pole. In advanced DKD stages, global glomerular sclerosis with severe interstitial fibrosis and tubular atrophy predominates (Figure 10.5).6,75 The international Renal Pathology Society work group developed a histopathological classification system to describe and grade severity of glomerular, interstitial, and vascular lesions of DKD.79
DIAGNOSIS AND ASSESSMENT OF DIABETIC KIDNEY DISEASE
The diagnosis of DKD is highly suggested by the presence of two abnormal measurements of albuminuria (UACR) ≥30 mg/g and/or a reduction in eGFR <60 mL/min/1.73 m2 at least 3 months apart, the presence of diabetic retinopathy, and the
absence of indicators of other causes of kidney disease.80,81 In T1D, the duration of diabetes is usually greater than 5 years before DKD onset, whereas DKD may be present at the time of T2D diagnosis. Evaluation for DKD should include a physical examination, family history, review of social and environmental factor exposure, and evaluation of medical history for presence of other systemic conditions with possible kidney involvement, including autoimmune disease (eg, systemic lupus erythematosus, vasculitis), chronic infections (eg, viral hepatitis, HIV), malignancies (eg, lymphoma, myeloma, solid tumors), frequent urinary tract infections, and episodes of acute kidney injury (AKI).2,81,82 A diagnostic kidney biopsy is performed infrequently, when there is clinical suspicion for an alternative diagnosis suggested by atypical features. Development of albuminuria greater than 5 years after diagnosis of T1D in the presence of diabetic retinopathy is strongly suggestive of DKD.11 Annual assessments of UACR and eGFR are recommended for all T1D patients starting 5 years following a diagnosis of diabetes and for all T2D patients beginning at the time of diagnosis.2,81 When used together, eGFR and UACR improve risk stratification and diagnostic accuracy (Figure 10.6).2,80,83,84 The American Diabetes Association (ADA) and the National Institute for Health and Care Excellence (NICE) from the United Kingdom recommend that those with a higher risk of diabetes and higher KDIGO risk categories for CKD should undergo assessment more frequently.78,81,83 Specifically, NICE recommends testing 2 to 4 times per year for individuals with UACR >30 mg/g and eGFR <45 mL/min/1.73 m2, whereas ADA recommends twice yearly monitoring for individuals with
UACR greater than or equal to 300 mg/g and/or eGFR 30 to 45 mL/min/1.73 m2 irrespective to therapy.81,83
absence of indicators of other causes of kidney disease.80,81 In T1D, the duration of diabetes is usually greater than 5 years before DKD onset, whereas DKD may be present at the time of T2D diagnosis. Evaluation for DKD should include a physical examination, family history, review of social and environmental factor exposure, and evaluation of medical history for presence of other systemic conditions with possible kidney involvement, including autoimmune disease (eg, systemic lupus erythematosus, vasculitis), chronic infections (eg, viral hepatitis, HIV), malignancies (eg, lymphoma, myeloma, solid tumors), frequent urinary tract infections, and episodes of acute kidney injury (AKI).2,81,82 A diagnostic kidney biopsy is performed infrequently, when there is clinical suspicion for an alternative diagnosis suggested by atypical features. Development of albuminuria greater than 5 years after diagnosis of T1D in the presence of diabetic retinopathy is strongly suggestive of DKD.11 Annual assessments of UACR and eGFR are recommended for all T1D patients starting 5 years following a diagnosis of diabetes and for all T2D patients beginning at the time of diagnosis.2,81 When used together, eGFR and UACR improve risk stratification and diagnostic accuracy (Figure 10.6).2,80,83,84 The American Diabetes Association (ADA) and the National Institute for Health and Care Excellence (NICE) from the United Kingdom recommend that those with a higher risk of diabetes and higher KDIGO risk categories for CKD should undergo assessment more frequently.78,81,83 Specifically, NICE recommends testing 2 to 4 times per year for individuals with UACR >30 mg/g and eGFR <45 mL/min/1.73 m2, whereas ADA recommends twice yearly monitoring for individuals with
UACR greater than or equal to 300 mg/g and/or eGFR 30 to 45 mL/min/1.73 m2 irrespective to therapy.81,83
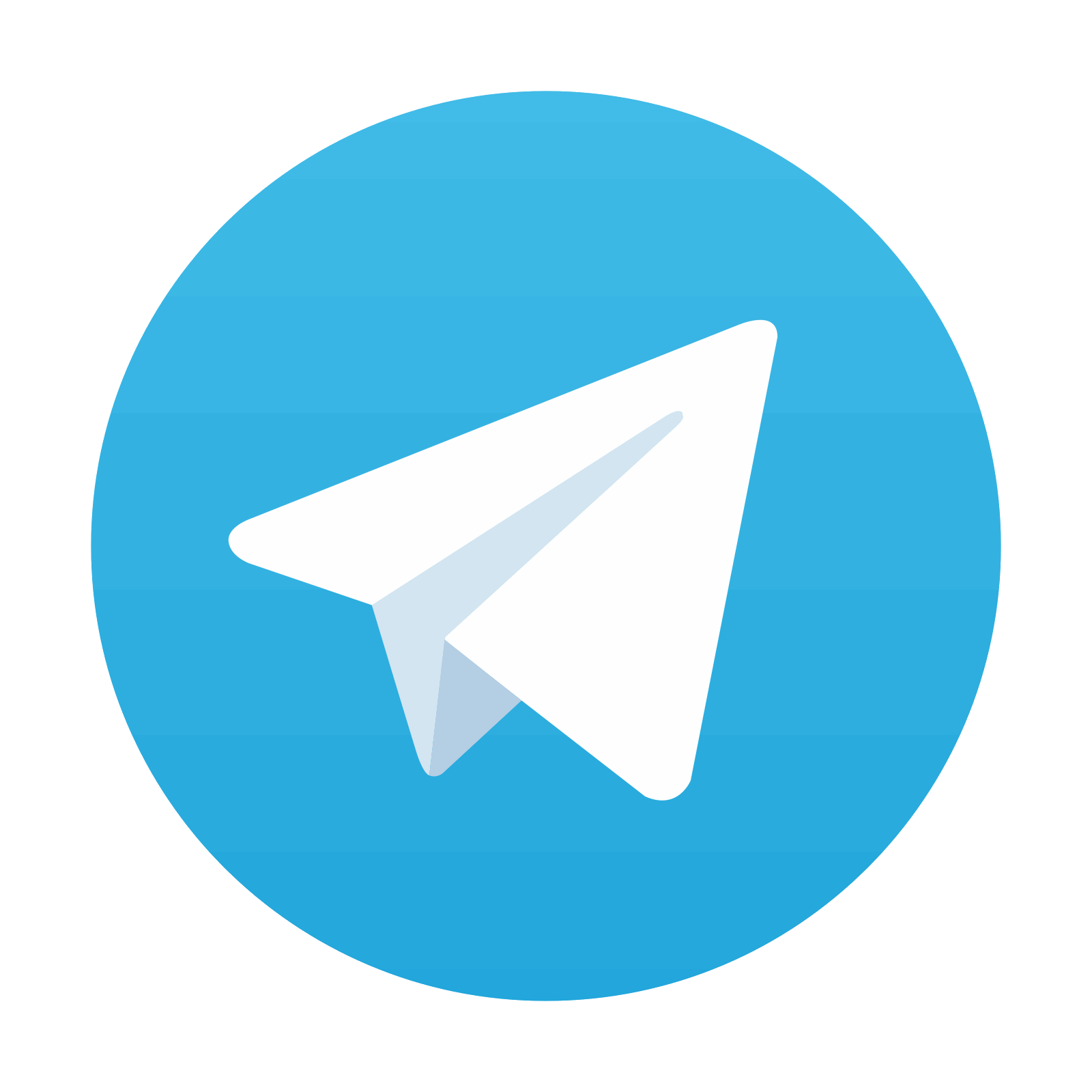
Stay updated, free articles. Join our Telegram channel

Full access? Get Clinical Tree
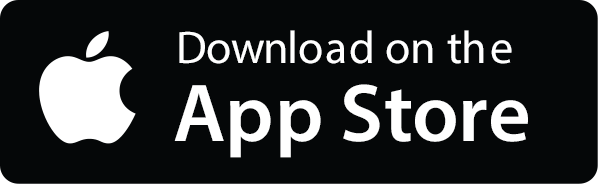
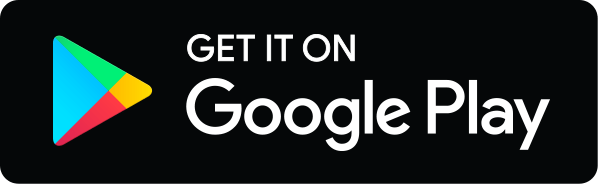