Abstract
Driven by the escalating prevalence of diabetes across the world, diabetic kidney disease (DKD) is an expanding global epidemic that incurs enormous human and economic cost. DKD is the most common cause of end-stage renal disease (ESRD) worldwide. It amplifies risk of other serious complications such as cardiovascular diseases and death. Many patients with DKD do not follow the classical paradigm of progressive albuminuria followed by declining kidney function. Currently, intensive glycemic control early in the course of types 1 and 2 diabetes produces a long-lasting preventive effect on DKD development. However, once DKD is established, intensive glycemic control increases the risk of hypoglycemia and has a nominal effect on DKD progression. Antihypertensive treatment with either an angiotensin-converting enzyme (ACE) inhibitor or an angiotensin II receptor blocker (ARB) reduces progression of established DKD to ESRD, but these agents do not prevent DKD onset. New approaches to improve DKD awareness, detection, and intervention are urgently needed to reduce its devastating complications.
Keywords
antihyperglycemic therapy, diabetic kidney disease, natural history, novel therapies, pathophysiological mechanism, structural changes
Outline
Introduction, 42
Diagnosis And Classification Of Diabetic Kidney Disease, 42
Risk Factors, 43
Natural History, 45
Kidney Structural Changes In Diabetic Kidney Disease, 46
Pathophysiological Mechanisms In DKD, 47
Current Treatment Of DKD, 51
New Therapies For Diabetic Kidney Disease, 55
Sodium–Glucose Cotransporter 2 Inhibitors, 57
Conclusions, 58
Acknowledgments
The authors would like to acknowledge Michele Rooney, MD, for creating and sharing histology images for Figs. 3.4, 3.5, and 3.8 .
Introduction
Diabetic kidney disease (DKD) is the leading cause of chronic kidney disease (CKD) worldwide and one of the most serious complications of diabetes. It is a major, yet underrecognized contributor to the global burden of chronic diseases. Despite many improvements in diabetes care over the past two decades, DKD still develops in approximately 30% of individuals with type 1 diabetes and nearly 50% of those with type 2 diabetes. Although the incidence of end-stage renal disease (ESRD) has in fact stabilized over the past decade, the increasing prevalence of diabetes due to obesity, aging, and reduction in cardiovascular disease–related deaths has greatly expanded the number of people living with diabetes. In 2015 there were an estimated 415 million cases of diabetes throughout the world; by 2040, this number is projected to increase to 642 million cases, of which 90% to 95% will be type 2 diabetes. These data clearly show that prevalence of DKD is growing at an unprecedented pace.
Development of DKD in people with diabetes amplifies the risk of cardiovascular diseases, infections, and cancers, as well as cardiovascular-related and all-cause mortality. Indeed, recent studies indicate that excess mortality associated with diabetes is mainly noted in those who developed kidney disease. Compared with the nondiabetic population, individuals with diabetes alone have a twofold higher risk of cardiovascular mortality, whereas individuals with both diabetes and kidney disease exhibit a threefold to twelvefold higher mortality risk.
Due to the frequent occurrence of death, only a minority of individuals with DKD survive to ESRD. Moreover, those with diabetes who survive to ESRD exhibit a 10- to 100-fold higher mortality risk than age-matched controls with normal kidney function. Due to the impact of DKD on diabetes-related complications and deaths, as well as the rising prevalence of diabetes, the global number of deaths attributed to DKD rose by 94% between 1990 and 2012. As the burden of DKD and diabetes-attributable ESRD grows, limited accessibility to renal replacement therapy (RRT) presents an increasingly emergent global healthcare challenge.
Diagnosis and Classification of Diabetic Kidney Disease
DKD is diagnosed by macroalbuminuria (albumin-to-creatinine ratio [ACR] >300 mg/g) or microalbuminuria (ACR 30 to 300 mg/g) associated with retinopathy (type 1 diabetes or type 2 diabetes), and in type 1 diabetes, more than 10 years’ duration of diabetes. Because a high glomerular filtration rate (GFR), or hyperfiltration, often occurs early after a diagnosis of diabetes, GFR <90 mL/min/1.73 m 2 may represent substantial loss of kidney function in DKD ( Table 3.1 ).
Albuminuria | ||||
---|---|---|---|---|
GFR (mL/min) | CKD Stage | Normoalbuminuria | Microalbuminuria | Macroalbuminuria |
>60 | 1 + 2 | At risk | Possible DKD | DKD |
30–60 | 3 | Possible DKD | Possible DKD | DKD |
<30 | 4 + 5 | Unlikely DKD | Unlikely DKD | DKD |
The preferred test for albuminuria is the urinary albumin-to-creatinine ratio (UACR) measured in a spot urine sample, preferably in the morning. Because UACR results are characterized by substantial day-to-day variability (up to 40%), abnormal results must be confirmed by at least two specimens collected over a 3- to 6-month period. In clinical practice, estimated GFR (eGFR) is usually calculated from serum creatinine concentration. Although the Modification of Diet in Renal Disease (MDRD) equation is commonly reported by clinical laboratories, the Chronic Kidney Disease-Epidemiologic Prognosis Initiative (CKD-EPI) equation is more accurate, particularly at eGFR levels in the normal or near-normal range.
S creening for DKD should be performed annually, starting 5 years after diagnosis of type 1 diabetes or at the time of type 2 diabetes diagnosis. The following features should prompt evaluation for non-DKD causes of kidney disease: absence of diabetic retinopathy, sudden onset of low eGFR, or rapidly decreasing eGFR; abrupt increase in albuminuria; development of nephrotic syndrome; presence of active urinary sediment; refractory hypertension; signs or symptoms of another systemic disease; and/or >30% decline in eGFR within 2 to 3 months of initiating treatment with a renin-angiotensin system (RAS) inhibitor.
Novel Biomarkers in Development
Current diagnostic methods are imprecise and lack adequate sensitivity for identifying patients with DKD. For example, many diabetic patients remain normoalbuminuric despite kidney structural damage that is severely advanced by the time eGFR declines. Testing for albuminuria and serum creatinine as primary diagnostic indicators also carries a number of limitations such as high biological and assay variability, lack of standardization, and low specificity. A number of new candidate biomarkers representing various molecular processes and disease pathways are under investigation for applications, including DKD diagnosis, risk stratification, monitoring, and prognosis ( Box 3.1 ). However, none are currently validated for clinical use.
Inflammation
Tumor necrosis factor alpha (TNF-α) – blood
Tumor necrosis factor receptor-1 (TNFR-1) – blood
Tumor necrosis factor receptor-2 (TNFR-2) – blood
Serum amyloid A (SAA) – blood and urine
Macrophage chemotactic protein-1 (MCP-1) – urine
Fibrosis
Connective tissue growth factor (CTGF) – urine
Transforming growth factor-β (TGF-β) – urine
Type IV collagen – urine
Angiogenesis
Vascular endothelial growth factor-A (VEGF-A) – blood
Endothelial Dysfunction
Amino terminal pro C-type natriuretic peptide (NT-proCNP) – blood
Mineral Metabolism
Fibroblast growth factor 23 (FGF-23) – blood
Tubular Injury
Neutrophil gelatinase associated lipocalin (NGAL) – urine
Kidney injury marker (KIM-1) – urine
Epidermal growth factor (EGF) – urine
Risk Factors
Demographic Factors
Several studies indicate that older age is an important predictor of progressive albuminuria and kidney dysfunction in diabetes. Male sex is a risk factor for kidney function decline in type 2 diabetes. Certain ethnicities also appear to exhibit greater susceptibility to, and/or a worse prognosis for, DKD. For instance, people of South Asian, African, American Indian, and Pacific Islander ancestry or Hispanic ethnicity are at higher risk of kidney function decline compared with people of European descent.
Alterations of Developmental Programming
Exposure to maternal diabetes during gestation increases the risk of DKD and high birth weight (≥4500 grams). Both low (<2500 grams) and high (≥4500 grams) birth weight are associated with an elevated risk of albuminuria in the offspring. For low-birth-weight infants, the proposed mechanism underlying increased risk of DKD is growth retardation, resulting in low nephron number and alterations of developmental programming that may predispose these infants to development of kidney disease later in life.
Hereditary Risk Factors
The fact that recognized risk factors fail to account for the variability of incident DKD suggests a genetic component underlying risk. Evidence for such inherited susceptibility to DKD comes from family studies that have shown increased risk of kidney disease in family groupings. The diabetic offspring of parents with diabetes and DKD have a cumulative risk of developing kidney disease of >70% and a threefold to fourfold increased incidence of kidney disease compared with children of diabetic parents without kidney disease. It appears that any inherited predisposition for developing DKD does not follow simple Mendelian rules. Genes that may be associated with DKD risk include those with functional networks related to the RAS, lipid metabolism, polyol pathways, fibrinogenesis, and kidney development; however, to date, no specific gene or gene combination has been definitively implicated in DKD risk.
Hyperglycemia
A relationship between elevated blood glucose and diabetic complications was observed as early as the 1930s, but five decades elapsed before an association between glycemic control and development of DKD was recognized. It is currently accepted that poor glycemic control independently predicts progression to development of albuminuria and ESRD in patients with type 1 diabetes and normoalbuminuria. Similarly, higher hemoglobin A1C (HbA1C) levels are among the strongest determinants of DKD progression independent of albuminuria in patients with type 2 diabetes.
Two landmark trials conducted in patients with either early-stage type 1 diabetes or type 2 diabetes found that early, intensive glycemic control reduced the long-term risk of DKD onset. The Diabetes Control and Complications Trial (DCCT) enrolled individuals with early, uncomplicated type 1 diabetes and compared effects of intensive versus conventional glycemic control. Time-averaged HbA1C during the 6-year study was 7.3% in the intensive therapy group and 9.1% in the conventional therapy group. The original DCCT participants were followed for another two decades in the Epidemiology of Diabetes Interventions and Complications (EDIC) study. Although the original differences in glucose control dissipated during the EDIC study, with HbA1C approximating 8% in both groups, an interim analysis including 9 years of EDIC data clearly showed that the intensive glycemic control group exhibited a 34% and 56% lower risk of developing microalbuminuria and macroalbuminuria, respectively. Pooled data from 22 years of follow-up showed that intensive glucose control was associated with about 50% lower risk of developing eGFR <60 mL/min/1.73 m 2 and ESRD, but the incidence of ESRD was small ( n = 8 participants in intensive care group, n = 16 participants in standard therapy group). The average rate of eGFR loss was significantly reduced from 1.56 mL/min/1.73 m 2 per year in the standard therapy group to 1.27 mL/min/1.73 m 2 per year in the intensive therapy group ( P < 0.001).
The United Kingdom Prospective Diabetes Study (UKPDS) enrolled individuals with newly diagnosed type 2 diabetes and compared the effect of conventional therapy to intensive therapy with either sulfonylurea or insulin or, in overweight patients, metformin. Between-group differences in glycemic control were lost 1 year into posttrial monitoring. Nevertheless, 10 years of posttrial follow-up data showed a 24% reduction in development of microvascular complications, including DKD, in the intensive therapy group compared with the conventional therapy group ( P = 0.001). After 12 years of posttrial follow-up, the intensive therapy group exhibited a 33% reduction in the risk of development of microalbuminuria or “clinical grade” proteinuria (macroalbuminuria) and a significant reduction in the proportion of patients with a doubling of blood creatinine level, relative to the conventional therapy group (0.9% vs. 3.5%, P = 0.0028).
In the wake of these studies, it was hypothesized that the observed beneficial effect of early strict glycemic control, termed the legacy effect or metabolic memory, could be explained by prevention of metabolism-driven epigenetic modifications. The mechanistic role of epigenetic alterations in DKD is supported by the observation that periods of uncontrolled hyperglycemia are associated with an elevated risk of developing DKD that does not reflect real-time HbA1C levels. Indeed, recent studies have indicated that greater glycemic variability, rather than real-time HbA1C levels, may better reflect the risk of DKD. The “legacy effect” may, at least in part, explain discrepant results from different observational studies evaluating the predictive value of HbA1C for DKD.
Hypertension
In patients with newly diagnosed type 2 diabetes followed for a median of 15 years, treatment to a target blood pressure of <150/85 mmHg compared with treatment to a target blood pressure <180/105 mmHg resulted in a significant reduction in overall risk of microvascular complications (37%, P = 0.009). Each 10 mmHg increase in mean systolic blood pressure was associated with a 13% ( P < 0.0001) increase in hazard ratio (HR) for development of both microalbuminuria and macroalbuminuria and impaired kidney function, defined as eGFR <60 mL/min/1.73 m 2 , or doubling of the blood creatinine level. A baseline systolic blood pressure >140 mmHg in patients with type 2 diabetes has also been shown to increase the risk for ESRD and death. In contrast to the legacy effects observed with intensive glycemic control, benefits of blood pressure control are transient and are lost if blood pressure rises again.
Obesity
The presence of obesity often precedes the diagnosis of diabetes by years or decades and exerts a continuously additive effect on the risk of DKD. The Verona Diabetes Study reported that obese participants with type 2 diabetes and preserved kidney function (baseline eGFR ≥60 mL/min/1.73 m 2 ) exhibited significantly faster age-adjusted annual eGFR decline during 10 years of follow-up (−1.2 ± 0.1 mL/min/1.73 m 2 /year) compared with normal-weight individuals. Although the specific mechanisms for obesity-induced kidney damage remain to be fully elucidated, a major insult appears to arise from glomerular hyperfiltration with resultant endothelial injury and activation of inflammatory pathways. For example, inflammatory adipokines and abnormalities of fatty acid metabolism may contribute to structural changes in the glomerulus leading to albuminuria. Obesity also exerts indirect effects by exacerbating other DKD risk factors, including hypertension, hyperglycemia, and insulin resistance.
Acute Kidney Injury
Several prospective and retrospective studies have identified a strong relationship between diabetes and incidence of acute kidney injury (AKI). This association is not age limited: a high proportion of children hospitalized with diabetic ketoacidosis developed AKI. Among 4000 diabetic patients followed from the years 1999 to 2008, a single episode of AKI was associated with over threefold increased risk for developing eGFR <30 mL/min/1.73 m 2 , independent of other major risk factors for DKD. This effect was dose dependent, such that each episode of AKI increased the risk of progression to advanced CKD stages.
Protein Intake
An elevation in circulating amino acids is an established inducer of hyperfiltration; consequently, the relationship of dietary protein intake to DKD prevention and progression has been under study for many years. Research in this area is limited by inherent difficulties associated with the conduct of nutritional studies, such as variability of diet composition and adherence to study diets. However, data from experimental studies suggest that long-term consumption of a lower-protein diet may reduce DKD progression via suppression of glomerular hyperfiltration, albuminuria, and inflammation.
In patients with type 2 diabetes, high protein intake is associated with development of albuminuria. Likewise, in patients with type 1 diabetes, reduced protein intake (0.89 g/kg/day) versus usual protein intake (1.02 g/kg/day) is associated with fewer cases of ESRD or death (10% in the reduced-protein diet group vs. 27% of patients on the usual-protein diet group, P = 0.042). However, presumably due to differences in diet composition, adherence, and study duration, other studies and metaanalyses have not consistently reported positive results. .
Risk factors contributing to the susceptibility, initiation, or progression of DKD are detailed in Table 3.2 .
Risk Factor | Susceptibility | Initiation | Progression |
---|---|---|---|
Demographics | |||
Older age | + | ||
Sex (male) | + | ||
Race/ethnicity (African American, American Indian, Hispanic, Asian/Pacific Islanders) | + | + | |
Development | |||
Low birth weight/high birth weight | + | + | |
Exposure to maternal diabetes | + | + | |
Heredity | |||
Family history of diabetic kidney disease | + | ||
Systemic Conditions | |||
Hyperglycemia | + | + | + |
Hypertension | + | + | |
Obesity | + | + | + |
Kidney Injuries | |||
Acute kidney injury | + | + | |
Smoking | + | + | |
Dietary Factors | |||
High protein intake | + | + |
Natural History
Progressive albuminuria followed by decline in GFR is the classical paradigm of DKD presentation. However, accumulating knowledge challenges this concept.
The UKPDS offered a unique opportunity to observe the natural history of DKD in patients with type 2 diabetes from early in the course of the disease. Approximately 2% of study participants per year progressed from normoalbuminuria to microalbuminuria and from microalbuminuria to macroalbuminuria. At a median of 15 years after diagnosis, 40% of UKPDS participants developed albuminuria and 30% developed either an eGFR <60 mL/min/1.73 m 2 or a doubling of the blood creatinine level. These data raised awareness that albuminuria and declining eGFR do not always coincide: 60% of individuals developing kidney functional impairment did not have preceding albuminuria, and 40% of study participants never developed albuminuria.
The Developing Education on Microalbuminuria for Awareness of Renal and Cardiovascular Risk in Diabetes (DEMAND) study confirmed that albuminuria and declining kidney function do not co-occur in a significant number of patients. Overall, 21% of individuals with normoalbuminuria, 31% of individuals with microalbuminuria, and 35% of individuals with macroalbuminuria developed a creatinine clearance of <60 mL/min. In a different study, about 20% of individuals with type 2 diabetes had eGFR <60 mL/min/1.73 m 2 and about 50% had albuminuria, with presentation varying across different races and ethnicities.
The classical paradigm of DKD is further complicated by recent data indicating that albuminuria is a dynamic, fluctuating condition rather than a linearly progressive process. For example, during nearly 8 years of follow-up in the Multifactorial Intervention for Patients with Type 2 Diabetes Study (Steno-2), 31% of participants with microalbuminuria progressed to macroalbuminuria, whereas 31% regressed to normoalbuminuria. Another 38% remained microalbuminuric during this period.
Over time, the clinical presentation of DKD has changed. In a representative US population, the prevalence of albuminuria alone as a clinical presentation decreased from 21% (1988 to 1994) to 16% (2009 to 2014). Across the same time span, the prevalence of low eGFR (<60 mL/min/1.73 m 2 ) increased from 9% to 14%, and severely reduced GFR (<30 mL/min/1.73 m 2 ) increased from 1% to 3%. Data from large clinical trial databases have also revealed different patterns of clinical DKD presentations associated with type 1 diabetes and type 2 diabetes. In data from over 20,000 patients, those with type 1 diabetes showed a lower frequency of eGFR <60 mL/min/1.73 m 2 and albuminuria compared with type 2 diabetes after a mean of 19 years. Frequencies of low eGFR and albuminuria were 8% and 19% in type 1 diabetes and type 2 diabetes, respectively. Factors underlying different presentations of DKD in type 2 diabetes may include longer exposure to hyperglycemia before diagnosis, older age, a higher burden of comorbidities, and other types of kidney diseases.
The practice of diagnosing DKD based on clinical presentation alone has been challenged by recent reports of kidney histology. Autopsies of diabetic individuals found a considerably higher prevalence of histologically diagnosed DKD compared with that shown by clinical laboratory testing. Of 168 patients with type 1 and type 2 diabetes, 106 (63%) exhibited histopathological changes characteristic of DKD. However, albuminuria and low eGFR were absent in 20% (20/106) of these patients throughout their lifetime. Structural changes were highly variable and encompassed a variety of histopathological classes of DKD.
CKD complications develop relatively early in DKD ( Fig. 3.1 ). There are several possible explanations why anemia and bone and mineral metabolism disorders manifest earlier in DKD than in other types of CKD. Predominant tubulointerstitial fibrosis associated with damage to the peritubular interstitial cells that produce erythropoietin is frequently seen in DKD. As a result, patients with diabetes are prone to erythropoietin deficiency and are nearly twice as likely to have anemia compared with patients with nondiabetic CKD and comparable eGFR. DKD is associated with lower parathyroid hormone levels compared with other types of CKD, probably because insulin is a cofactor for parathyroid hormone release. Lower parathyroid hormone levels may predispose DKD patients to adynamic bone disease.

Deaths due to cardiovascular diseases and infections are highly prevalent and compete with progression to ESRD. The UKPDS study showed that the overall death rate after onset of DKD among patients with blood creatinine levels >2 mg/dL or among those receiving kidney replacement therapy was nearly 20% per year 82 . More recent data show that the annual mortality rate in individuals with DKD was 64.1 per 1000 individuals (95% confidence interval [CI], 60.2 to 68.3) The mortality rate sharply increased with severity of kidney disease and ranged from 37 per 1000 individuals in stage 3A, 58 per 1000 individuals in stage 3B, 98 per 1000 individuals with stage 4, and 199 per 1000 individuals with stage 5 DKD.
Kidney Structural Changes in Diabetic Kidney Disease
Diagnosis of DKD is made based on a constellation of histological findings, with no single histological change being characteristic of DKD ( Fig. 3.2 ). Structural changes in both types of diabetes are similar, but changes in type 2 diabetes are more heterogeneous and less predictably associated with clinical presentations. Knowledge of structural kidney changes in patients with types 1 and 2 diabetes is derived mostly from clinical biopsies, and is therefore limited by selection bias, because most who undergo a biopsy have atypical presentations. Selection bias for kidney biopsy contributes to a high prevalence of nondiabetic kidney disease by histology, especially in type 2 diabetes. However, recent clinical biopsy studies estimate prevalence of nondiabetic kidney disease in patients with diabetes and albuminuria to be about 10%.

An early histological finding in DKD is thickening of the glomerular basement membrane, which is typically apparent 1.5 to 2 years after type 1 diabetes diagnosis. It is paralleled by capillary and tubular basement membrane thickening. Other glomerular changes include loss of endothelial fenestrations, mesangial matrix expansion, and loss of podocytes with effacement of foot processes ( Fig. 3.3 ). Mesangial volume expansion is detectable within 5 to 7 years of type 1 diabetes onset. Later, segmental mesangiolysis with disruption of capillary tufts gives rise to microaneurysms. Classical Kimmelstiel–Wilson nodules and microaneurysms often present together.

With progression, efferent arterioles as well as capillary loops become hyalinized. The exudative lesions result from subendothelial deposits of plasma proteins, which form periodic-acid–Schiff-positive and electron-dense deposits and accumulate in small arterial branches, arterioles, and glomerular capillaries, as well as microaneurysms. These deposits result in luminal compromise (e.g., hyaline arteriosclerosis). Similar subepithelial deposits are seen in Bowman capsule (“capsular drop lesion”) and proximal renal tubules. In later stages of DKD, there is coalescence into segmental and global sclerosis ( Figs. 3.4 and 3.5 ). An international consensus working group has provided a pathological classification system to address the heterogeneity of DKD presentation ( Tables 3.3 and 3.4 ).


Type of Lesion | Criterion | Score |
---|---|---|
Interstitial fibrosis and tubular atrophy (IFTA) | Absent | 0 |
<25% | 1 | |
25%–50% | 2 | |
>50% | 3 | |
Interstitial inflammation | Absent | 0 |
Infiltration only in relation to IFTA | 1 | |
Infiltration in areas without IFTA | 2 | |
Vascular lesions arteriolar hyalinosis | Absent | 0 |
At least one area of arteriolar hyalinosis | 1 | |
More than one area of arteriolar hyalinosis | 2 | |
Presence of large vessel arteriosclerosis | No intimal thickening | 0 |
Intimal thickening less than thickness of media | 1 | |
Intimal thickening greater that thickness of media | 2 |
Class | Description | Inclusion Criteria |
---|---|---|
I | Mild or nonspecific light microscopy changes and electron microscopy–proven GBM thickening | GBM >395 nm in female and >430 nm in male individuals 9 years of age and older |
Biopsy does not meet any of the criteria mentioned later for class II, III, or IV | ||
IIa | Mesangial expansion, mild | Mild mesangial expansion in >25% of the observed mesangium |
Biopsy does not meet criteria for class III or IV | ||
IIb | Mesangial expansion, severe | Severe mesangial expansion in >25% of the observed mesangium |
Biopsy does not meet criteria for class III or IV | ||
III | Nodular sclerosis (Kimmelstiel–Wilson lesion) | At least one convincing Kimmelstiel–Wilson lesion |
Biopsy does not meet criteria for class IV | ||
IV | Advanced diabetic glomerulosclerosis | Global glomerular sclerosis in >50% of glomeruli |
Lesions from classes I through III |
Pathophysiological Mechanisms in DKD
Pathophysiology of DKD is a complex and dynamic process. It involves multidirectional and self-perpetuating signaling pathways, with crosstalk between various mediators regulating initiation and progression of the disease.
Glomerular hyperfiltration is an early hemodynamic change in DKD. It occurs in up to 75% of patients with early type 1 diabetes. Among patients with type 2 diabetes, prevalence of glomerular hyperfiltration is approximately 40%. Although mechanisms underlying glomerular hyperfiltration in diabetes are incompletely understood, one plausible mechanism is increased proximal tubular reabsorption of glucose via sodium–glucose cotransporter 2 (SGLT-2), which decreases distal delivery of solutes, particularly sodium chloride, to the macula densa. The resulting decrease in tubuloglomerular feedback dilates the afferent arteriole to increase glomerular perfusion, while concurrently, high local production of angiotensin II at the efferent arteriole produces vasoconstriction. These mechanisms operating in tandem produce high intraglomerular pressure and glomerular hyperfiltration. In type 2 diabetes, systemic hypertension and obesity also contribute to glomerular hyperfiltration via mechanisms such as high transmitted systemic blood pressure and glomerular enlargement ( Fig. 3.6 ).

The inability of the endothelial cells to downregulate glucose transport in response to hyperglycemia results in excessive intracellular glucose flux, leading to mitochondrial generation of reactive oxygen species (ROS), formation of advanced glycation products (AGE), increased expression of receptors for advanced glycation products (RAGE), and activation of various protein kinase C (PKC) isoforms ( Fig. 3.7 ). Recent proteomic studies indicate that activation of inflammatory pathways antedates development of DKD, and inflammatory markers are detected in the urine of patients with early diabetes. It is now believed that ongoing activity of inflammatory and fibrotic molecules and pathways underlies the structural and functional changes observed in DKD ( Figs. 3.8 and 3.9 ).



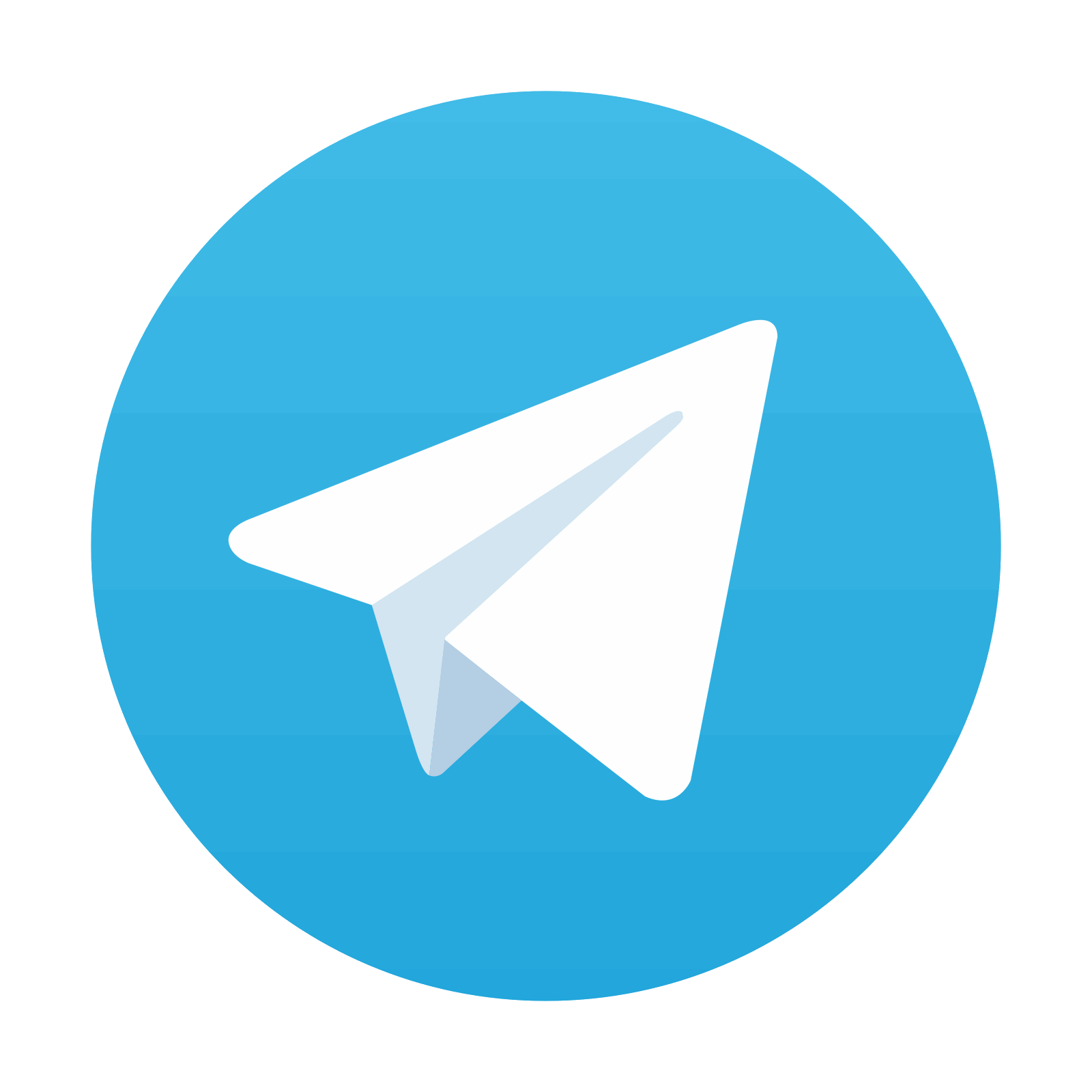
Stay updated, free articles. Join our Telegram channel

Full access? Get Clinical Tree
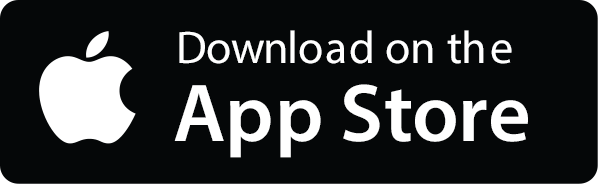
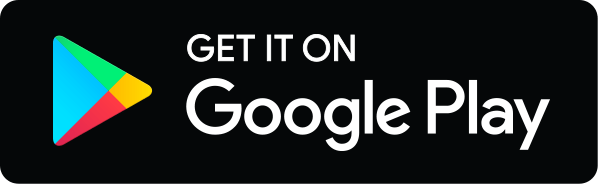
