Chapter 4.6
Decompensated liver disease and nutrition
Simran Arora1, Gillian Gatiss2, Laura M. McGeeney2 and Nina C. Powell2
1Royal Free London NHS Foundation Trust, London, UK
2Cambridge University Hospitals NHS Foundation Trust, Cambridge, UK
4.6.1 Dietary causes and effects of decompensated liver disease
Liver disease can be caused by various factors as shown in Table 4.6.1. Largely it is the symptoms and severity of the disease that determine the nutritional treatment, rather than the aetiology.
Table 4.6.1 Types of liver disease
Cause | Type of liver disease |
Infections | Hepatitis A, hepatitis B, hepatitis C, hepatitis D, hepatitis E |
Toxic | Alcohol-related liver disease, drug overdoses, other poisons |
Cholestatic | Primary sclerosing cholangitis, primary biliary cirrhosis |
Metabolic | Non-alcoholic fatty liver disease, non-alcoholic steatohepatitis, hereditary haemochromatosis, alpha-1 antitrypsin deficiency, Wilson’s disease |
Vascular | Budd–Chiari syndrome |
Other | Cystic fibrosis-related liver disease, autoimmune hepatitis, cryptogenic liver disease |
Due to the vital metabolic role of the liver, loss of function has significant nutritional consequences. The reported incidence of undernutrition in this population varies with assessment technique and stage of disease; it can be as high as 100% [1–3]. Malnutrition affects important clinical outcomes including rates of variceal bleeding, encephalopathy, infections, ascites, poor muscle function, length of hospital stay and mortality rates [4–7]. The level of protein-energy malnutrition (PEM) is dependent on the severity of the liver disease and level of nutritional input into patient care rather than the aetiology.
Factors contributing to PEM include a reduced oral intake due to nausea, vomiting, pain, encephalopathy, early satiety (which may be due to the pressure of ascites), alcohol withdrawal, periods of being nil by mouth for tests, anorexia, nutrient and fluid restrictions, unpalatable diets (such as very low-salt diets), altered sense of taste and fatigue. Patients with PEM may also have malabsorption in the form of diarrhoea or steatorrhoea due to cholestasis, the use of regular lactulose or changes to GI microbiota following antibiotics, and may have increased requirements for energy and protein due to changes in energy metabolism.
Metabolic changes in cirrhosis
Figure 4.6.1 shows the major metabolic changes that occur in a person with cirrhosis and the connections between them.
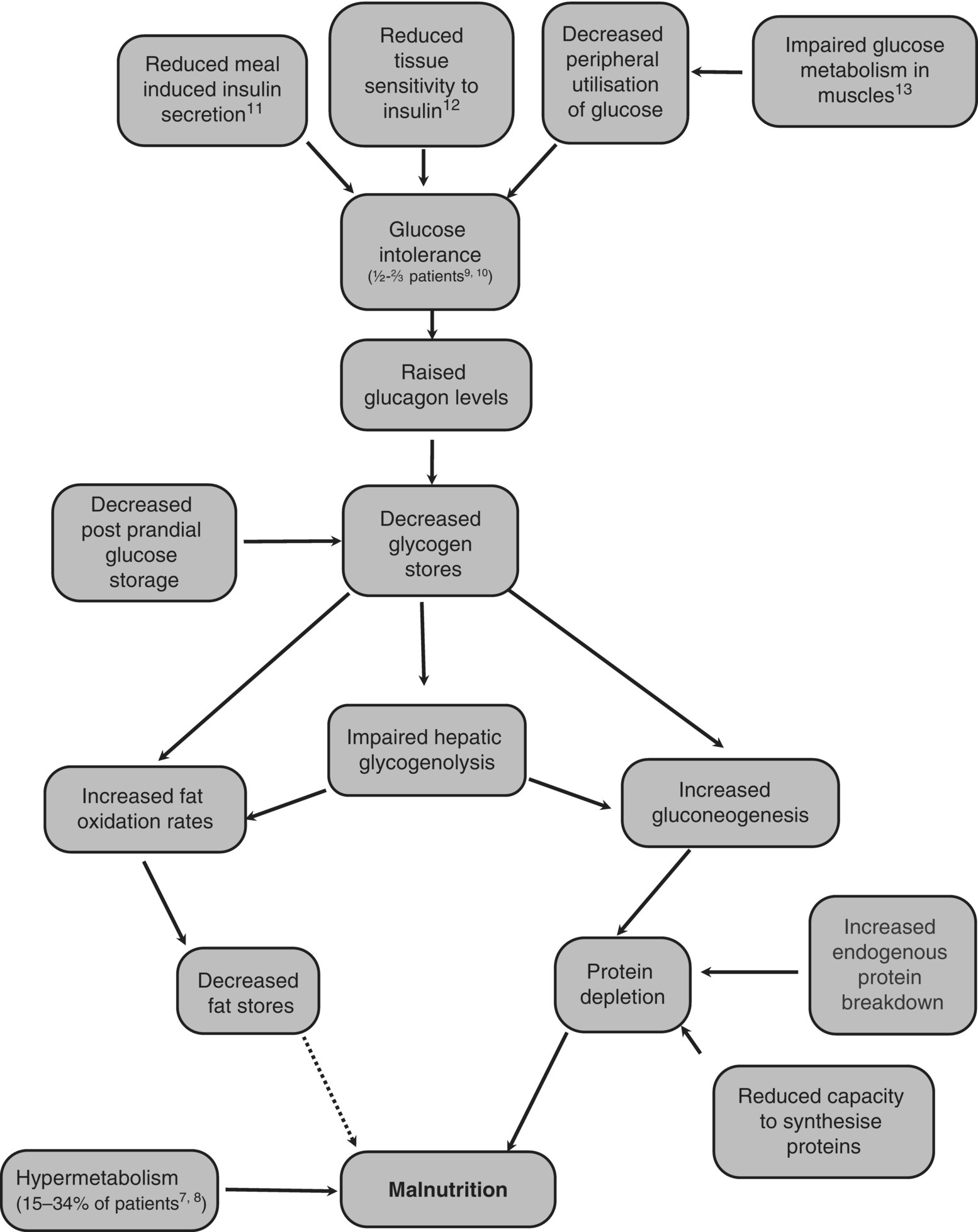
Figure 4.6.1 The major metabolic changes that occur in someone with cirrhosis [7–13].
Malnutrition in liver disease has been called a ‘glycogen storage disease’ as even in the fed state, glycogen stores remain low [14]. Hepatic glycogenolysis is also impaired, making glycogen a less suitable fuel for cirrhotic patients. To maintain blood glucose concentrations in the short term when there has been no recent intake of carbohydrate, there is an increase in gluconeogenesis from amino acids, resulting in protein depletion [15]. Petersen et al. compared gluconeogenesis and glycogenolysis rates in cirrhotic subjects and matched healthy controls using nuclear magnetic resonance spectroscopy and 2H2O and found that they had similar rates of glucose production but the cirrhotic subjects had an increased rate of gluconeogenesis and decreased rate of glycogenolysis compared to the control subjects [16]. Fat is also used as an alternative fuel source [17]. However, the fat oxidation rates do return to normal after refeeding [18].
Using indirect calorimetry, the percentage of total energy from fat, carbohydrate and protein in control subjects after a 36–72-h fast were comparable to those found in cirrhotic patients after an overnight fast (10–12 h) [19]. These proportions were significantly different from overnight fasted control subjects. This indicates that cirrhotic patients reach a fasted state much more rapidly than healthy controls; cirrhosis has been termed a disease of accelerated starvation. Increased endogenous protein breakdown leads to increased protein turnover. However, the protein resynthesis rate does not increase and the capacity of the cirrhotic liver to synthesise and store proteins is reduced, leading to muscle wasting. The mechanism of this hypermetabolism is unclear but is thought to be extrahepatic as it persists for over a year following liver transplantation [20].
Not all cirrhotic patients are hypermetabolic; those that are have a similar percentage of muscle mass as normometabolic cirrhotic patients, but a reduced body cell mass [8]. They also have raised serum cytokines, indicating a potential role for the inflammatory response in the hypermetabolic state. Greco et al. showed hypermetabolism, increased lipid oxidation rates and insulin resistance after an overnight fast in Childs B cirrhotic patients when compared to matched healthy controls [20a]. The Body Mass Index (BMI), fat free mass and fat mass of the patients were not statistically significantly different from the healthy controls, suggesting that metabolic changes precede weight loss rather than being a consequence of it.
Muscle wasting can be a major feature of cirrhosis but with skilled nutritional assessment and therapy this can be prevented.
Nutritional assessment
Undernutrition can be present in the early stages of liver disease but is not always readily evident, especially in people who are overweight. Detailed nutritional assessment is needed to identify people who are undernourished or at risk of becoming so. Accurate assessment of nutritional status can be particularly difficult in patients with cirrhosis due to altered fluid homeostasis. Traditional nutritional assessment methods and nutrition screening tools are often based on weight and BMI changes but more specific measures of nutritional status are required for this patient group.
Nutritional assessment would include a review of symptoms, medical history, social history, assessment of body composition, biochemistry, detailed dietary intake and fluid restrictions. Symptoms such as nausea, anorexia, pain, fatigue and encephalopathy can reduce nutritional intake, as can any fluid restrictions. While constipation can lead people to be more likely to experience encephalopathy, diarrhoea reduces the absorption of nutrients and can also lead people to be less inclined to eat. Medical history including co-morbidities such as renal failure, hepatopulmonary syndrome or diabetes will potentially have an impact on patient nutritional status as well as the dietary counselling given. A person’s social situation including financial situation, living conditions, alcohol and illicit drug use will have an impact on their nutritional status. Changes in a patient’s liver function tests can give an indication of an improvement or decline in their condition; it is important to monitor electrolytes in potential refeeding syndrome patients and biochemistry can also be used to monitor conditions such as renal failure.
When taking a diet history, it is particularly important to note the pattern of nutritional intake as well as the nutritional content and portion sizes, looking out for the frequency and amount of carbohydrate and protein consumed in particular. As with any other patient group, it is important to take into account patient preferences and tolerances, daily pattern of activities and any concerns they have.
Upper arm anthropometry
Upper arm anthropometry, mid arm circumference (MAC), triceps skinfold thickness (TST) and mid arm muscle circumference (MAMC) are used in the clinic or ward setting to differentiate between fat and muscle stores. These measurements indicate a patient’s nutritional status compared to an expected population. Serial measurements in one patient every 3–8 weeks are most useful as body fat has to change by several kg before it is detectable by skinfold measurements [21]. It is important to be aware of inter- and intraobserver error. Reliability and accuracy can be improved by standardising the observer’s technique or by undertaking specialist anthropometry training.
Hand grip strength
Hand grip strength is measured using a dynamometer and is a measure of muscle function. Low handgrip strength has been shown to be a predictor of complications and poor clinical outcome in patients with cirrhosis [22]. Patients’ handgrip strength changes more rapidly than the measured change in muscle bulk and can be assessed weekly if required.
Nutrition screening
Tools that incorporate information from several sources to a patient in a structured way can be particularly helpful. The Royal Free Hospital Global Assessment (RFH-GA) which uses BMI (calculated using estimated dry body weight), MAMC, dietary intake and symptoms in an algorithm [23] has very good intra- and interobserver reproducibility and is validated in this patient group [24]. Accurate use of this assessment requires specialist training and can take an hour to complete. The Royal Free Hospital Nutritional Prioritising Tool (RFH-NPT) has recently been developed and validated. This has very good intra- and interobserver reproducibility, with a diagnostic sensitivity of 100% and specificity of 73% [25]. It takes 2–3 min and little training to complete but gives a less detailed assessment than the RFH-GA. This tool includes the presence/absence of ascites and/or oedema with different subsequent questions depending on this answer.
Dry weight
Table 4.6.2 can be used to estimate the weight of fluid and therefore to calculate dry weight. The estimated weight of ascites and peripheral oedema is subtracted from the patient’s ‘wet weight’. Patients may have ascitic volumes larger than 14 kg. Even in patients with no ascites or oedema, weight alone is a crude measure of nutritional status as it does not distinguish between fat and muscle stores. For patients with ascites who have regular paracentesis, it is useful to monitor how much fluid is removed during each paracentesis session, how much fluid is remaining after paracentesis, a typical postparacentesis weight and the duration since the last paracentesis, to individualise estimates of dry body weight.
Table 4.6.2 An estimate of the weight of ascites and peripheral oedema [26]
Ascites | Peripheral oedema | |
Minimal | 2.2 kg | 1.0 kg |
Moderate | 6.0 kg | 5.0 kg |
Severe | 14.0 kg | 10.0 kg |
Reproduced with permission from Taylor & Francis.
Nutritional requirements
When gluconeogenesis rather than glycogenolysis is used to maintain hepatic glucose release there is an increase in both resting energy expenditure (REE) and protein requirements [19,27]. Gluconeogenesis uses more energy than glycogenolysis and requires amino acids as substrates, therefore both energy and protein requirements for cirrhotics are increased. People with liver disease can replete their stores with increased intakes [28,29]. The European Society of Parenteral and Enteral Nutrition (ESPEN) guidelines aim for 25–40 kcal/kg body weight but whether dry, actual or ideal body weight is used is not agreed (Table 4.6.3) [30]. If a patient is undernourished, unless they are overtly critically ill or septic, the addition of an extra 400–1000 kcal per day is encouraged to promote repletion of stores.
Table 4.6.3 Energy and protein requirements for people with liver disease
Stress factora | Energy kcal/kg dBW/day | Protein g/kg dBW/day | |
Compensated | 0–20% | 25–35 | 1.2–1.3 |
Decompensated | 30–40% | 35–40 | 1.2–1.5 |
Acute (fulimant) | 20–30% | 1.2–1.5 | |
Post transplant (approx. 1 month) | 30% | 1.2–1.5 |
In the UK, energy (for weight maintenance) and protein requirements use dBW (dry body weight) [30,31].
aA stress factor is used to adjust for additional metabolic stress and multiplied by basal metabolic rate or by the specific kcal/kg for each condition by body weight [30].
Reproduced with permission from Elsevier.
Protein stores of lean body mass (LBM) are depleted by the use of amino acids for glucose generation, therefore extra dietary protein is required to maintain and replete LBM. Swart et al. used radiolabelled [15 N]glycine to investigate rates of nitrogen flux, protein synthesis and protein breakdown in people with cirrhosis and healthy controls and suggested that cirrhotic patients require an increase in nitrogen intake to achieve a positive nitrogen balance due to their increase in gluconeogenesis [29]. Protein requirements are calculated based on the patient’s estimated dry weight and are shown in Table 4.6.3.
Patients, particularly if at home and mobile, may need to increase their intake beyond these calculated requirements in order to replete or maintain their muscle stores. It is important to monitor their nutritional status regularly and adjust their dietary recommendations accordingly.
In obese patients, protein and energy requirements are adjusted to account for increased body mass with a likely reduced percentage of metabolically active tissue. In the UK, protein and energy requirements are reduced by 25% and 400–1000 kcal per day respectively. When making any such adjustments, it is important that patients are regularly monitored so any decline in nutritional status can be identified and mitigated. Preservation of muscle function and a patient’s fitness take priority over any aim to reduce fat mass. ESPEN guidelines do not give guidance specifically for obese liver patients.
Fluid requirements are rarely estimated from predetermined calculations but assessed on an individual basis and dependent on the patient’s fluid status.
4.6.2 Dietary causes of hepatic encephalopathy
The cause of hepatic encephalopathy (HE) is likely to be multifactorial and is still not fully understood. Consequently dietary manipulation in HE has evolved as a greater level of evidence supports or disproves theories. One of the first theories about the cause was that dietary protein causes HE in liver failure. Hence, historically dietary protein restriction was advised to reduce the risk and facilitate the management of HE [32]. This is no longer advised as protein turnover studies have demonstrated that the requirement for protein and the overall energy need increase with the development of end-stage liver failure [3,33]. Restricting intake can lead to muscle catabolism and the associated poor outcomes in these patients [34].
It is thought that the need for additional dietary protein is driven by the utilisation of branched chain amino acids (BCAA) for the repair of the liver injury or utilisation of them to dispose of ammonia [35], lowering the ratio of the BCAA relative to the aromatic amino acids. Furthermore, if the additional calorie requirements are not met, the breakdown of muscle protein increases the circulating concentration of aromatic amino acids (phenylalanine, tyrosine) relative to the circulating concentrations of BCAA (leucine, isoleucine, valine). Ultimately a higher ratio of aromatic amino acids to BCAA facilitates the movement of aromatic amino acids across the blood–brain barrier, altering the mental state associated with HE. This theory is further supported by the development of HE following a gastrointestinal bleed. It is proposed that the digestion of blood, which is deficient in isoleucine, a branched chain amino acid, alters the ratio of aromatic amino acids to BCAA, causing acute HE [36].
A high concentration of gastrointestinal ammonia is another theory for the cause of HE. During periods of fasting, the utilisation of amino acids from muscle for gluconeogenesis produces ammonia. Circulating ammonia is removed by muscle tissue, hence muscle loss in itself may exacerbate the development of HE [37]. Dietary factors that can alter the gastrointestinal microbiota and reduce the high concentrations of ammonia production are thought to reduce the risk and facilitate the management of HE. Dietary factors that have been studied in this process include lactulose, probiotics, vegetable protein and zinc.
4.6.3 Nutritional consequences of ascites
Ascitic volume is normally defined as mild, moderate or severe (see Table 4.6.2) but can exceed 25 litres in some patients. Moderate-to-severe ascites can have a detrimental effect on nutritional status due to multiple factors (Box 4.6.1).
Disease-related anorexia and intake
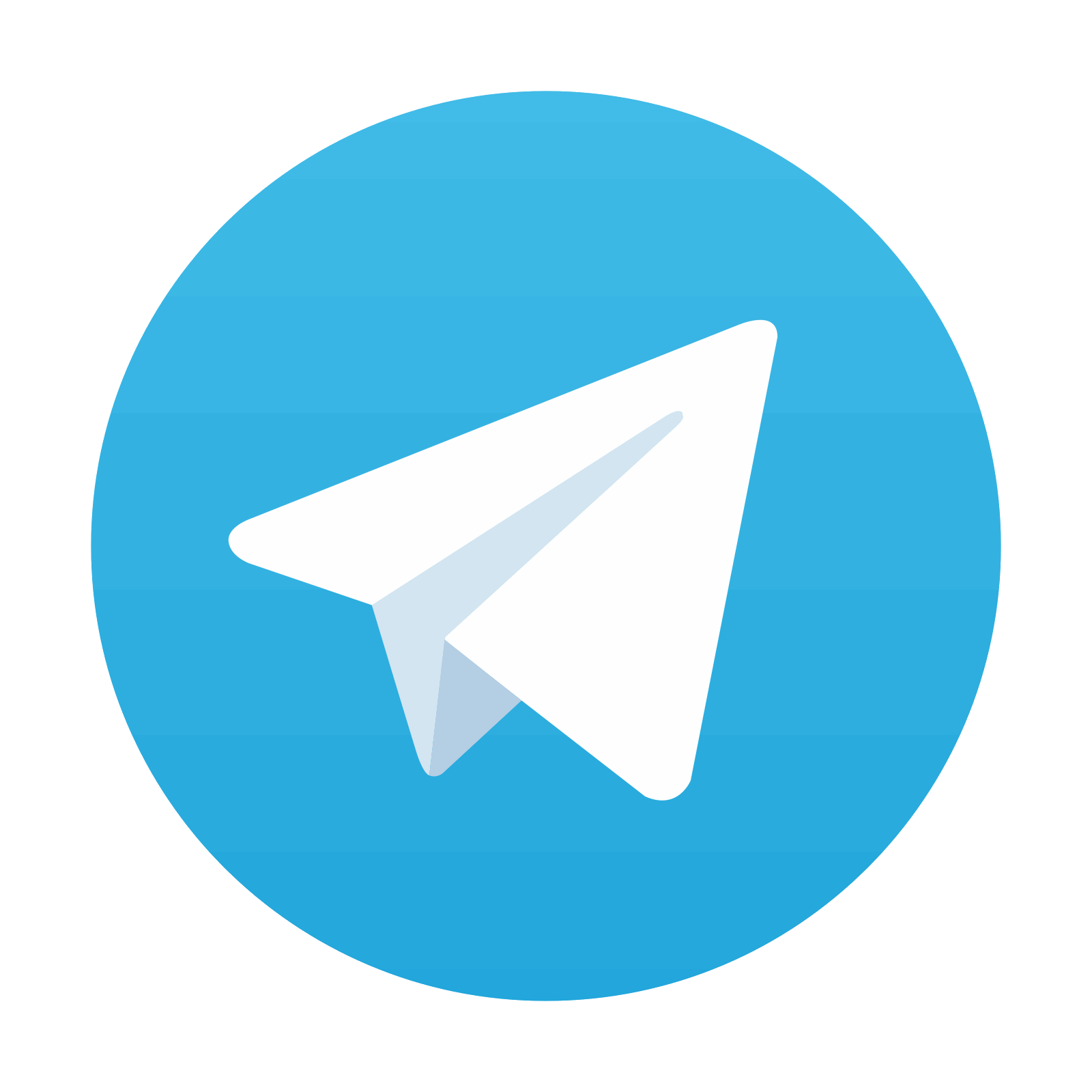
Stay updated, free articles. Join our Telegram channel

Full access? Get Clinical Tree
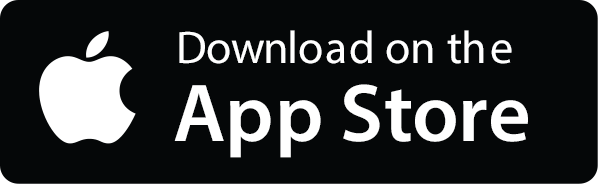
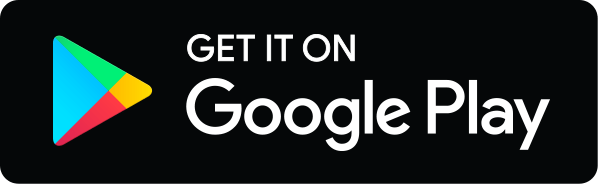