137 Rajan Ramanathan, Yaw A. Nyame, & J. Stephen Jones Department of Urology, Glickman Urological and Kidney Institute, Cleveland Clinic, Cleveland, OH, USA Cryosurgery involves the use of ultra‐low temperatures, below the freezing point of water, which causes thermal tissue damage and cell death. Cryoablation of the prostate is a minimally invasive treatment option for prostate cancer that offers shorter hospital stays, minimal risks of bleeding, and can even be offered to patients who are not good surgical candidates. In 2004 almost 25% of patients underwent minimally invasive primary treatment of prostate cancer and of these 2.8% underwent cryoablation [1]. In 2010 this number had risen to 4% [2], and from the Cryo‐On‐Line Database (COLD) registry data we note that over 50% of patients who underwent cryoablation did so as a primary treatment modality for prostate cancer. Cryoablation actively uses the Joule–Thomson effect. When a compressed gas undergoes sudden expansion it causes a change in temperature. Many gases cause a cooling effect, but helium causes warming (Figure 137.1). This physical effect of cooling during cryoablation was first achieved using nitrogen and then, later, argon. With the demonstration that freeze–thaw cycles cause a more sustained and predictable cell death, the newer generation machines for ablation started using argon–helium combination. From when prostate cryoablation was first described in the 1960s, the technology and technique have undergone a process of steady evolution. Figure 137.1 Ultrathin needle probes: Argon gas expands through the orifice and causes cooling (Joule–Thomson Effect). Source: Endocare Inc., a subsidiary of HealthTronics Inc., Texas, USA. Reproduced with permission of HealthTronics, Inc. One of the earliest descriptions of cryosurgery involved the use of salt solutions to achieve subzero temperatures in order to induce tissue destruction in easily accessible cancers like those of the breast and uterine cervix. Subsequently cotton swabs soaked in liquid nitrogen were described for the treatment of skin lesions [3]. In 1961, Cooper and Lee first described the use of a hollow cannula constructed of stainless steel with an outer and inner tube. The length of the cannula was electrically insulated using copper wire and this was kept at body temperature, but the tip itself was noninsulated and was used for delivery of iceball measuring 8 mm in size in their experiments [4]. Prostate cryosurgery was first described by Gonder et al. in the 1960s [5, 6], and since then many technical refinements have continued to occur. The third‐generation devices now use the argon–helium gas combination to produce the freeze–thaw cycles. Ablation probes have also decreased in size, and fine 17 gauge cryoprobes can now deliver precise iceballs measuring 8–10 mm in diameter. The use of transrectal ultrasound (TRUS) to monitor the “cryolesion” or iceball was developed and popularized by Onik et al. in 1988 [7], and subsequently the same group described the use of a urethral warming device in 1993 to protect the urethra against injury [8]. Techniques aimed at improving the efficiency of cryoablation while minimizing complication rates followed. Onik et al. reported that raising the TRUS probe to push on the posterior wall of the rectum caused the anterior rectal wall to be pulled away from the prostate, creating a spatial separation between the prostate and the rectum [8]. Onik later described another technique to further accentuate this separation by injecting saline into the layers of the Denonvilliers’ fascia [9] to minimize the chances of a rectal injury and rectourethral fistula. They also showed that the use of 6–8 cryoprobes results in better prostate tissue destruction than the original five‐probe technique [9]. Prostate cryoablation devices are now into the third generation. The first‐generation devices used liquid nitrogen and the probes were larger. The total time required for the procedure was also longer and temperature monitoring was not possible. The machines were also bigger and lacked mobility (Figure 137.2). The second‐generation machines were more compact, and used freeze–thaw cycles with helium gas for the thaw cycles. The third‐generation machines incorporated the technology into ultrathin 17G probes, along with temperature monitoring (Figure 137.2). Figure 137.2 First‐ (left), second‐ (center), and third‐generation (right) cryoablation machines. Source: Endocare Inc., a subsidiary of HealthTronics Inc., Texas, USA. Reproduced with permission of HealthTronics, Inc. Cryoablation causes cell damage through a drop in temperature that causes freezing. The aim of cryoablation is to cause destruction of the tumor by creating overlapping cryoablation zones to encompass the entire volume of tumor, while minimizing collateral damage to surrounding normal tissues. Various studies have looked at the minimum temperature required to achieve destruction of tumors, and the mechanisms of injury, importance of cooling rates, and time of contact, as well as the effect of freeze–thaw cycles. In their review, Cooper summarized five mechanisms that are responsible for tissue destruction: dehydration and change in electrolyte concentration due to removal of water from solution, crystallization with rupture of cellular membranes, permanent change in the protein molecules of the cell membrane causing irreversible loss of semi‐permeability, thermal shock, and vascular stasis and coagulation [10]. Today we understand the importance of intracellular ice formation which causes the secondary osmotic shifts from structural damage to the semipermeable membrane. This has been postulated to be an important mechanism of cellular rupture and death in cells in contact with, or very near to the cryoprobe and its iceball. However, there is a rise in temperature more peripherally and in this zone other mitochondrial and cytokine‐mediated cellular injury occurs [11]. Studies have shown that Bax, a cytoplasmic protein that promotes apoptosis, is increased in the cytoplasm on thawing, [11], but it is the ratio of the Bax to the Bcl‐2 (a mitochondrial membrane antiapoptotic protein) that determines whether a cell can survive the effect of marginal freezing that does not cause direct cell rupture [12]. Robilotto and colleagues have performed in vitro studies describing possible apoptotic pathways responsible for cell death using PC‐3 prostate cancer cell lines and by analyzing caspase activity. Caspase‐9 is considered a key component of the intrinsic pathway that follows release of mitochondrial proteins and cytochrome c, whereas caspase‐8 is a marker of the Fas pathway death‐induced signaling complex (DISC) [13]. Robilotto et al. showed that cell death started within 30 minutes of freezing to temperatures <−30°C, peaked at 90 minutes and was complete within 6 hours. Cell death in this model occurred predominantly through membrane‐mediated pathways [13]. Apoptosis was more delayed in cells that were exposed to temperatures over −30°C occurring between 6 and 24 hours, and the mechanism was believed to be through an intrinsic or mitochondrial pathway [13]. Other in vitro cryoablation models have been conducted in the laboratory for the purpose of determining the causes of cell death and also to refine ablation techniques (i.e. to optimize the number of freeze–thaw cycles). Temperatures of −15°C to −20°C are achieved before ice crystals start to form [10]. However, Neel et al. showed that even −28°C is not uniformly lethal to tumor cells [14], and recommended repetitive freezing to temperatures below −60°C for effective tumor ablation [15]. Staren et al. studied the effect of cryoablation on breast tumors, and found that when the temperature at the periphery of tumors was maintained at −40°C for <7 minutes, there was good response in tumors <1.5 cm, but there was viable tumor cells at the periphery with larger tumors. When the temperature was further dropped to −70°C, there was still no significant difference in the outcomes even with the addition of a two‐freeze, passive thaw cycle, until the freeze time was increased to 15 minutes [16]. A general consensus exists that temperatures of −40°C, have been shown to cause adequate cell destruction. The mechanism of cell death is different at different freezing rates: at rapid freeze rates of over 25°C per minute it is due to rapid intracellular ice formation, but at slower freeze rates of less than 5°C per minute, changes in the chemical composition of the cells were the main mechanism of cell death [17]. Since intracellular ice formation is an important component of cryoablation, it is essential to understand the factors that affect it: rate of cooling and the endpoint temperature can be controlled extrinsically, but the water content of tissues and the presence or absence of nearby heat sinks (such as major blood vessels that can transfer energy rapidly and prevent achievement of target temperatures) are intrinsic to the tissues being ablated. The cooling rate and endpoint temperature achieved are also interrelated. A more rapid cool rate of 25°C/minute can achieve cell death at higher temperatures of −19°C and the endpoint temperature does not need to go all the way down to −40°C [17]. Ice is a bad conductor of heat and, as more ice forms, it starts to prevent further propagation of the iceball. There are, therefore, limits to the size of the iceball that can be achieved for a given rate of cooling, and for cooling rates more than 25°C/minute, an iceball equilibrium is achieved at 8–10 mm in size [17]. Today’s latest generation machine can achieve adequate intracellular ice formation through double or even triple freeze–thaw cycles [17, 18], and by ensuring the correct cooling rate for a given endpoint temperature. The commonly used cryoblation techniques are shown in Figure 137.3. Targeted ablation is not often used since a conventional biopsy cannot detect all tumors. Targeted ablation is ablation of a well‐defined lesion, proven on biopsy. The ablation aims to destroy the tumor and a part of surrounding normal prostate similar to the partial nephrectomy model. (Figure 137.3 left side of prostate) This form of ablation can have a good nerve‐sparing effect, but the risk of recurrent or residual cancer is higher. Targeted ablation would be optimal for repeat or second ablations in patients who have had a primary ablation. Zonal ablation is a slightly more extended form of ablation that involves more prostate tissue and aims to encompass all areas with biopsy‐proven cancer. In general, zonal ablation involves contiguous areas, but can be combined with targeted ablation of a single focal lesion too. Hemigland ablation (Figure 137.3 right side of the prostate) involves ablation of one lobe of the prostate and whole‐gland ablation involves ablation of the whole prostate. Figure 137.3 Types of cryoablation: Targeted ablation on the left side of the prostate and hemiablation on the right. Prostate cryoablation is now being offered in the primary and salvage setting. Appropriate case selection is essential for ensuring optimal results, better biochemical relapse‐free survival, and lower complication rates. An ideal treatment model relies on the accurate and consistent identification of tumors in their entirety, and treatment delivery that consistently and predictably eliminates all of the cancer without causing any collateral damage to normal tissues. Cryoablation for prostate cancer does not consistently conform to the ideal treatment model. First, assessment of the anatomical extent of disease in terms of tumor location and volume cannot be accurately or consistently performed in all cases. Second, cryoablation, as a technology, has some limitations in how consistently and predictably it can eliminate prostate cancer without affecting the urethra, external sphincter, and neurovascular bundles. Lastly, there are challenges with following patients after nonexcisional treatment such as cryoablation, since we continue to rely on surrogate markers of recurrence used after radiation therapy. Since the aim of treatment is to provide oncologic cure, while minimizing complications, it is extremely important to identify any parameters that are statistically associated with higher failure rates or with poorer clinical outcomes. In general, patients with high‐volume or aggressive disease, such as extraprostatic extension or seminal vesicle involvement, will demonstrate poor clinical outcomes as a result of higher rates of residual disease and/or higher rates of complications. On the other hand, patients without high‐volume or high‐risk disease are potential candidates for focal treatment, which has lower complication rates compared to whole‐gland cryoablation. A study on salvage cryoablation showed higher rates of urinary incontinence (13% vs. 0%), erectile dysfunction (100% vs. 71%), and fistulas (4% vs. 0%) in patients undergoing total cryoablation as compared to focal ablation [19]. Prostate cancer is often multifocal, as found in 79.5% of whole‐mount prostate specimens after radical prostatectomy [20]. Cancer proximity to the urethra is also another cause for concern since urethral warming devices, designed to prevent cryo‐injury to the urethra can potentially result in undertreatment in select patients. Studies have shown that in up to 66% of cases, foci of cancer were found within 5 mm of the urethra [20] with a mean distance of 3 mm from the urethra (17% of them in contact with the urethra) [21]. The prostate apex is another area that is at risk for residual cancer after cryoablation, with some studies suggesting that up to 74% of patients have apical cancers [21]. Results from salvage prostatectomy series also reflect similar patterns, with multifocal cancers seen in 28% of men, apical recurrences in 95% of men, and 65% of cancers found to be within 5 mm from the urethra (7% involving the urethra) [22]. In fact, radiation‐resistant cancers show high incidence of extraprostatic extension (43%) and seminal vesicle involvement (28–62.5%) [22, 23], and such patients are not suitable for cryoablation with curative intent. Routine prostate biopsies often do not detect the extent or volume of prostate cancer, and studies have demonstrated multifocal bilateral disease in 70.7–72.0% of men diagnosed with unilateral cancer on biopsy after review of the final radical prostatectomy specimens [24, 25]. Moreover, extracapsular disease was seen in 5% of men who underwent prostatectomy, on the side deemed to have completely benign pathology at biopsy [24]. More extensive saturation biopsies, in theory, may appear to be able to improve detection rates. This may then improve concordance between biopsy and radical prostatectomy [26], but other studies have not confirmed this. True unilaterality was inaccurately noted in 90% of patients (35 of 39) and 1–3 prostate cancer foci were missed on the saturation biopsy, of which 76% were determined to be clinically significant [27]. Multiparametric magnetic resonance imaging (mpMRI) fusion biopsy is increasingly being used and is now a recommendation for the workup of patients undergoing focal therapy as part of clinical trials [28]. Siddiqui et al. showed that there is good concordance between mpMR fusion biopsy and standard biopsy (69%). More specifically, mpMRI‐targeted fusion biopsy demonstrate a 30% improvement in the detection of significant cancer over standard 12‐core biopsy, and a combination of mpMRI‐targeted and standard template biopsy had an overall sensitivity of 85% for detecting prostate cancer [29]. However, mpMRI technology is relatively new, with a significant economic cost of adoption, and results will vary based on physician experience. In addition, some tumors are not visible on mpMRI, and technical errors such as patient movement or mismatches in registration and/or fusion can cause inadequate sampling of the target lesion [30]. Conventional prostate cancer staging such as computed tomography (CT) abdominal sectional imaging and bone scans are inaccurate at the lower PSA levels commonly seen in the group of patients undergoing focal therapy. Overall, MRI staging accuracies of 71–72% have been reported [31]. However, there are some useful findings that MRI can provide with regard to disease staging. For example, the presence of suspected T3 disease on MRI is highly correlative, with metastatic disease in nodes as small as 0.8–1 cm, the accuracy of which improves when combined with staging nomograms [32]. The rates of micrometastatic nodal disease can be predicted with high sensitivity (88.9–100%) and specificity (65–94.6%) in the presence of clinical T3 (a and b) disease on MRI [31]. The role of a routine pelvic lymph node dissection (PLND) for staging prior to cryoablation has not been established. PLND can be done either by the conventional open technique or by laparoscopic or robot‐assisted laparoscopic techniques. For PLND to be useful, enough lymph nodes, have to be sampled. The rate of residual cancer is about 13% in patients who undergo standard PLND when compared to extended templates which includes the presacral nodes [33]. An open extended PLND does blunt the advantage that a minimally invasive procedure such as cryoablation has to offer in the first place. Advances in minimally invasive surgical techniques provide additional options to open PLND that may confer the added benefit of less pain, improved cosmesis, and less hospital days with acceptable oncologic outcomes, but many of these studies demonstrated the efficacy of the minimally invasive technique when offered concurrently with the prostatectomy [34]. At this time standard open PLND is associated with a yield of 21–23 nodes [35], and minimally invasive approaches to PLND may potentially understage patients as a result of lower node counts on resection [36]. Assessing occult bone metastases can also be challenging. When the PSA is <10 ng/ml, bone scans have not been shown to be useful. Positive bone scans are seen with increasing PSA levels, rising from 8% for PSA levels between 20 and 50 ng/ml to 40% for PSA >50 ng/ml [37]. Other modalities, including NaF positron emission tomography (PET)/CT using 18F‐sodium fluoride [38], MRI–diffusion‐weighted imaging (DWI) and 11C‐choline PET/CT [39], and fluciclovine (18F) PET scans [40], are demonstrating promise in improving the detection of bony and other visceral distant metastases. Prostate cryoablation is now being offered to more patients in the primary setting. When the COLD registry was reviewed as of December 2015, over 5000 patients were noted to have undergone primary cryoablation (Figure 137.4). A consensus study involving experts in the field of focal therapy looked at selection criteria for patients. There was a high level of agreement that focal therapy should now be used more in National Comprehensive Cancer Network intermediate‐risk patients, and this is a shift over time, from previous policies restricting treatment to the low‐risk group, due to concerns about “overtreatment” in the low‐risk group [41]. There are other studies showing the effectiveness of focal therapy in the intermediate‐risk group. Bahn et al. studied focal ablation in a selected group of 73 patients: 48 underwent biopsies after hemigland ablation for low and intermediate disease. Although 12 biopsies were positive, only 1 was positive on the treated side [42]. In their matched‐pair analysis with radical prostatectomy, patients fared comparably to surgery, and there was no statistical difference in the need for salvage treatment [42]. However, when there is evidence of locally advanced or clinical T3 cancer, a much higher rate of biochemical failure (48–52%) is noted compared to radical prostatectomy or radiation therapy [43, 44], with higher rates of rectourethral fistula [43]. Figure 137.4 Cryo‐On‐Line Database (COLD) data: Number of patients undergoing cryoablation as of December 2015. (data provided by Ahmed Elshafei) Undiagnosed lymph node involvement (LNI) can occur and could be a cause of treatment failure. Accurately discerning the presence of LNI presents challenges to practitioners performing cryoablation, as positive nodes cannot be reliably identified by standard imaging modalities. For this reason, PLND is recommended for high‐risk patients (i.e. those with higher PSA, higher grade disease, and shorter PSA doubling times). Primary cryoablation should be offered to patients with acceptable risk criteria. Low‐risk patients may be better followed on active surveillance and a recent study showed an actuarial cause‐specific survival rate of 98.1% and 94.3% at the 10‐ and 15‐year period, with 2.8% of patients developing metastatic disease [45]. Intermediate‐risk patients with preexisting erectile dysfunction may be excellent candidates for cryoablation. Intermediate‐risk patients with good preexisting sexual function should be aware that postprocedure sexual dysfunction is a possibility before proceeding with cryoablation. Although there are studies to demonstrate effectiveness of cryoablation for high‐grade disease [43] routine use of cryoablation for patients with Gleason scores 8 or greater, tumor stage >T3, or those suspected of having LNI is not recommended. Similar criteria for patient selection applies to men undergoing salvage cryoablation after radiotherapy. The diagnosis of prostate cancer recurrence after radiotherapy needs to be confirmed and is aided by numerous imaging modalities, including MRI and confirmed by a biopsy. Pelvic MRI can utilize diffusion weighting and dynamic contrast enhancement to differentiate benign tissue from malignant disease. Magnetic resonance spectroscopy can be used to detect local prostate cancer recurrence [46]. In addition, mpMR has demonstrated increased accuracy in detecting prostate cancer in patients when compared to standard T2‐weighted images, and consequently has significant utility in the detection of disease recurrence following radiotherapy [47]. These adjunctive imaging modalities can facilitate the selection of men for prostate biopsy who are at risk for local recurrence following radiotherapy. Guidelines on the use of salvage radiation are often used for selecting men for cryoablation [48]. Specifically, these guidelines suggest that patients should demonstrate only localized recurrence without distant metastases, longer time interval (>2 years) between primary treatment and recurrence, PSA levels <10 ng/ml, PSA doubling time of >6–9 months, and a life expectancy greater than 5–10 years. These guidelines have been standardized for salvage cryoablation, and a study panel recommended men should typically have a PSA <15 ng/ml, clinical tumor stage T1c/T2a, Gleason score 3 + 3 or 3 + 4, a life expectancy of more than 10 years, and any prostate volume [28]. A complete preoperative assessment is recommended for patients undergoing cryoablation and this includes anesthesia clearance, and appropriate cardiac and respiratory testing. Strict exclusion criteria for treatment, based on our experience, include uncontrolled bleeding diathesis or anticoagulant therapy that cannot be discontinued perioperatively. For those who can hold anticoagulants, these medications should be resumed within 24–48 hours following the procedure or after the urine clears, whichever is earlier. A limited bowel preparation may be used, at the discretion of the practitioner, to free the rectum, however, there is no evidence that a bowel prep decreases treatment efficacy or decreases the rates of postoperative complications, such as infection. Standard antibiotic prophylaxis should be provided for no more than 24 hours in accordance with guideline recommendations and started within 1 hour of starting the procedure. Urethral strictures may need an internal urethrotomy or dilation and anal stenosis may require dilation in order to facilitate the insertion of a Foley catheter and the rectal probe, respectively, at the time of the procedure. Patients without a rectum or anal opening, such as patients who have undergone abdominal–perineal resection, are not suitable for cryoablation and other treatment modalities should be considered. The last consideration prior to cryoablative therapy is the prostate gland volume. Men with very large glands are at higher risk of requiring multiple probes and are at increased risk of undertreatment. In this setting, neoadjuvant androgen‐deprivation therapy (ADT) can be used to reduce gland volume prior to treatment. Specifically, neoadjuvant ADT has been associated with a mean reduction in prostate volume of 35% and tumor volume reduction of 50% within two months of treatment, although this rarely downstages the existing disease [49]. In one retrospective study, neoadjuvant ADT in men undergoing primary cryoablation (compared with those who did not get neoadjuvant ADT) demonstrated no significant differences in treatment outcomes or complications, with the exception of intermediate‐risk men who had improved 5‐year disease‐free survival [50]. For these reasons, in our experience, we limit whole‐gland cryoablation to men with prostate volumes greater than 80 g. For larger glands, we have used neoadjuvant ADT for size reduction for six months in men who could wait without high risk of disease progression. The patient is brought to the operative room and anesthesia is induced at the discretion of the anesthesia team. The patient is then positioned in the dorsal lithotomy position with appropriate padding, avoiding hyperflexion to minimize neuropraxia or injury. The lower abdomen, genitalia, and perineum are prepped using a betadine‐based solution, and draped in the standard fashion. At this time, a transrectal ultrasound probe is introduced into the rectum and the prostate is carefully inspected. Measurements are taken of the prostate to calculate prostate volume. The ablation machine is calibrated for treatment and the precise location of probe insertion is determined. The simulation provides probe targets, and adjustment are made if needed to ensure that the final probe locations are sufficiently far away from the urethra and rectum to minimize injury and fistula.
Cryosurgical Ablation of the Prostate
Introduction
History of cryoablation
Mechanism of tissue injury and cell death in cryoablation
Types of cryoablation
Patient selection
Primary cryoablation
Salvage cryoablation
Procedure, operative considerations, and technique of prostate cryoablation
General considerations and patient preparation
Operating technique
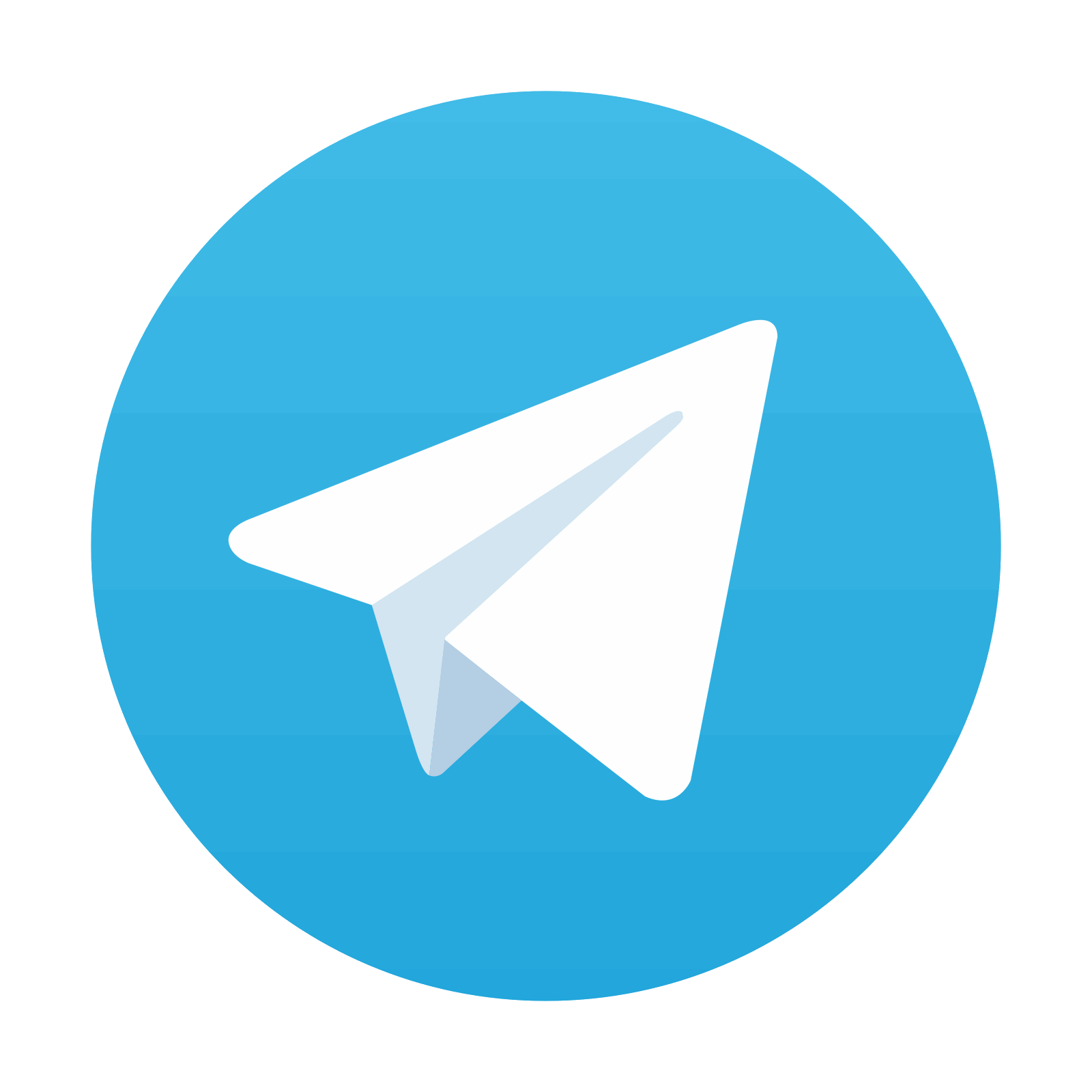
Stay updated, free articles. Join our Telegram channel

Full access? Get Clinical Tree
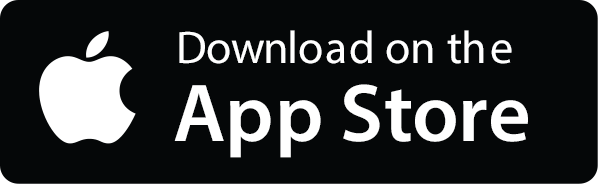
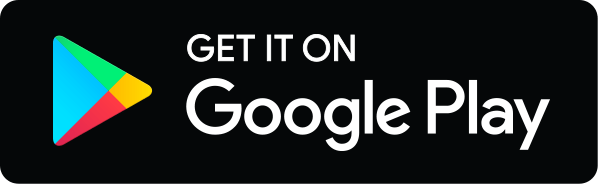