Mike Thomson and Krishnappa Venkatesh Modern endoscopy has recently seen the development of technological advances with the aim of increasing and optimizing diagnostic yield from the procedure. These have included video and magnification endoscopes. Greater surface definition has been achieved with chromoendoscopy and recently, narrow‐band imaging has allowed greater definition of vascular architecture. However, in vivo subsurface pathology remained obscure to the endoscopist until the advent of confocal endomicroscopy which affords magnification up to 1000×, and with sequentially deeper images from the epithelial surface to approximately 250 μm below the surface, producing histological assessment of the in vivo gastrointestinal (GI) mucosal structure at the cellular and subcellular levels. In addition, this technique avoids crush artefact from the grasp biopsy forceps and changes from histopathological processing. The diagnosis of upper GI disorders in children depends, to a great extent, on endoscopy and subsequent histology of biopsy specimens. Pathologies such as gastroesophageal reflux disease (GERD), eosinophilic esophagitis (EE), Helicobacter pylori gastritis, and celiac disease (CD), in conjunction with various other investigative modalities, have histological confirmation as pivotal to their diagnosis. Similarly, pediatric ileocolonic conditions such as inflammatory bowel disease (IBD), familial adenomatous polyposis (FAP), graft‐versus‐host disease (GVHD), and allergic colitis necessitate a tissue diagnosis. Confocal laser endomicroscopy involves the use of a highly miniaturized confocal microscope which has been incorporated into the distal tip of a flexible endoscope to allow in vivo microscopic examination of the gut mucosa. The confocal microscope uses a single optical fiber to deliver 488 nm laser light to the distal tip of the endoscope where it is focused to a single diffraction limited point within the tissue. The laser light excites fluorescent molecules within the tissue. Fluorescent light emanating from the specific point of focus is collected into the same optical fiber of the confocal microscope and delivered to the photodetector. Light emanating from outside the focally illuminated spot is not focused into the optical fiber and is therefore rejected from detection. The focused point of laser light is scanned in a raster pattern across the field of view, and the intensity of the fluorescent signal returning to the detector from successive points is measured (12‐bit digitization) to produce two‐dimensional images that are en face to the tissue surface. By moving the microscope optics within the confocal microscope, the operator can dynamically adjust the imaging depth to allow microscopic imaging at and below the surface of the mucosa; hence, each image is an optical section representing one focal plane within the specimen, and collection of multiple optical sections at successive depths results in true volumetric sampling of the tissue. As a three‐dimensional volume is thus sampled, this can be thought of as a “virtual biopsy.” The Pentax EC3870CILK endoscope has a 5 mm diameter miniaturized confocal microscope integrated into the distal tip of the endoscope (Figure 18.1). The diameter of the distal tip and insertion tube of the endoscope is 12.8 mm. In addition to the integrated confocal microscope, the distal tip also contains a colour CCD camera which enables simultaneous confocal microscopy with standard video‐endoscopy, air and water jet nozzles, two light guides, a 2.8 mm working channel and an auxiliary water jet channel. During CLE, the laser delivers an excitation wavelength of 488 nm at a maximum laser output of 1 mW to the tissue (typically 300–700 W). Confocal images can then be collected at either 1024 × 1024 pixels (0.8 frames/second) or 1024 × 512 pixels (1.6 frames second). The optical sections have a 475 × 475 μm field of view, with a lateral resolution of 0.7 μm, axial resolution of 7.0 μm, and the imaging depth (z‐axis) range of 0–250 μmbelow the tissue surface in 4 μm steps. The imaging depth below the tissue surface can be dynamically controlled by the operator. CLE magnifies images 1000‐fold and they are visualized in real time on a monitor. A library of images is then collected and analyzed subsequently or contemporaneously. Figure 18.1 Confocal laser endomicroscope (Pentax). Fluorescein sodium (FS) 10% and acriflavine hydrochloride (AH) 0.05% are used as contrast agents. FS is highly water soluble and, on intravenous administration, rapidly diffuses in seconds from the capillaries into the extravascular tissue. FS, when exposed to light of wavelength 465– 490 nm (blue), emits light at longer wavelengths (520–65 nm, with peak emission in the 520–530 nm green‐yellow region). This enables visualization of microvessels, cells, and connective tissue. However, FS is not enriched in the nuclei of intestinal epithelial cells and hence the nuclei are not readily visible in the confocal images. To circumvent this limitation, AH (0.05%) is used topically to enrich the superficial nuclei and to a lesser extent the cytoplasm. To limit peristaltic artefacts, 10–20 mg Buscopan (hyoscine‐N‐butyl‐bromide, Boehringer, Ingelheim, Germany) can be given intravenously. Following duodenal or ileal intubation, 0.05– 0.1 mL/kg of 10% fluorescein sodium is administered intravenously and flushed adequately with normal saline. AH (0.05%) is applied to the mucosa using a spray catheter at all sites undergoing confocal imaging. Confocal laser endomicroscopy image acquisition is performed by placing the tip of the colonoscope in direct contact with the target tissue site. Using gentle suction to stabilize the mucosa, image acquisition and focal plane z‐axis scanning depth is actuated using two discrete handpiece control buttons. Confocal images are sequentially obtained from third‐part duodenum, gastric antrum and body, distal and proximal esophagus in the upper GI tract, and ileum, cecum, ascending, transverse, descending, and sigmoid colon, and rectum in the ileocolon. Confocal images are acquired simultaneously with ongoing video‐endoscopic imaging. Same‐site mucosal specimens are obtained using standard biopsy forceps. The biopsy specimens are fixed in buffered formalin solution, embedded in paraffin wax, serial section obtained and stained with hematoxylin and eosin (H&E). The histological specimens from each site are compared with same‐site confocal images jointly by the endoscopists and experienced pediatric and GI histopathologists, who are often in the endoscopy room. It is notable that the CLE images are cross‐sectional in parallel with the mucosal surface, which is in contrast to standard histology in which a vertical section is made. Once this adjustment in perception is made by the observer, it is relatively easy to compare CLE images in vivo and postfixed tissue sections by histology. Of course, no artefact due to fixing and staining occurs in the CLE images. Recently in our center, CLE occurred in 57 children intubating the duodenum at upper GI endoscopy, and the terminal ileum at ileocolonoscopy. In all those in whom this occurred, the pylorus at upper GI CLE and the terminal ileum at lower GI CLE were intubated in all patients except one – the exception being a patient who weighed 10 kg and who was 8 months old in whom the pylorus and ileocecal valve were both too narrow to accept the confocal endomicroscope. The youngest and smallest patient to have full successful examinations up to third‐part duodenum and terminal ileum was 18 months old and 11 kg. The procedure time for upper GI endoscopy ranged from 7 to 25 minutes (median 16.4 minutes), and for ileocolonoscopy 15–45 minutes (median 27.9 minutes). A total of 132 pinch biopsies were taken from the upper GI tract from 33 procedures and 184 from the ileocolon from 30 procedures, i.e., not all patients had both upper and lower GI CLE. No complications or adverse effects occurred except on one occasion when precipitation was observed in the peripheral venous line when fluorescein was injected immediately after neostigmine, but no patient morbidity occurred as the precipitant did not enter the patient. In our unit, 33 patients underwent upper GI CLE, and the duodenum was intubated in all but one. A total of 4368 confocal images was obtained which included 1835 from the duodenum, 1451 from the stomach and 1082 from the esophagus, which were compared with 44 biopsies from each site. The esophagus is lined by nonkeratinized squamous epithelium with polygonal epithelial cells. The nuclei of the epithelial cells are highlighted clearly following topical administration of acriflavine. Further, the capillary loops in the papillae are visible in deeper planes following subsurface optical sectioning, and surface to capillary distance can be measured as each level is deeper by 4 μm. This allows assessment of GERD‐like histopathology given that papillary height is increased and epithelial surface to papillary tip (i.e., where capillary loops appear on confocal endomicroscopy) distance is thereby shorter. Recent in vivo data indicate that the epithelium is thinner in GERD versus normal versus EE and this is a very interesting phenomenon as it may allow real‐time differentiation of esophageal pathologies. The gastric pits or foveolae appear as invaginations on the surface epithelium. Each confocal image shows several evenly spaced such pits lined by columnar epithelium. The centers of the pits appear dark. On confocal imaging, the duodenal villi have a long, slender and finger‐like appearance similar to histological specimens. The single layer of brush border columnar epithelial cells interspersed with intraepithelial lymphocytes and goblet cells is well visualized. Crypts are not usually visible except in the presence of villous atrophy. Celiac disease is particularly amenable to diagnosis by CLE. Comparative histological and CLE images are shown in Figure 18.2. On confocal imaging, in CD with subtotal villous atrophy (Marsh type 3a/b) (Figure 18.2a), the duodenal villi are broad with loss of the hexagonal pattern of the surface epithelium and a decrease in goblet cells. A characteristic feature observed was linking between adjacent villi giving an appearance of the villi being “sticky” (Figure 18.2b). Furthermore, the villi appear to be folded onto themselves. In contrast, in CD with total villous atrophy (Marsh type 3c on histology) (Figure 18.2c), on confocal imaging, villi are absent and crypts are visible with dense cellular infiltration in the surrounding stroma (Figure 18.2d), similar to histology. Figure 18.2 Comparison of confocal images with conventional histological images in celiac disease. (a) Histological image showing marked villous atrophy with increased intraepithelial lymphocytes and crypt hyperplasia. (b) Comparative confocal image. (c) Histological image showing total villous atrophy of the duodenum. (d) Comparative confocal image. The typical features of the colonic crypt pattern can be seen in Figure 18.3 with a polygonal appearance. Goblet cells show up as dark cells, whilst enterocytes are light gray. Technological innovations have led to the development of chromoendoscopy in which dyes such as methylene blue and indigo carmine have been used to aid localization of lesions, and magnifying endoscopy has enabled visualization of surface structures at approximately 100‐fold magnification. In adults, several studies have validated these techniques in differentiating neoplastic from nonneoplastic lesions, diagnosis of neoplastic lesions in flat and depressed lesions in the colorectum, and in cancer surveillance in patients with long‐standing ulcerative colitis. Differentiation of types of colitis is feasible although specificities such as presence of eosinophils are not observable. Typical features of ulcerative colitis are demonstrable, and CLE allows targeting of biopsy taking in order to allow such issues as dysplasia to be identified. The following features are seen in inflammatory bowel disease on histology and on CLE (Figure 18.4). Figure 18.3 Comparison of confocal images with conventional histological images of the lower GI tract. (a) Confocal image of normal colonic mucosa showing regularly spaced crypts with numerous goblet cells. (b) Comparative histological image – note different plane. Figure 18.4 (a) Inflammatory bowel disease showing bifid crypt pattern, crypt distortion/destruction/abscess and cryptitis, goblet cell depletion, inflammatory cell infiltration prominence. (b) Comparative confocal image showing similar features plus tortuous vessel architecture suggesting inflammatory activity. Figure 18.5 CLE of GVHD showing nuclear debris representing apoptotic bodies and obviating the need for taking of actual biopsies for histological confirmation. Granulomata cannot reliably be distinguished as yet, in our experience, but ileal inflammatory features can be, and therefore distinction between ulcerative colitis and Crohn’s disease is further aided. Other pathologies such as collagenous colitis are noted easily with the typical increase in the thickness of the basement membrane. Graft‐versus‐host disease (GVHD) may also be identified with nuclear debris representing apoptotic bodies in the rectal mucosa (Figure 18.5), and this would potentially negate the need for biopsy which, in immunocom‐promised children who may be at increased risk of mucosal infection and hemorrhage associated with biopsies being obtained, offers a cogent clinical benefit over standard endoscopy and biopsy. The technology of CLE offers an insight into in vivo histology and allows targeting of biopsies which not only allow more accurate diagnostics but may help in diminishing the number of biopsies and concurrent costs to an endoscopy service. Smaller endoscopes may be useful but we have shown this technology to be applicable practically in children as young as 18 months of age. The tantalizing prospect of targeted biopsies or even a biopsy‐free endoscopic procedure in the diagnosis of childhood GI disorders arises with obvious potential benefits in terms of avoidance of biopsy‐associated complications and diminution of the considerable histological burden that this patient cohort places on already overstretched histopathological services with the prospect of considerable associated cost savings.
18
Confocal laser endomicroscopy in the diagnosis of pediatric gastrointestinal disorders
Contrast agents
Upper GI tract
Lower GI tract
Conclusion
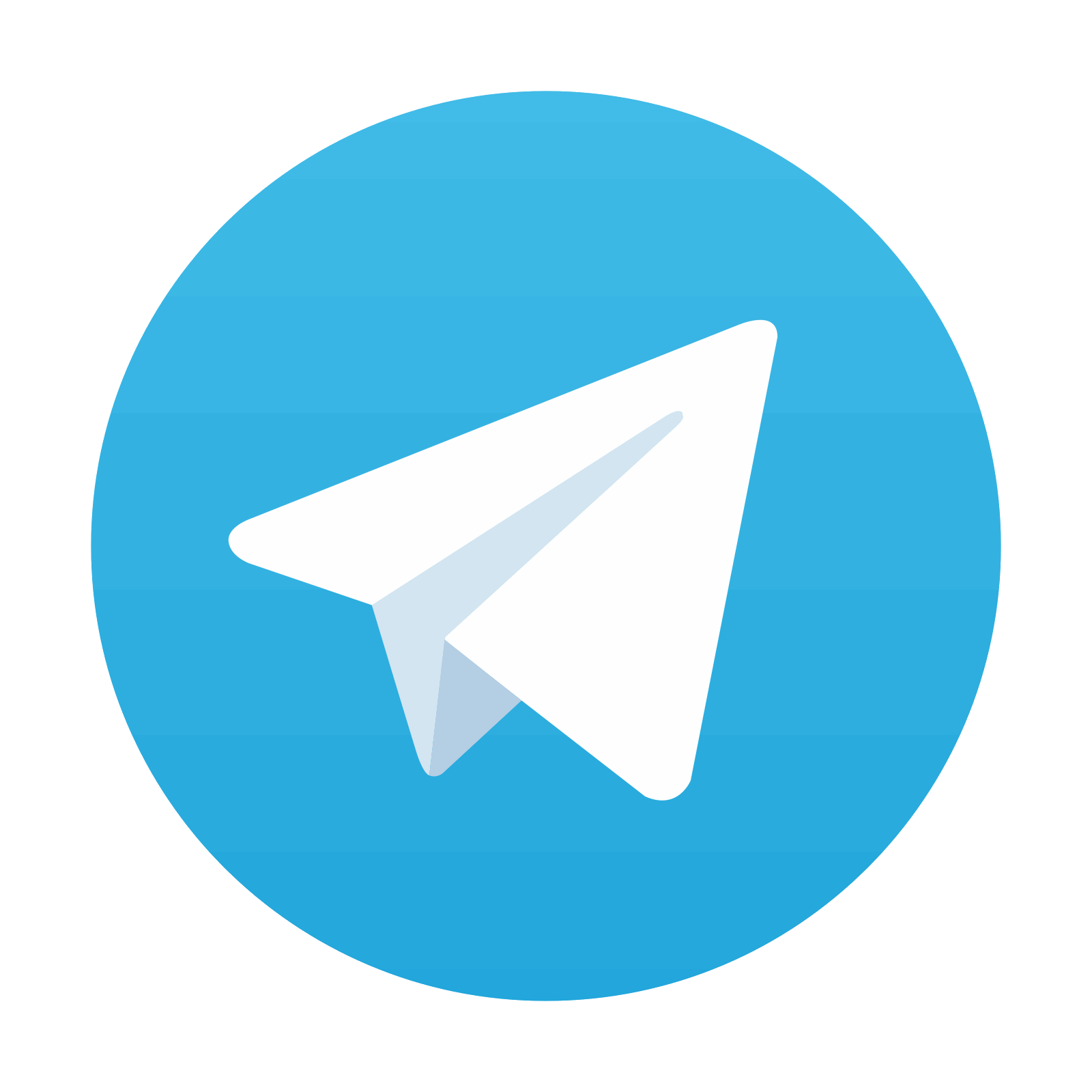
Stay updated, free articles. Join our Telegram channel

Full access? Get Clinical Tree
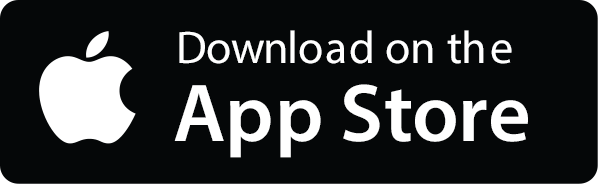
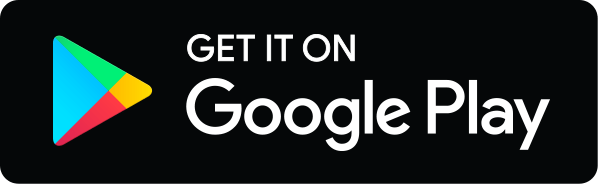