Fig. 1
(a) Liver transplants done as part of combined organ transplants (Data from http://srtr.transplant.hrsa.gov/annual_reports/2012/. slide 34 SRTR report liver slides). (b) Total number and percentage of simultaneous liver–kidney transplantation (SLK) of all deceased donor, adult liver transplantation. Model of the end-stage liver disease (MELD) score was implemented in February 2002. Original reference is Data from Organ Procurement and Transplantation Network (OPTN) as of June 2011 (http://optn.transplant.hrsa.gov) (This figure is adapted from Nadim et al. 2012)

Fig. 2
Kidney listings after Liver Transplants (Data from United network for organ sharing)

Fig. 3
Kidney transplants after liver transplants (Data from United network for organ sharing)
Estimating Renal Function
The preoperative evaluation of renal function in candidates being considered for simultaneous renal and nonrenal solid organ transplantation should emphasize assessment for a past history of AKI including duration and prior reversibility, risk factors and stage of CKD, and best estimates about anticipated rate of progression of CKD to end-stage renal disease after nonrenal solid organ transplantation. This starts with a detailed history and physical examination, an accurate measure of kidney function, urine studies including urinalysis and urine protein/creatinine ratio, and renal ultrasonography. Based on these results, a renal biopsy and other studies may be considered. If dual listing is pursued, waiting time for both organs is determined primarily by liver or heart allocation algorithm. Therefore, the decision to list for a combined nonrenal and renal transplant should be made very carefully given the profound kidney organ shortage coupled with an increased demand with already more than 100,000 patients awaiting a renal transplant alone.
Candidates for liver, heart, or small bowel transplantation typically have reduced muscle mass due to underlying advanced organ failure and consequently, low serum creatinine values. Creatinine is derived from the metabolism of creatine produced by skeletal muscle and from dietary meat intake. It is freely filtered across the glomerulus and is neither reabsorbed nor metabolized by the kidney. However, approximately 10–40 % of urinary creatinine is derived from tubular secretion by the organic cation secretory pathways in the proximal tubule (Shemesh et al. 1985). Creatinine excretion is usually a good clinical marker of renal function since its reabsorption and secretion as well as total production and excretion are equal under steady state. For normal females, creatinine excretion is 15–25 mg/kg of lean body weight/day and for males it is 20–30 mg/kg of lean body weight per day (Rose 1989). These values are helpful to determine if 24 h urine collections are accurate.
Estimation of glomerular filtration rate (GFR) using 24 h urine creatinine clearance is the most commonly available method used clinically with the caveat that certain prerequisite criteria are met to ensure accuracy: (1) Patients have normal muscle mass for their gender. (2) Serum creatinine is stable. (3) Absence of agents which affect creatinine reabsorption or excretion. (4) The 24 h urine collection is complete. (5) Creatinine is equally reabsorbed and secreted. These criteria are frequently violated in patients awaiting a liver, heart, or small bowel transplant since they have underlying sarcopenia. Therefore a near normal serum creatinine value may not necessarily reflect normal kidney function, especially in states of heart or liver failure where affected patients frequently have poor nutritional status, low muscle mass, weight loss, and edema. Values for urine creatinine less than the lower limit for the patient’s gender on a 24 h urine collection usually suggests an undercollection. However, for liver and heart failure patients, it is unusual to find values at or above the lower limit for creatinine excretion due to reduced creatinine generation. To overcome this problem and increase accuracy, repeat 24 h urine collections should be done and total creatinine excretions that are similar on repeated collections suggest that the collections are at least complete (McCauley 1997). Estimation of GFR is most commonly done in clinical practice based on estimation equations utilizing serum creatinine. Some examples of these include Cockcroft-Gault equation, the Modification of Diet in Renal Disease (MDRD) study equations, and the Chronic Kidney Disease Epidemiology Collaboration (CKD-EPI) equation. A recent study using iohexol clearance as the gold-standard measure of GFR in 300 liver transplant candidates confirmed that the six-variable MDRD equation had superior accuracy to the abbreviated MDRD-4 as well as to the CKD-EPI equations in identifying patients with GFR <30 mL/min (Francoz et al. 2014). The OPTN Kidney and Liver Intestinal Organ Transplantation Committees have also recommended using the six-variable MDRD equation for GFR estimation. The six-variable MDRD equation is highlighted below:
(Creatinine and BUN in mg/dl and Albumin in gm/dl)
![$$ \mathrm{eGFR}=170\times \mathrm{Serum}\ {\mathrm{Creatinine}}^{-0.999}\times {\mathrm{Age}}^{-0.176}\times \left[0.762 \mathrm{if}\ \mathrm{Female}\right]\times \left[1.180 \mathrm{if}\ \mathrm{Black}\right]\times {\mathrm{BUN}}^{-0.170}\times {\mathrm{Albumin}}^{+0.318} $$](https://i0.wp.com/abdominalkey.com/wp-content/uploads/2017/08/A321347_1_En_8_Chapter_Equa.gif?w=960)
There are some limitations to using creatinine clearance or serum creatinine-based equations. Overestimation of GFR is commonly encountered in end-stage heart or liver failure since serum creatinine value is falsely low due to underproduction. Overestimation can also happen in chronic kidney disease as the contribution of tubular secretion of creatinine to total creatinine clearance is increased. Some have suggested competitively inhibit creatinine secretion by the administration of cimetidine which blocks the renal tubular secretion, however wide variability in its blocking effect may make interpretation difficult (van Acker et al. 1992). Finally, overestimation is also encountered due to extrarenal creatinine elimination from increased gastrointestinal bacterial overgrowth and increased creatininase activity in advanced kidney failure when estimated GFR falls below ≤15 mL/min/1.73 m2 (Dunn et al. 1997). Therefore, it is reasonable to conclude that creatinine clearance represents an upper limit of what the true GFR may be in these scenarios. In other scenarios, underestimation of GFR is seen due to spuriously elevated serum creatinine levels. Transient increase in serum creatinine acutely as a result of volume depletion or excessive intake of animal protein intake are the most common circumstances when this happens.
More precise methods of estimating GFR rely on the renal clearance of various radionuclide markers, like 99mTc-labeled diethylene triamine penta-acetic acid (DTPA), 51Cr-labeled ethylenediaminetetraacetic acid (EDTA), and 125I-labeled iothalamate (Tanriover et al. 2008) but have restricted application outside of research protocols due to limited availability and cost.
Defining Acute Kidney Injury and Causes of Renal Disease in Liver Failure Patients
Establishing the cause, severity, and chronicity of pretransplant renal dysfunction is important in patients with liver failure. After liver transplantation, analyzing perioperative events and their influence on renal recovery are equally important. These are some of the questions that need to be answered to help develop selection criteria for SLK candidates. Definitive conclusions regarding long-term outcomes in patients undergoing liver transplantation with or without kidney transplantation with pretransplant renal dysfunction are lacking due to lack of standardized definition of AKI. Accordingly, some have adopted the modified RIFLE (Risk, Injury, Failure, Loss, End stage)/AKIN criteria (Acute Kidney Injury network) in cirrhotic patients (Table 1) to complement research initiatives to define patient outcomes (Mehta et al. 2007). The RIFLE criterion has been validated in over half a million critically ill patients including patients with ESLD and has demonstrated strong concordance for mortality predictions with worsening RIFLE class (Jenq et al. 2007; O’Riordan et al. 2007; Ferreira et al. 2010).
Table 1
Modified RIFLE/AKIN criteria for definition and classification of acute kidney injury
AKI stage | Serum creatinine criteria | Urine output criteria |
---|---|---|
1 (Risk) | Increase Scr of ≥0.3 mg/dL within 48 h or a 1.5- to 2-fold increase from baseline | >0.5 mL/kg/h for >6 h |
2 (Injury) | Increase Scr >2- to 3-fold from baseline | >0.5 mL/kg/h for >12 h |
3 (Failure) | Increase Scr >3-fold from baseline or Scr ≥4.0 mg/dL with an acute increase of ≥0.5 mg/dL or initiation of renal replacement therapy | >0.3 mL/kg/h for 24 h or anuria for 12 h |
The etiology for AKI is variable in patients with liver disease ranging from prerenal etiology, hepatorenal syndrome, and acute tubular injury before liver transplantation to post-transplant events like CNI-induced nephrotoxicity and tubular injury related to the procedure itself (Table 2). Increased severity of liver failure is marked by increased renal vasoconstriction. Many potential mechanisms are implicated in causing this predisposition such as activation of renal angiotensin system, sympathetic overactivity, and a decrease in vasodilators like prostaglandins and kinins. Hepatorenal syndrome is usually difficult to differentiate from prerenal azotemia but lack of improvement after fluid challenge in hepatorenal syndrome is a differentiating factor. Finally, other postrenal causes such as obstruction can be easily ruled out by obtaining a kidney ultrasound to rule out hydronephrosis. This may also provide information about the size, echogenicity of the kidneys to assess if CKD is present and a Doppler study will rule out renal artery stenosis (McCauley 1997).
Table 2
Differentiating factors for most common causes of AKI in patients with liver disease
Laboratory parameter | Prerenal azotemia | Hepatorenal syndrome | Acute tubular necrosis |
---|---|---|---|
Urinary sodium | <10 | <10 | >30 |
Urine/plasma creatinine | >30:1 | >30:1 | <20:1 |
Urine/plasma osmolarity | U Osm > P Osm | U Osm > P Osm | U Osm = P Osm |
Urine sediment | normal | Unremarkable except bile pigmented casts | Muddy brown casts, cellular debris |
Hepatorenal Syndrome
Hepatorenal syndrome (HRS) represents the end stage of a sequence of reductions in renal perfusion induced by increasingly severe liver injury. This is marked by arterial vasodilation in the splanchnic circulation due to overproduction of nitric oxide triggered by portal hypertension. However, the changes in the renal bed are the opposite marked by increase in renal vascular resistance as a result of renin angiotensin activation in response to systemic hypotension (Ginès and Schrier 2009, Wadei et al. 2006). This leads to a reduction in glomerular filtration rate (GFR) as a result of decreased renal perfusion with a concomitant decrease in renal sodium excretion (often to less than 10 meq/day in advanced cirrhosis). Based on the rapidity of onset, two forms of HRS have been described (Ginès and Schrier 2009). Type 1 hepatorenal syndrome has a rapid onset, fast progression, characterized by oliguria and twofold increase in serum creatinine to a level greater than 2.5 mg/dL in less than 2 weeks. Type 2 Hepatorenal has slower onset, less severe renal impairment, and is clinically marked by diuretic resistant ascites. Often precipitants of this syndrome are gastrointestinal bleeding and spontaneous bacterial peritonitis. Hepatorenal syndrome is a diagnosis of exclusion after other etiologies such as prerenal azotemia, acute tubular injury, glomerulonephritis, and obstruction are ruled out first. There should also be absence of hematuria and proteinuria in these patients and a lack of improvement in renal function in response to normal saline infusion and/or Albumin administration. Since hepatorenal syndrome is considered a functional form of renal failure, patients will usually recover renal function after liver transplantation; however, they do tend to be sicker than non-HRS patients and may have a greater overall risk of developing ESRD (Gonwa et al. 1995).
Acute Tubular Injury or Necrosis
Usage of nephrotoxic medications, intra-arterial or intravenous iodinated contrast-based studies, and hemodynamic instability from bleeding or sepsis can result in acute tubular injury or necrosis (ATI/ATN). Traditional laboratory parameters used to distinguish ATI/ATN from prerenal azotemia such as fractional excretion of sodium above 2 % in tubular injury and <1 % in prerenal azotemia may not be accurate since it is possible this value may be <1 % in cirrhotic patients who have persistent renal ischemia as a result of hepatic disease (Diamond and Yoburn 1982). The urinalysis also may be misrepresentative since granular and epithelial cell casts may be seen with marked hyperbilirubinemia alone and not necessarily representative of ATN. Post liver transplantation, recovery of ATN can often be delayed due to recurrent renal injury during perioperative period and use of calcineurin inhibitors which promote persistent renal vasoconstriction especially in native kidneys with intact autonomic regulation and therefore lead to delayed regeneration of renal epithelial cells. CNI usage has also been incriminated in inhibiting proliferation of renal epithelial cells in dose-dependent manner (McCauley et al. 1991). Dialysis-dependent acute tubular necrosis pre or post liver transplantation may also be complicated by the bouts of intermittent hypotension occurring during hemodialysis which may impede renal recovery. It is therefore important to maintain mean arterial pressures above 60–65 mmHg and avoid aggressive volume removal over short periods of time on dialysis.
Glomerular Disease and Liver Failure
Many liver transplant patients have liver diseases which may be associated with glomerulonephritis (GN) and chronic renal insufficiency (Table 3). Hepatitis B has been associated with membranoproliferative GN (MPGN), membranous GN, and polyarteritis nodosa (Johnson and Couser 1990, Lai and Lai 1991). Glomerular diseases associated with Hepatitis C virus infection include Mixed cryoglobulinemia, membranous nephropathy, and Polyarteritis nodosa (PAN) (Davis et al. 1994, Misiani et al. 1992). Finally, in liver failure, secondary Ig A nephropathy due to impaired removal of Ig A containing complexes by the Kupffer cells predisposes to Ig A deposits in the kidney (Amore et al. 1994) with deposits also noted in skin and hepatic sinusoids (Van de Wiel et al. 1988). Adults usually have no clinical manifestations of glomerular disease (Pouria and Feehally 1999) while up to one third children may have asymptomatic hematuria or proteinuria (Noble-Jamieson et al. 1992). It is postulated that the lack of symptomatic presentation may be due to absence of concomitant IgG deposition which may minimize activation of complement and other inflammatory mediators (Emancipator 1990). Apparently, restoration of normal hepatic function after liver transplantation is adequate to allow dissipation of these deposits from the kidney and other sites and therefore IgA deposits on renal biopsies before liver transplantation can generally be viewed as a relatively benign finding.
Table 3
Common causes of AKI and chronic kidney disease in liver failure patients
Glomerular/vascular (acute or chronic) | Hepatitis B and C resulting in membranous or MPGN Type II mixed cryoglobulinemia Secondary Ig A nephropathy Hemochromatosis, sickle cell disease Polyarteritis nodosa |
Other causes of acute kidney injury | Prerenal azotemia Acute tubular necrosis (hypotension, sepsis, mushroom poisoning, myoglobin, hemoglobin, bilirubin cast nephropathy, contrast agent) Hepatorenal syndrome |
Non cirrhotic liver diseases with CKD | Methylmalonic aciduria Familial non-neuropathic amyloidosis Primary oxalosis Atypical hemolytic uremic syndrome (HUS) |
Un related CKD with liver disease | Hypertension Diabetes mellitus |
Noncirrhotic Liver Disease and Chronic Kidney Disease
These include metabolic disease such as methylmalonic aciduria, familial non-neuropathic amyloidosis, primary oxalosis, and atypical hemolytic uremic syndrome (HUS). These patients are usually referred for simultaneous liver and kidney transplantation with MELD exception. Primary oxalosis and Atypical HUS are outlined below.
Primary Oxalosis
Primary hyperoxaluria (PH) is a rare autosomal recessive inborn error of glyoxylate metabolism characterized by overproduction of oxalate, which is deposited as calcium oxalate in various organs. The kidney is a principal target for oxalate deposition resulting in recurrent stones and progressive nephrocalcinosis which may progress to ESRD. Type 1 PH accounts for 80 % of all cases and is characterized by decreased or absent activity of hepatic peroxisomal enzyme: alanine glyoxylate aminotransferase (AGT) which leads to increased glyoxalate pool and production of oxalate. When the GFR falls below 30–40 mL/min/1.73 m2, combination of oxalate overproduction and reduced urinary oxalate excretion results in systemic oxalosis with deposition in the heart, blood vessels, joints, bone, and retina. Combined liver and kidney transplantation has increasingly become the treatment used in patients with PH type 1 with progressive renal disease (Bergstralh et al. 2010) since the transplanted liver provides the missing enzyme. The outcome of simultaneous transplantation is probably best when the procedure is performed as the glomerular filtration rate (GFR) falls to 25 mL/min/1.73 m2 and prior to marked tissue oxalate deposition.
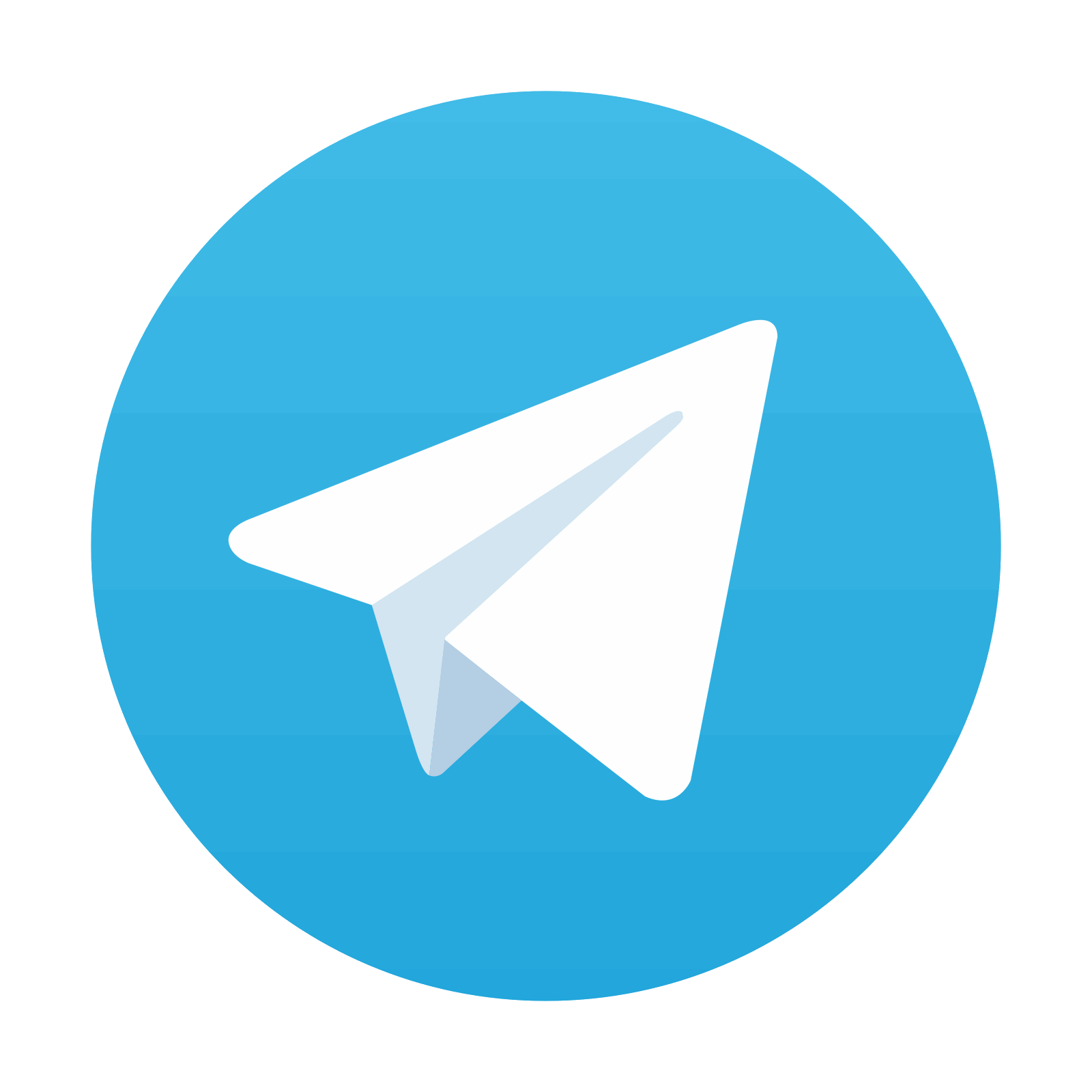
Stay updated, free articles. Join our Telegram channel

Full access? Get Clinical Tree
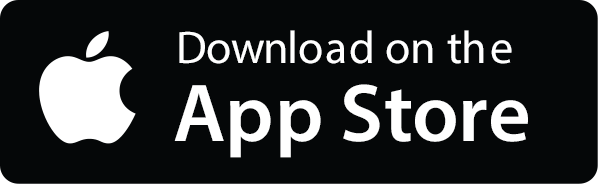
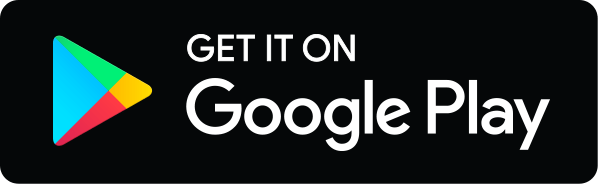