Abstract
The liver is a multifunctional organ that is involved in a number of critical excretory, synthetic and metabolic functions. Biochemical assessment of these functions in children is undertaken by utilizing a number of tests performed in clinical laboratories. Many of the most commonly utilized serum chemistry tests, like aminotransferase and alkaline phosphatase levels, are often referred to as liver function tests (LFTs), which is a misnomer, as these do not actually measure or indicate liver function. Rather, these tests should be referred to as liver enzyme tests, reserving the term LFTs to refer to measures of hepatocyte synthetic function such as international normalized ratio (INR), prothrombin time (PT) or serum albumin. Any single biochemical test provides limited information, which must be placed in the context of the entire clinical and historic picture. Currently, the most commonly available laboratory evaluative tests of the liver are used to: (1) screen for and document liver injury; (2) identify the type or pattern of liver disorder and the site of injury; (3) prognosticate and follow-up children with chronic liver disease; and (4) serially monitor the course of liver disease, evaluate the response to treatment and adjust a treatment regimen, when appropriate. Tests to evaluate liver disease can be divided into five categories (Table 7.1)
The liver is a multifunctional organ that is involved in a number of critical excretory, synthetic and metabolic functions. Biochemical assessment of these functions in children is undertaken by utilizing a number of tests performed in clinical laboratories. Many of the most commonly utilized serum chemistry tests, like aminotransferase and alkaline phosphatase levels, are often referred to as liver function tests (LFTs), which is a misnomer, as these do not actually measure or indicate liver function. Rather, these tests should be referred to as liver enzyme tests, reserving the term LFTs to refer to measures of hepatocyte synthetic function such as international normalized ratio (INR), prothrombin time (PT) or serum albumin. Any single biochemical test provides limited information, which must be placed in the context of the entire clinical and historic picture. Currently, the most commonly available laboratory evaluative tests of the liver are used to: (1) screen for and document liver injury; (2) identify the type or pattern of liver disorder and the site of injury; (3) prognosticate and follow-up children with chronic liver disease; and (4) serially monitor the course of liver disease, evaluate the response to treatment and adjust a treatment regimen, when appropriate. Tests to evaluate liver disease can be divided into five categories (Table 7.1):
1. Tests that detect liver injury are based on measurement of the serum level of endogenous substances released from damaged hepatocytes including alanine aminotransferase (ALT), aspartate aminotransferase (AST), and lactic acid dehydrogenase (LDH).
2. Tests that detect impaired bile flow or cholestasis are based on measurement of the serum level of endogenous substances released from damaged tissue such as alkaline phosphatase (AP), γ-glutamyltransferase (GGT), and 5′-nucleotidase (5′-NT).
3. Tests of liver synthetic capacity include the serum levels of albumin, INR, PT and partial thromboplastin time (PTT), and individual clotting factors (such as factor VII and factor V). Triglyceride, cholesterol, lipid and lipoprotein synthesis also occur in the liver.
4. Tests of hepatic excretory function are based on measurements of serum concentrations of substances metabolized and transported by the liver, including endogenously produced compounds such as bilirubin and serum bile acids, as well as the determination of the rate of clearance of exogenously administered dyes or drugs, such as indocyanine green, caffeine, lidocaine, and para-aminobenzoic acid (PABA).
5. Tests of hepatic metabolic function reflect the liver’s central role in metabolic and regulatory pathways, including the detoxification and clearance of endogenous metabolites such as ammonia. Multiple metabolic abnormalities due to specific inherited deficiencies of enzymes which reside almost exclusively in the liver such as the urea cycle defects can have a primary or secondary effect on the liver.
Endogenous substances released by damaged hepatocytes | Alanine aminotransferase (ALT) Aspartate aminotransferase (AST) Lactic dehydrogenase (LDH) |
Endogenous substances reflecting impaired bile flow (cholestasis) | Gamma-glutamyltransferase (GGT) Alkaline phosphatase (AP) 5′-nucleotidase (5′NT) Leucine aminopeptidase (LAP) Urobilinogen |
Tests based on substances synthesized by the liver | Albumin Coagulation factors Serum lipids and lipoproteins Triglycerides Cholesterol |
Tests based on substances metabolized and transported by the liver | Endogenous substances (serum bilirubin, serum bile acids) Exogenous substances (Lidocaine, caffeine, aminopyrine, para-aminobenzoic acid) |
Tests based on substances detoxified and cleared by the liver | Endogenous substances (ammonia) |
Miscellaneous specific serum tests | Serum immunoglobulins Autoantibodies Plasma and urine amino acids |
Clinical interpretation of laboratory liver chemistry test results relies heavily on the availability of reference intervals (i.e., normative values) to guide the need for subsequent investigations such as further diagnostic radiographic imaging, interventional radiology procedures, or liver biopsy. In the field of pediatric laboratory medicine, accurate age- and sex-specific reference intervals established using samples from healthy children and adolescents have been less readily available, forcing many clinical laboratories to report adult reference intervals with pediatric test results, or to utilize available pediatric reference intervals established with a small sample size, inpatient or outpatient samples, outdated methodologies, and/or inappropriate statistical procedures [2]. National and global initiatives in Canada [2], the USA [58], Germany [9, 37], Australia [68], Iran [55], China [43], Nordic countries [62], and others have established currently available pediatric reference intervals. The Canadian Laboratory Initiative on Pediatric Reference Intervals (CALIPER) has collected health information and blood samples from over 9,700 healthy community children and adolescents recruited to establish a comprehensive database of age- and sex-specific reference intervals (in accordance with Clinical and Laboratory Standards Institute (CLSI) guidelines) for over 100 biomarkers of pediatric disease, with normative values for liver biochemical tests such as AST, ALT, GGT, AP, LDH, albumin, and bilirubin, which is freely available at: www.sickkids.ca/Caliperproject/index.html and on a mobile app (CALIPER Reference App on both iTunes and Google Play) [2].
Specific limitations of liver biochemical tests must be recognized. Firstly, screening laboratory tests may lack sensitivity. That is, if a liver chemistry test is normal, it does not ensure that the patient is free of liver disease. Children with chronic liver disease can have normal serum aminotransferase levels. Secondly, these tests are not specific for liver dysfunction. For example, aminotransferase levels may be elevated in patients with a non-hepatic disorder such as a musculoskeletal condition or cardiomyopathy. Finally, liver chemistry tests rarely provide a specific diagnosis; rather, they suggest a general category of liver disorder. For example, abnormal liver biochemical tests do not distinguish viral hepatitis from autoimmune hepatitis, or delineate intrahepatic from extrahepatic etiologies of cholestasis.
The sensitivity and specificity of screening laboratory tests in the detection of liver disease may be increased when utilized as a battery [1, 40, 51]. When more than one test run within a battery is serially abnormal, then the probability of liver disease is higher. When all test results within the battery are normal, the probability of missing occult liver disease is lower. Ultimately, the clinical significance of any liver chemistry test abnormality in a child must be interpreted in the context of the clinical setting.
In this chapter, the tests most frequently used in assessing liver function and injury in children will be discussed.
Tests Based on Substances Released from Injured Liver
Aminotransferases
Concentrations of liver enzymes in plasma are widely used as indicators of liver disease in children. The serum aminotransferases (also known as transaminases), ASpartate aminoTransferase (AST) and ALanine aminoTransferase (ALT), are the liver chemistry tests most commonly in use to assess injury to hepatocytes and to identify patients with liver disease [54].
Aspartate aminotransferase is an enzyme that catalyzes the reversible transfer of the amino group from aspartic acid to the α-keto group of α-ketoglutaric acid to form oxaloacetic acid plus glutamic acid (and hence AST’s former name, Serum Glutamic Oxaloacetate Transaminase, SGOT). Similarly, ALT is the enzyme that catalyzes the reversible α-amino group transfer from the amino acid alanine to the α-keto group of α-ketoglutaric acid to yield pyruvic acid plus glutamic acid (and hence ALT’s former name, Serum Glutamic Pyruvic Transaminase, SGPT).
Aspartate aminotransferase is present as both cytosolic and mitochondrial isoenzymes, and found in high concentrations in many non-liver tissues, including heart muscle, skeletal muscle, kidney, brain, pancreas, lung, leukocytes, and red blood cells. ALT is a cytosolic enzyme that is present in highest concentrations in the liver, and to a lesser extent within skeletal muscle cells. Elevation of enzyme activities in serum results from damage to or destruction of tissues rich in the aminotransferases, or to a change in cell membrane permeability allowing AST and/or ALT to leak from damaged cells into serum. However, because of the wide tissue distribution of AST, the hepatic origin of an isolated serum AST level elevation should be confirmed by simultaneously obtaining a serum ALT level. More predominant rises in AST have been reported in fulminant echovirus infection and some metabolic diseases. A disproportionately isolated increase in serum AST level should prompt a search for evidence of hemolysis (including difficult venipuncture attempts); acute rhabdomyolysis (such as seen during a systemic viral illness); a myopathic process; myocardial disease (including undiagnosed cardiomyopathy); and recent vigorous physical activity (such as long-distance running or weight lifting). Serum haptoglobin, lactate dehydrogenase, creatine phosphokinase or aldolase levels may verify hemolysis or myopathy/muscle disease as the underlying etiology of a disproportionately elevated AST level. Finally, spuriously high serum levels of AST can be due to the presence of a macro-AST, formed by the enzyme complexing with an immunoglobulin, usually immunoglobulin G, leading to decreased clearance, similar to the confusion that can occur in the case of macroamylasemia in the assessment of pancreatic diseases.
The SAFETY (Screening ALT for Elevation in Today’s Youth) study recently demonstrated the wide variability in the upper limit of ALT used in acute care children’s hospitals in the USA. [54, 55, 56] They concluded that ALT cut-off values are set too high for reliable detection of pediatric chronic liver diseases, and proposed consideration of lowering the upper limit of normal threshold to ALT > 25 IU/L for boys and ALT > 22 IU/L for girls [58]. Extensive tables of pediatric reference values for ALT are freely available on the CALIPER database at www.sickkids.ca/Caliperproject/index.html, as well as on a mobile app (CALIPER Reference App on both iTunes and Google Play) [2].
The differential diagnosis of asymptomatic elevation of serum ALT (and/or AST) levels should consider underlying genetic diseases, including Wilson disease, alpha-1-antitrypsin deficiency, Alagille syndrome, hereditary fructose intolerance, glycogen storage disease, and ornithine transcarbamylase deficiency [32], Celiac disease [22, 42], autoimmune hepatitis, drug-induced liver injury [31], and non-alcoholic fatty liver disease (NAFLD) [48, 72, 74, 75]. After blunt abdominal trauma, parallel elevations in aminotransferase levels may provide an early clue to liver injury [35]. In the pediatric patient who has undergone liver transplantation, the differential diagnosis of elevated aminotransferase levels should include the different types of allograft rejection (hyperacute, acute cellular or chronic) [64], de novo autoimmune hepatitis [20], infection (hepatitis or intercurrent systemic), and biliary or vascular complications [43].
Serial measurements of aminotransferases are one of the important means of following the clinical activity of acute hepatitis from viral or autoimmune etiology in children, evaluating the response to immunosuppressive therapy in autoimmune hepatitis or acute cellular rejection after liver transplantation, detecting drug-induced hepatotoxicity and monitoring for progression or regression to liver injury [31]. Further understanding of the susceptibility to drug-induced liver injury requires an increased understanding of relevant mechanisms, the genetic and environmental factors influencing such mechanisms, and advances in toxicogenomics and proteomics.
Some of the highest AST and ALT levels (elevations greater than 15 times normal) are seen following an acute episode of hypoxia or hypoperfusion (shock liver), and during the course of acute viral hepatitis, medication- or toxin-induced hepatotoxicity, and autoimmune hepatitis. Ischemic and hypoxic acute liver damage is more likely in patients with concomitant clinical conditions such as sepsis or low-flow hemodynamic states [44]. There is a poor correlation between serum elevations of aminotransferases and the extent of liver cell necrosis seen on liver biopsy. Nevertheless, a rapid decline in aminotransferase levels, reflecting massive destruction and loss of viable hepatocytes, in association with increasing bilirubin levels and a coagulopathy portends a poor prognosis in the setting of the child with acute liver failure.
The value of an increased AST:ALT ratio is less relevant in pediatrics. Amongst adult alcoholic patients, often deficient in pyridoxal 5′-phosphate, the coenzyme needed for ALT synthesis, increased serum AST:ALT ratios reflect altered synthesis ratios in the liver [17]. Physiologically, impairment of functional hepatic blood flow leads to a resultant decrease in hepatic sinusoidal uptake of AST in patients with cirrhosis [40]. Pediatric experience with AST:ALT ratios is very limited; a retrospective review of 73 infants with chronic liver disease at one single center demonstrated an increase in AST:ALT ratio over a 13 month period of follow-up in those with a worse clinical outcome [57]. An AST:ALT ratio of more than four, in the appropriate clinical setting, is highly suggestive of fulminant Wilson disease [7].
Lactate Dehydrogenase
Lactate dehydrogenase (LDH) is a cytoplasmic enzyme present in many tissues. There are five isoenzymes of LDH present in the serum, which can be separated using electrophoretic techniques. The slowest migrating band predominates in the liver. The differential diagnosis of elevated serum LDH levels includes skeletal or cardiac muscle injury, hemolysis, stroke, renal infarction, as well as acute and chronic liver disease. Due to limited specificity, serum LDH levels rarely add information to that obtained from the aminotransferases alone. Uncommon clinical situations in which serum LDH levels may be diagnostically useful include the massive and transient elevation characteristic of ischemic hepatitis [12] and the sustained elevation accompanying elevated serum alkaline phosphatase levels suggesting malignant infiltration of the liver.
Enzymes that Detect Impaired Bile Flow or Cholestasis
Alkaline Phosphatase
Alkaline phosphatase (AP) is a group of isoenzymes originating in different tissues in the body that hydrolyze organic phosphate esters at alkaline pH, generating inorganic phosphate and an organic radical. The APs are true isoenzymes because they all catalyze this reaction, with differences in physicochemical properties. APs are found in several tissues, including the canalicular membrane of hepatocytes, bone osteoblasts, the brush border of enterocytes in the small intestine, proximal convoluted tubules of the kidney, the placenta and white blood cells. While the bone AP isoenzyme appears to be involved with calcification, the precise function of the liver AP isoenzyme is not known, although some postulate that it may participate in transport processes [60, 59, 76].
Normal growing children and rapidly growing adolescents, in particular, have elevations of serum AP of bone origin, with good correlation with the rate of bone growth that causes influx of enzymes from osteoid tissues. Therefore, an isolated increase in AP may not indicate hepatic or biliary disease if other liver biochemical tests are normal. Initial management may involve repeating the test, or confirming the hepatic origin with another liver chemistry test such as a GGT or 5′-nucleotidase (discussed in the next section). Interpretation of serum AP levels in children is challenging due to extensive changes with growth and puberty leading to distinct sex- and age-specific dynamics. Recently developed percentile charts for AP activity in girls and boys from birth to 18 years are available.
The differential diagnosis of increased serum AP levels [59, 60] in the absence of liver disease includes pregnancy, familial inheritance, chronic renal failure, blood group types B or O [5], and transient hyperphosphatemia of infancy (THI). THI is an apparently benign condition characterized by a marked transient and disproportional increase in serum AP lasting several weeks in the absence of any clinical, radiological, or biochemical evidence of bone or liver pathology [21, 67, 68, 76]. The rise in AP is quite dramatic, often exceeding ten times the upper limit for the laboratory, with return to normal levels within 8 to 12 weeks. Awareness of this condition will curtail unnecessary extensive investigations for hepatobiliary disease [21, 70].
A low serum AP level is seen in zinc deficiency and Wilson disease. Since zinc is a cofactor for the AP, the measured activity of serum AP will be low in zinc deficiency states accompanying intestinal disorders such as acrodermatitis enteropathica and Crohn disease. Serum AP activity can be low in the fulminant presentation of Wilson disease.
Gamma-Glutamyltransferase
Gamma-glutamyltransferase (GGT) is a microsomal enzyme that catalyzes the transfer of γ-glutamyl groups from peptides such as glutathione to other amino acids. GGT is present in the cell membranes of multiple body organs, including the kidney, pancreas, liver, spleen, brain, breast and the small intestine. Since the enzyme is found in so many tissues, including the biliary epithelium and hepatocytes, the usefulness of an elevated GGT level is limited by its lack of specificity [10]. Elevations in GGT have been described in adults with a wide range of clinical conditions including chronic alcoholism, exocrine pancreatic diseases, cardiovascular disease, heart failure, NAFLD, renal failure, chronic obstructive pulmonary disease, and diabetes [39, 69]. Because GGT does not increase in the serum of patients with bone disease or children with active bone growth, it is helpful in confirming the hepatic origin of an elevated AP level in pediatrics.
Gamma-glutamyltransferase is present in normal human serum. The newborn may have very high levels of GGT, up to five to eight times the upper limit of normal for adults [10]. In premature infants, in the first few days of life the values of GGT may be even higher than in the full-term infant. Serum values then decline rapidly in both the full-term and premature infant and reach adult normal levels by six to nine months of age [10]. The CALIPER database and mobile app provide age- and gender-specific reference values for GGT (www.sickkids.ca/Caliperproject/index.html) [2].
As with other microsomal enzymes, GGT activity is inducible by certain drugs. Hence elevated GGT levels are often found in children taking anticonvulsants such as phenobarbital and phenytoin. Valproic acid may not induce an increase in serum GGT levels, except in cases of true hepatotoxicity, making this biochemical test a good candidate for monitoring for liver injury during therapy with this anticonvulsant [15].
The highest levels of GGT are found in biliary obstruction. Serum GGT was most elevated in all children with biliary atresia, sclerosing cholangitis, paucity of intrahepatic bile ducts (Alagille syndrome), and cholestatic patients with α1-antitrypsin deficiency [47]. The presence of increased serum GGT levels in both intrahepatic and extrahepatic cholestasis is variable and cannot be used to differentiate between them.
Normal or decreased serum GGT levels in the clinical setting of persistent jaundice are utilized as a test to distinguish between the different subtypes of genetic cholestatic syndromes [74]. Progressive familial intrahepatic cholestasis (PFIC) is a general term encompassing a group of illnesses characterized by persistent and profound cholestasis without extrahepatic pathology, typically presenting in the neonatal or infancy period, and with a progressive clinical course to cirrhosis. PFIC is often divided into two broad categories: “Low-GGT PFIC,” in which serum activity of GGT is usually low (or normal), and “high-GGT PFIC,” in which the activity is above normal. In reality, occasional patients have intermediate levels and can be hard to categorize according to this criterion. While not typically correlated with genotype data, elevated serum levels of GGT are thought to distinguish PFIC type 3 (caused by mutations in ABCB4, the gene which encodes the multidrug resistance gene 3 (MDR3) protein) from the normal to low serum GGT levels more typical of PFIC type 1 (caused by mutations in ATP8B1, the gene which encodes the FIC1 protein) and PFIC type 2 (caused by mutations in ABCB11, the gene which encodes the bile salt export pump (BSEP) protein). Recent scientific advances have helped clarify the molecular basis of many of these disorders [71, 73]. Low GGT familial intrahepatic cholestasis also occurs in infants with benign recurrent intrahepatic cholestasis (BRIC) with a good long-term prognosis [47]. As considered in detail in Chapter 13, BRIC is an autosomal recessive liver disease characterized by intermittent attacks of cholestasis, which starts at any age and lasts for several weeks to months, and shares a locus (chromosome 18q21) with PFIC type 1. Low GGT cholestasis is also seen in children with defects in bile acid synthesis or conjugation, children with abnormalities of contact between liver cells, and children with abnormalities of cell organization manifest as arthrogryposis-renal dysfunction-cholestasis (ARC) syndrome.
5′-Nucleotidase
5′-Nucleotidase (5′NT) catalyzes the hydrolysis of nucleotides such as adenosine-5′-phosphate or inosine-5′-phosphate, which are unique for having the phosphate group attached to the 5′ position of a pentose sugar moiety. 5′NT is found in the liver, intestine, brain, heart, blood vessels, and endocrine pancreas. Despite this widespread tissue distribution, marked serum elevations of 5′NT levels are found almost exclusively in the setting of liver disease. In the liver, 5′NT is located in both sinusoidal and canalicular membranes, but its precise physiologic purpose is not known. Serum 5′NT levels are substantially lower in children than in adults, increase gradually with adolescence, and reach a plateau after 50 years of age [30].
Serum 5′NT levels are elevated in hepatobiliary disease such as biliary obstruction, cholestasis of various etiologies, and hepatic infiltration. The spectrum of abnormal 5′NT values may parallel that of serum AP levels, probably because both enzymes have similar locations within the hepatocyte. However, unlike AP, serum 5′NT levels typically do not increase in the presence of bone disease. Elevated 5′NT levels in the presence of high ALT and AST suggest both hepatic and biliary tree involvement as in patients with sickle cell disease [4]. The main value of 5′NT is its specificity for hepatobiliary disease when it is elevated in the non-pregnant patient, especially with a concomitantly increased serum AP level. Pregnancy also causes an increased value. Occasionally, the 5′NT may be normal while the AP is elevated, yet the phosphatase may still be of hepatic origin [30].
Leucine Aminopeptidase
Leucine aminopeptidase (LAP) catalyzes the hydrolysis of amino acids from the N-terminus of peptides and proteins, but its physiologic function is not known. The name derives from the fact that LAP reacts most readily with peptides containing leucine. LAP is found widely in human tissues, but activity is especially high in liver, particularly in biliary epithelium. Activity is increased in both pregnancy and hepatobiliary diseases, but not in bone disease. Values are the same in children and adults. LAP appears to be as sensitive in detecting obstructive biliary disease as 5′NT and AP, but also cannot differentiate among intrahepatic and extrahepatic causes of cholestasis [6]. Because of the common availability of other liver biochemical tests including AP, 5′NT, and GGT, LAP has not found its way into common clinical use.
Tests of Liver Synthetic Function
Albumin
Albumin, the principal serum protein, is synthesized only in the liver. The serum albumin level at any point in time reflects the rate of synthesis, rate of degradation and volume of distribution. Albumin is synthesized in the rough endoplasmic reticulum of hepatocytes at a rate of 150 mg/kg/day. Because serum albumin has a long half-life in the serum of approximately 20 days, low serum levels are often taken as a sign of chronic liver disease rather than acute injury. However, a patient with compensated chronic liver disease may demonstrate an abrupt decrease in serum albumin concentration during an acute illness, such as sepsis or even a flu-like minor illness. [33] [63] This is caused partly by an acute decrease in synthesis below that already present due to the parenchymal liver disease, possibly regulated by cytokines such as tumor necrosis factor and interleukin-1. Albumin synthesis is regulated by changes in nutritional status, osmotic pressure, systemic inflammation, and hormone levels. Traditionally, the biologic and therapeutic role of albumin in liver disease was attributed to its oncotic effects, but it is now understood that albumin has a wide range of other important physiologic functions such as immunomodulation, endothelial stabilization, antioxidant effects and binding multiple drugs, toxins and other molecules [63].
Hypoalbuminemia is not specific for liver disease because it also occurs in the setting of protein-losing enteropathy, chronic infection and nephrotic syndrome. In the absence of other non-hepatic etiologies, serum albumin levels can be useful in assaying hepatic synthetic function. Chronic inflammation, chronic liver disease and protein malnutrition can inhibit albumin synthesis, whereas the presence of ascites causes an increased volume of distribution.
Coagulation Disorders in Liver Disease
The liver is responsible for the synthesis of many of the procoagulant and anticoagulant proteins responsible for maintaining hemostasis. Abnormal hemostasis is a common complication of liver disease [72]. Mechanisms resulting in these defects include: (1) diminished hepatic synthesis of coagulation factors V, VII, IX, X, and XI, prothrombin and fibrinogen (reflected in prolongation of the prothrombin time); (2) dietary vitamin K deficiency due to inadequate intake or malabsorption (based on intrahepatic or extrahepatic cholestasis and intestinal malabsorption); (3) dysfibrinogenemia; (4) enhanced fibrinolysis, due to decreased synthesis of alpha2-plasmin inhibitor; (5) disseminated intravascular coagulation; and (6) thrombocytopenia due to hypersplenism. [70] While liver dysfunction is often assumed to be associated with increased bleeding risk, other factors such as sepsis, hepatorenal syndrome, hypotension, and endothelial dysfunction also contribute to this bleeding tendency, rather than isolated liver disease or cirrhosis. Global tests of clot formation, such as rotational thromboelastometry and thromboelastography may eventually have a role in the evaluation of clotting in patients with advanced liver disease, but currently lack validated target levels, especially in pediatrics [52]. As storage capacity of vitamin K in the liver is limited, depletion occurs quickly when absorption is impaired, and the PT and INR increases above normal ranges.
The PT is the time (in seconds) it takes plasma to clot after addition of tissue factors. PT assesses how well the extrinsic and common pathways of the coagulation cascade work together. However, PT results will vary according to the type of analytical system and reagents employed by the laboratory. For this reason, INR is more frequently used instead of PT, since it is a calculated ratio and thus does not change no matter the testing method. However, commonly utilized thresholds for INR correction are not supported by evidence [52].
A persistently elevated INR time in a previously well child (with evidence of liver injury on clinical assessment or liver biochemical tests) can be the single laboratory test that draws attention to the possibility of the diagnosis of pediatric acute liver failure, and early communication with a pediatric liver transplant program is strongly recommended [57]. The paradoxical findings of significant rates of hypercoagulable (35%) and hypocoagulable (20%) states in patients with fulminant liver failure [3], INR may be best used to provide information about the synthetic function of the liver, but not to assess hemorrhagic risk exclusively [28]. In children with chronic cholestatic liver disease, an elevated INR level refractory to maximal vitamin K therapy and decreasing serum albumin should raise for consideration the merits of a referral to a liver transplantation center for assessment. Factor VIII, being made in non-hepatic tissues, is helpful in differentiating the depression of clotting factor activity and prolongation of hemostasis due to severe liver disease alone (normal factor VIII) from that caused by accompanying depressed factor VIII activity from consumption (DIC) [34]. DIC is more common in children with end-stage liver failure because of the increased risk of infection from general debilitation and synthetic deficiencies of plasma proteins such as complement and opsonins normally made by the liver. The demonstrations of adverse outcomes of thrombotic events in patients with coagulopathy of liver disease suggests a rebalancing of hemostatic variables, and that INR and PT continue to provide the clinical care provider with important information about the synthetic function of the liver, but broader consultation is encouraged for assessing the risk of bleeding before an invasive and traumatic diagnostic procedure is undertaken [45].
Most patients with extrahepatic obstruction respond promptly to parenterally administered vitamin K. In patients with jaundice, the type of response to vitamin K is therefore of some value in differential diagnosis, especially because it can be surmised that parenchymal function is good if the PT or INR return to normal within 24 hours after a single parenteral injection of vitamin K. In some inherited metabolic diseases in the newborn such as tyrosinemia, there may be profound elevations in INR out of proportion to other parameters of liver dysfunction [13].
Lipids and Lipoproteins
The liver plays a central role in production and degradation of lipoproteins [18] [57]. Lipoprotein abnormalities are common in chronic cholestatic disorders of either intrahepatic or extrahepatic etiology [49]. Marked elevations in the plasma levels of cholesterol and phospholipids occur, due to the regurgitation into plasma of biliary phospholipids that produce secondary effects leading to an increase in plasma cholesterol, due to enhanced hepatic synthesis of cholesterol. The cholesterol is transported in the blood in lipoprotein X, an unusual vesicular form of lipoprotein specific to cholestasis [25]. However, in non-cholestatic liver diseases, declining lipoprotein cholesterol may also reflect deteriorating liver function and an indicator of prognosis.
In acute hepatocellular injury, levels of hepatic enzymes such as lecithin-cholesterol acyltransferase (LCAT) and triglyceride lipase (TGL) are decreased. Patients with acute liver disease have increased levels of plasma triglycerides, a decreased percentage of cholesterol esters, and abnormal electrophoretic lipoprotein patterns. Mild hypertriglyceridemia is characteristic of acute hepatocellular injury, with accumulation of triglyceride-rich low-density lipoprotein levels.
However, attempts to correlate blood lipid and apolipoprotein levels with specific causes of liver dysfunction have been unsuccessful [59]. Some investigators advocate analyses of multiple apolipoproteins and subclasses, particularly apoA-II, as a more sensitive index of liver dysfunction [46].
Tests Based on Substances Metabolized and Transported by the Liver
Bilirubin
Bilirubin is a yellow tetrapyrrole pigment produced from the breakdown of ferroprotoporphyrin IX (heme), an integral part of heme-containing proteins. Approximately 75% of total bilirubin produced comes from the heme moiety of hemoglobin released from senescent erythrocytes destroyed in the reticuloendothelial cells of the liver, spleen and bone marrow. The remaining bilirubin is produced from the premature destruction of red blood cell precursors in the bone marrow (i.e., ineffective erythropoiesis) and from the turnover and catabolism of other heme-containing proteins such as myoglobin, cytochromes and peroxidases.
Unconjugated bilirubin must be taken up into the hepatocyte and conjugated into the glucuronide form by the endoplasmic reticulum enzyme bilirubin UDP-glucuronyltransferase (bilirubin-UGT); the water-soluble bilirubin mono- and di-glucuronides are then secreted across the canalicular membrane into bile [26]. The molecular mechanisms of these processes have been delineated, with many excellent recent reviews [24, 67].
When hepatic excretion of bilirubin glucuronides is impaired, serum levels of bilirubin glucuronides increase. Bilirubin glucuronides covalently bound to serum albumin form a fourth form of bilirubin, known as delta (δ) bilirubin; δ bilirubin is identified in the fourth fraction (the first three forms of bilirubin being α, β, and γ, corresponding to unconjugated, monoconjugated and diconjugated species, respectively) to elute when bilirubin is fractionated by high-pressure liquid chromatography [74]. In both children and adults, the appearance of δ bilirubin is associated with elevations of conjugated bilirubin and not with those disorders producing unconjugated bilirubin. Albumin-bound δ bilirubin has a prolonged half-life of approximately 14 days compared with the four-hour half-life of bilirubin. The prolonged half-life of albumin-bound δ bilirubin explains why some children with reversible hepatobiliary diseases have serum bilirubin levels that decline slower than one might expect during an otherwise satisfactory clinical recovery. The increase in δ bilirubin accompanying the decrease in total serum bilirubin levels during recovery from an obstructive hepatobiliary disorder likely reflects decreased clearance of δ bilirubin because of its protein-bound nature and larger size. The percentage of δ bilirubin in jaundiced neonates is low, compared with icteric adults. This may reflect delayed maturation of the enzymatic processes which produce protein-bound bilirubin from elevated conjugated bilirubin in serum.
Unconjugated hyperbilirubinemia may result from hemolysis or in genetic diseases such as Crigler-Najjar syndrome, a rare genetic disease that presents shortly after birth, characterized by a severe or total impairment of bilirubin conjugation by the liver [19] [64] due to a deficiency of bilirubin-UGT. Diminished expression of the same enzyme is also the defect causing Gilbert syndrome, a benign, unconjugated hyperbilirubinemia occurring in up to 5% of the normal population. The term physiologic jaundice is used to describe the frequently observed jaundice in otherwise completely normal neonates, and the result of a number of factors involving increased bilirubin production and decreased excretion.
Conjugated hyperbilirubinemia (greater than 20% of the total bilirubin) indicates hepatobiliary disease and is always pathologic. It is usually accompanied by bilirubin in the urine, the presence of which can be tested quickly and cheaply by utilizing a urine dipstick. The presence of bilirubin in the urine confirms the presence of conjugated hyperbilirubinemia because unconjugated bilirubin is not excreted in urine. Bilirubinuria can appear before overt clinical jaundice. Further diagnostic evaluations in this setting should never be delayed, with an approach focused on the age-specific onset of diseases affecting the developing liver. The merits of quickly diagnosing causes amenable to specific medical therapies (such as sepsis, hypopituitarism, and galactosemia) prior to the development of co-morbid complication or to early surgical interventions (such as biliary atresia or choledochal cyst) must be emphasized. Various infectious, metabolic, toxic, genetic and anatomic causes for a mechanical obstruction to bile flow or a functional impairment of any of the many processes involved in hepatic excretory function and bile secretion must be meticulously sought [23].
Although serum bilirubin levels increase in cholestatic disorders, the magnitude of the increase does not help differentiate between intrahepatic and extrahepatic biliary disorders. Both conjugated and unconjugated bilirubins are retained in these disorders, and a wide range of elevated serum concentrations of each form of bilirubin may be observed. Cholestasis is associated with complex transcriptional and post-transcriptional alterations of hepatobiliary transporters and enzymes participating in bile formation. Extensive studies on genotype-phenotype correlations in monogenic diseases such as PFIC and BRIC facilitate diagnostics and improve the risk assessment of hepatobiliary transporter gene variants in bile transport pathophysiology [71]. Genome-wide association scans are the next step on gathering information about contributors toward polygenic (multifunctional) cholestatic diseases [38, 50].
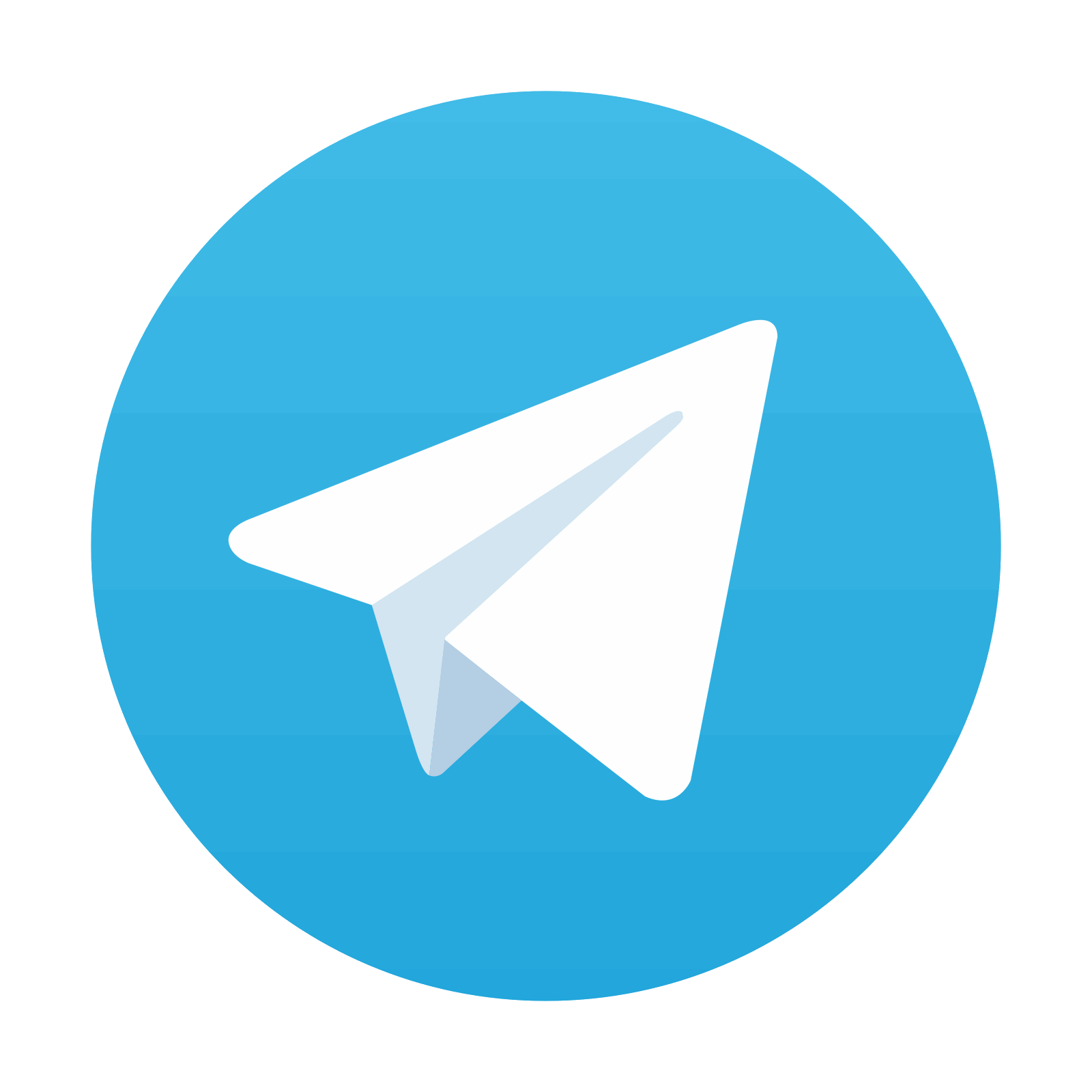
Stay updated, free articles. Join our Telegram channel

Full access? Get Clinical Tree
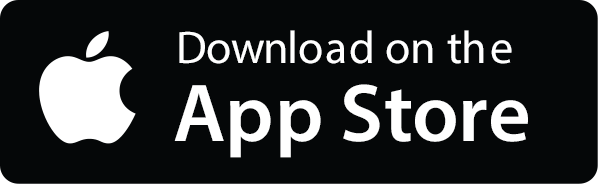
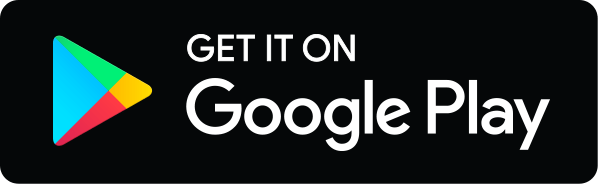
