Abstract
Elevation of the serum bilirubin level is a common finding during the first week of life. This can be a transient phenomenon that resolves spontaneously, or it can signify a serious or even potentially life-threatening condition. There are many causes of hyperbilirubinemia, and each has its own prognostic and therapeutic implications. Independent of the etiology, elevated serum bilirubin levels can be potentially toxic to the newborn infant. This chapter will review perinatal bilirubin metabolism and address assessment, etiology, toxicity, and therapy for neonatal jaundice. Finally, the diseases in which there is a primary disorder in the metabolism of bilirubin will be reviewed regarding their clinical presentation, pathophysiology, diagnosis, and treatment. For more extensive referencing, see this chapter in the third edition of this textbook [1].
Introduction
Elevation of the serum bilirubin level is a common finding during the first week of life. This can be a transient phenomenon that resolves spontaneously, or it can signify a serious or even potentially life-threatening condition. There are many causes of hyperbilirubinemia, and each has its own prognostic and therapeutic implications. Independent of the etiology, elevated serum bilirubin levels can be potentially toxic to the newborn infant. This chapter will review perinatal bilirubin metabolism and address assessment, etiology, toxicity, and therapy for neonatal jaundice. Finally, the diseases in which there is a primary disorder in the metabolism of bilirubin will be reviewed regarding their clinical presentation, pathophysiology, diagnosis, and treatment. For more extensive referencing, see this chapter in the third edition of this textbook [1].
Bilirubin Metabolism
Production and Circulation
Bilirubin (from Latin, bilis, bile; rube, red) is formed from the degradation of heme-containing compounds (Figure 12.1). The largest source for the production of bilirubin is hemoglobin. However, other heme-containing proteins are also degraded to bilirubin, including the cytochromes, catalases, tryptophan pyrrolase, and muscle myoglobin.
Figure 12.1 Chemical structures depicting the conversion of heme to bilirubin. Bilirubin is frequently represented by any of the three structures (2–4) shown at the bottom.
The formation of bilirubin is initiated by cleaving the tetrapyrrole ring of protoheme (protoporphyrin IX), which results in a linear tetrapyrrole (biliverdin). The first enzyme system involved in the formation of bilirubin is microsomal heme oxygenase. Heme oxygenase reduces the porphyrin iron (FeIII to FeII) and hydroxylates the α-methine =C-carbon. This carbon is then oxidatively excised from the tetrapyrrole ring, yielding carbon monoxide and opening the ring structure; this is associated with oxygenation of the two carbons adjacent to the site of cleavage. The cleaved α-carbon is excreted as carbon monoxide, which also functions as a neurotransmitter. The iron released by heme oxygenase can be reutilized by the body. The resultant linear tetrapyrrole is biliverdin IXα. The stereospecificity of the enzyme produces cleavage almost exclusively at the α-carbon of the tetrapyrrole.
In utero, bilirubin IXβ (cleavage between the two β-carbons, Figure 12.1) is the first bile pigment seen and can be found in bile or meconium by 15 weeks of gestation. Small amounts of bilirubin IXβ are also found in adult human bile. The central (C-10) carbon on biliverdin IXα is then reduced from a methine to a methylene group (-CH2-) forming bilirubin IXα. This is accomplished by the cytosolic enzyme biliverdin reductase. The proximity of this enzyme results in very little biliverdin ever being present in the circulation. The daily production rate of bilirubin is 6–8 mg/kg in healthy term infants and 3–4 mg/kg in healthy adults. In mammals, approximately 80% of bilirubin produced daily originates from hemoglobin. Degradation of hepatic and renal heme appears to account for most of the remaining 20%, reflecting the very rapid turnover of certain of these heme proteins. Catabolism of hemoglobin occurs very largely from the sequestration of erythrocytes at the end of their lifespan (120 days in adult humans, 80 days in newborns). A small fraction of newly synthesized hemoglobin is degraded in the bone marrow. This process, termed “ineffective erythropoiesis,” normally represents <3% of daily bilirubin production but may be substantially increased in people with hemoglobinopathies, vitamin deficiencies, and heavy metal intoxication.
Infants produce more bilirubin per unit body weight because red blood cell mass is greater and red blood cell lifespan is shorter in infants. Additionally, hepatic heme proteins represent a larger fraction of total body weight in infants. Although bilirubin has long been thought of solely as a waste product of heme catabolism, there are data to suggest that some mild degree of hyperbilirubinemia may be helpful because of the antioxidant capacity of bilirubin and its potential role as a free-radical scavenger and cytoprotectant.
Bilirubin is poorly soluble in aqueous solvents and requires biotransformation to more water-soluble derivatives for excretion from the body. This poor solubility is related to the structure of bilirubin. Rather than being linear (structure 5, Figure 12.1), bilirubin undergoes extensive internal hydrogen bonding (structure 4, Figure 12.1). This shields the polar propionic acid side-chains and makes bilirubin very non-polar and lipophilic. The carbon–carbon double bonds at positions 4–5 and 15–16 can assume two different configurations (similar to cis and trans) depending on whether the higher priority atoms or groups (based on atomic number) are on the same (Z, zusammen, German: “together”) or opposite (E, entgegen, “opposite”) sides of the double bond. The naturally occurring form of bilirubin, (4Z,15Z)-bilirubin IXα, can be represented by any of the three structures [3–5] depicted at the bottom of Figure 12.1. Knowledge of this stereochemistry is important in understanding phototherapy, the mainstay treatment for hyperbilirubinemia.
The poor aqueous solubility makes a carrier molecule, albumin, necessary for bilirubin transport from its sites of production in the reticuloendothelial system to the liver for excretion (Figure 12.2). Each albumin molecule possesses a single high-affinity binding site for one molecule of bilirubin. A binding affinity of this magnitude implies that, at normal serum bilirubin levels, all bilirubin will be transported to the liver bound to albumin, with negligible amounts free to diffuse into other tissues.
Figure 12.2 A schematic overview of bilirubin metabolism in the fetus, neonate, and adult. R: membrane carrier; GST: glutathione S-transferase (ligandin); UDPG: uridine diphosphate glucose; UDPGA: uridine diphosphate glucuronic acid; UDPNAG: uridine diphosphate N-acetyl glucosamine; P: permease; UGT1A1: bilirubin UDP-glucuronosyltransferase; NDPase: nucleoside diphosphatase; PPi: inorganic pyrophosphate; BDG/BMG: bilirubin di- or mono-glucuronide; cMOAT: canalicular multispecific organic anion transporter (also known as multidrug resistance-associated protein 2 (MRP2) and encoded by ABCC2); BG: bilirubin glucuronide.
Hepatocyte Uptake
The structure of the liver is well suited for the uptake of bilirubin by individual hepatocytes. Cords of hepatocytes are arranged radially so that adjacent sinusoids border all hepatocytes. The flow of blood through the sinusoids is slower than that of other capillary beds because it is generated by portal venous pressure rather than arterial pressure. Albumin-bound bilirubin passes from the plasma into the tissue fluid space (space of Disse) between the endothelium and the hepatocyte since the sinusoidal endothelium of the liver lacks the basal laminae that are found in other organ capillary systems. The pores of the endothelium allow direct contact with the plasma membrane of the hepatocyte.
An hepatocyte with a schematic illustration of bilirubin metabolism is shown in Figure 12.2. In the first step, bilirubin dissociates from its albumin carrier and enters the hepatocyte either via a membrane receptor carrier or by passive diffusion. The carrier in the basolateral plasma membrane, known as organic anion transporting polypeptide 2 (human OATP2 recently named OATP1B1 under new nomenclature; transporter symbol SLC21A6) also transports other bilirubin glucuronides and bromosulfophthalein. This carrier protein is competitively inhibited by simultaneous exposure to bromosulfophthalein and indocyanine green.
Once within the aqueous environment of the hepatocyte, bilirubin is again bound by a protein carrier, glutathione S- transferase, traditionally referred to as ligandin. This is a family of cytosolic proteins that have enzymatic activity and also bind non-substrate ligands. Although the affinity of purified glutathione S-transferase for bilirubin is less than that of albumin, this compound is thought to prevent bilirubin and its conjugates from refluxing back into the circulation.
Conjugation
Inside the endoplasmic reticulum (microsomes) of the hepatocyte, bilirubin is conjugated with glucuronic acid. The glucuronic acid donor is uridine diphosphate glucuronic acid. The conjugation results in an ester linkage formed with either or both of the propionic acid side-chains on the B and C pyrrole rings of bilirubin (Figure 12.3). The enzyme responsible for this esterification is bilirubin UDP-glucuronosyltransferase.
The specific isoform responsible for bilirubin conjugation is UGT1A1. This is part of the UDP glycosyltransferase super-family of enzymes encoded by UGT1 gene complex on chromosome 2 that is involved with metabolism of many xenobiotic and endogenous substances. UGT1 encodes several isoforms and has a complex structure consisting of four common exons [5] and 13 variable exons encoding different isoforms (Figure 12.4). Numerous UGT1 mutant alleles have been described which cause Gilbert syndrome (GS) and Crigler–Najjar (CN) syndrome types I and II. The isoform UGT1A1 catalyzes the formation of bilirubin mono- and diglucuronides. In normal adult humans, the majority of bilirubin conjugates are excreted in the bile as bilirubin diglucuronides (~80%) (Figure 12.5, middle panel). Lesser amounts of bilirubin monoglucuronides (~15%) are also excreted along with very small amounts of unconjugated bilirubin or other bilirubin conjugates (e.g., glucose, xylose, and mixed diesters). In infants, since there is lower UGT1A1 activity, bile contains less bilirubin diglucuronide and more bilirubin monoglucuronide than the adult (Figure 12.6B).
Figure 12.4 The human gene for UDP- glucuronosyltransferase-1 (UGT1). UDPGA: uridine diphosphate glucuronic acid.
Figure 12.5 Bile pigment excretion in the adult human as assessed by high performance liquid chromatography. Chromatograms represent analysis of serum (20 μl, (A)), duodenal bile (20 μl, (B)), and stool extract (equivalent to 50 mg of wet stool, (C)) from a normal man. Scale of y axes varies. Serum bile pigments are almost all bilirubin. The bilirubin diglucuronides (BDG) and monoglucuronides (BMG) that predominate in adult bile are not present in adult feces because of metabolism by intestinal bacteria.
Figure 12.6 Bile pigment excretion in the newborn human as assessed by high performance liquid chromatography. Chromatograms represent analysis of serum (20 μl, (A)) of an infant receiving phototherapy in the first week of life, and duodenal bile (20 μl, (B)) and stool extract (equivalent to 50 mg of wet stool, (C)) from a normal full-term, formula-fed female infant on day three of life. Serum bile pigments include lumirubin (L). Scale of y axes varies. Neonates lack an intestinal bacterial flora and, hence, large quantities of bilirubin diglucuronides (BDG), and monoglucuronides (BMG) and bilirubin (B) are present in feces. IS: internal standard.
Excretion of Bilirubin Conjugates
Following conjugation, bilirubin conjugates are excreted against a concentration gradient from the hepatocyte through the canalicular membrane into the bile. Data suggests that bilirubin glucuronides are transported across the canalicular membrane by both ATP-dependent and membrane potential-dependent transport systems. The ATP-dependent transporter responsible for bilirubin glucuronide passage from the hepatocyte through the canalicular membrane is the canalicular multi-specific organic anion transporter (cMOAT; OMIM *601107). cMOAT is a member of the ATP-binding cassette (ABC) transporter superfamily and is homologous to the multidrug resistance-associated protein (MRP2); it is also known as ABCC2 since it is encoded by ABCC2. This transporter is involved with ATP-dependent transport across the apical canalicular membrane of a variety of endogenous compounds and xenobiotics including bilirubin mono- and diglucuronide. Genetic mutations which alter these ABC transporters cause diseases which include cystic fibrosis, hyperinsulinemia, progressive familial intrahepatic cholestasis types 2 and 3, adrenoleukodystrophy, multidrug resistance, and Dubin–Johnson syndrome (DJS). This mechanism can be saturated with increasing amounts of bilirubin or bilirubin conjugates.
Under normal conditions, there is evidence that bilirubin conjugates equilibrate across the sinusoidal membrane of hepatocytes. This results in small amounts of bilirubin conjugates being present in the systemic circulation. If there is diminished hepatic glucuronidation of bilirubin (e.g., in the neonate), there will be a decreased amount of bilirubin conjugates present in the serum. In full-term newborns, there is an increase in the serum level of bilirubin diconjugates (0.55 ± 0.25% on days two to four and 1.62 ± 0.99% on days 9 to 13) that is consistent with the maturation of bilirubin glucuronidation. In contrast, in premature infants younger than 33 weeks of gestation, bilirubin diconjugates were very low and remained so, suggesting a more severe immaturity of the glucuronidation process.
In many pathologic circumstances, bilirubin mono- and diglucuronides are not excreted from the hepatocyte fast enough to prevent significant reflux back into the circulation. The resulting elevation of serum bilirubin conjugate levels results in the transesterification of bilirubin glucuronide with an amino group on albumin, producing a covalent bond between albumin and bilirubin [2]. This product is formed spontaneously and is known as δ-bilirubin. δ-Bilirubin is not formed in hyperbilirubinemic conditions unless there is elevation of the conjugated bilirubin fraction. Both δ-bilirubin and bilirubin conjugates are direct reacting in the van den Bergh test, which explains how direct bilirubin may continue to be elevated in patients who otherwise are recovering from an hepatic insult; δ-bilirubin lingers because of the long half-life (~20 day) of albumin.
Enterohepatic Circulation
When bilirubin conjugates enter the intestinal lumen (Figure 12.2), several possibilities for further metabolism arise. In adults, the normal bacterial flora hydrogenate various carbon double bonds in bilirubin to produce assorted urobilinogens (Figure 12.7). Subsequent oxidation of the middle (C-10) carbon produces the related urobilins. Since there are a large number of unsaturated bonds in bilirubin, there are many compounds formed by reduction and oxidation of these bonds. This large family of related reduction–oxidation products of bilirubin is known as the urobilinoids [3] and is excreted in the feces. The conversion of bilirubin conjugates to urobilinoids is important because it blocks the intestinal absorption of bilirubin, known as the enterohepatic circulation [4]. Neonates have a relative lack of intestinal bacterial flora and have not yet established adequate enteral feedings and motility, and thus are more likely to absorb bilirubin from the intestine. This difference in bile pigment excretion between adults and neonates is demonstrated by comparing Figures 12.5 and 12.6.
Bilirubin conjugates in the intestine can also act as substrate for either bacterial or endogenous tissue β-glucuronidase, which hydrolyses glucuronic acid from bilirubin glucuronides. The unconjugated bilirubin produced is more rapidly absorbed from the intestine [5]. In the fetus, tissue β-glucuronidase is detectable by 12 weeks of gestation and facilitates intestinal bilirubin absorption, which enables bilirubin to be cleared via the placenta. Following birth, increased intestinal β-glucuronidase can increase the neonate’s likelihood of experiencing higher serum bilirubin levels [6]. Breastmilk can contain high levels of β-glucuronidase and this is one factor related to the higher jaundice levels seen in breastfed infants [7]. Feeding specific nutritional ingredients, such as L-aspartic acid, that inhibit β-glucuronidase has been shown to result in increased fecal bilirubin excretion and lower levels of jaundice [8].
Jaundice Assessment
Jaundice (French jaune, yellow) and icterus (Greek ikteros, jaundice) both refer to the yellow discoloration of the tissues (skin, sclerae, etc.) caused by the deposition of bilirubin. Jaundice is a sign that hyperbilirubinemia exists (i.e., total serum bilirubin is approximately >1.4 mg/dL (23.8 μmol/L) after six months of age). The degree of yellowness is directly related to the level of serum bilirubin and the related amount of bilirubin deposition into the extravascular tissues. Hypercarotenemia can impart a yellow hue to the skin but the sclerae remain white. There are many conditions associated with neonatal jaundice. “Physiologic” jaundice refers to the mild and self-limited hyperbilirubinemia related to general immaturities of the bilirubin metabolism and excretion pathways as described above. Alternatively, jaundice can be a sign of severe hemolysis, infection, or liver failure.
Measurement of the total serum bilirubin concentration allows quantification of jaundice. In 2009, an expert panel of the American Academy of Pediatrics recommended that universal pre-discharge bilirubin screening, using total serum bilirubin or transcutaneous bilirubin measurements, would help to reduce risk of subsequent severe hyperbilirubinemia [9]. Two components of total serum bilirubin can be routinely measured in the clinical laboratory: conjugated bilirubin (“direct” reacting because in the van den Bergh test color development takes place directly without adding methanol), and unconjugated bilirubin (“indirect” fraction). Although the terms “direct” and “indirect” are used equivalently with conjugated and unconjugated bilirubin, it is now known that this is not quantitatively correct since the direct fraction includes both conjugated bilirubin and δ-bilirubin. Elevation of either of these fractions can result in jaundice. There is a long history of undesirable variability in the measurement of serum bilirubin fractions. The Jendrassik–Grof procedure is the method of choice for total bilirubin measurement, although this method also has problems. When the total serum bilirubin level is high, factitious elevation of the direct fraction has been reported.
Newer methods, including high performance liquid chromatography (HPLC) and multi-layered slide technology, have been developed that can more accurately determine the various bilirubin fractions (unconjugated, monoconjugated, diconjugated, and albumin bound). HPLC is superior but too expensive and time consuming for the clinical laboratory. Analysis with automated multi-layered slide technology is in use in many clinical laboratories. This allows measurement of specific conjugated and unconjugated bilirubin fractions without inclusion of δ-bilirubin. The conjugated bilirubin measurement is an earlier indicator of relief from biliary cholestasis than is direct bilirubin because of the long half-life of δ-bilirubin.
Non-invasive methods to measure jaundice levels also exist and have been shown to be particularly useful in neonates. Current commercially available methods include the BiliCheck (Respironics, Pittsburgh, PA, USA) and the Minolta/Hill-Rom Air-Shields Transcutaneous Jaundice Meter 103 (Air-Shields, Hatboro, PA, USA). The device is placed on the skin in a painless manner with the immediate point-of-care measurement of transcutaneous bilirubin that correlates well with serum bilirubin.
Neonatal Jaundice
In general, infants are not jaundiced at the time of birth. This is because of the impressive ability of the placenta to clear bilirubin from the fetal circulation. Jaundice at birth, in the rare circumstances when it is present, is typically a sign of severe disease. However, within the first few days of life, most infants develop elevated serum bilirubin levels (>1.4 mg/dL). As the serum bilirubin rises, the skin becomes more jaundiced in a cephalocaudal manner. Icterus is first observed in the head and progresses caudally to the palms and soles. Kramer found the following serum indirect bilirubin levels as jaundice progressed: head and neck, 4–8 mg/dL; upper trunk, 5–12 mg/dL; lower trunk and thighs, 8–16 mg/dL; arms and lower legs, 11–18 mg/dL; palms and soles >15 mg/dL [10]. Hence, when the bilirubin was >15 mg/dL, the entire body was icteric. However, darker skin tones can make jaundice difficult to estimate visually. At least one-third of infants develop visible jaundice. Jaundice is best observed by blanching the skin with gentle digital pressure under well-illuminated (white light) conditions, however, the data are conflicting as to whether visual detection of jaundice can be used as a sensitive tool. A combined analysis of several large studies involving thousands of infants during the first week of life showed that moderate jaundice (bilirubin >12 mg/dL) occurs in at least 12% of breast-fed infants and 4% of formula-fed infants, while severe jaundice (>15 mg/dL) occurs in 2% and 0.3% of these feeding groups, respectively [11].
In recent decades, changes in perinatal care including earlier hospital discharge after birth, have made severe neonatal jaundice a larger problem and there was a re-emergence of kernicterus. This prompted guidelines from the American Academy of Pediatrics aimed at assessing the risk of severe hyperbilirubinemia, evaluating the causes and optimizing therapy for neonatal jaundice. The American Academy of Pediatrics updated their 1994 guidelines [12] for the management of hyperbilirubinemia in newborn infants in 2004 [13] and an expert panel further clarified these recommendations in 2009 [9].
Jaundice can be caused by increased bilirubin production, decreased bilirubin excretion or a combination of these mechanisms:
increased production of bilirubin
fetal–maternal blood group incompatibilities
extravascular blood in body tissues
polycythemia
red blood cell abnormalities (hemoglobinopathies, membrane and enzyme defects)
induction of labor
decreased excretion of bilirubin
increased enterohepatic circulation of bilirubin
breast-feeding
inborn errors of metabolism
hormones and drugs
prematurity
hepatic hypoperfusion
cholestatic syndromes
obstruction of the biliary tree
combined increased production and decreased excretion of bilirubin
sepsis
intrauterine infection
congenital cirrhosis.
Figure 12.8 presents a clinical approach to assess these diagnoses. The term “physiologic jaundice” has been used to describe the frequently observed jaundice in otherwise completely healthy neonates. Physiologic jaundice is the result of a number of factors involving increased bilirubin production and decreased excretion. Jaundice should always be considered as a sign of a possible disease and not assumed to be physiologic. Specific characteristics of neonatal jaundice to be considered abnormal until proven otherwise include [1] development before 36 hours of age; persistence beyond ten days of age; serum bilirubin >12 mg/dL at any time; and elevation of the direct reacting fraction of bilirubin (>0.5–1.0 mg/dL or 10% of the total serum bilirubin) at any time [14, 15].
There are a number of epidemiologic risk factors related to neonatal jaundice. Some of the factors associated with increased neonatal bilirubin levels are male sex, low birth weight, prematurity, certain ethnicities (Asian, American Indian, Greek), maternal medications (e.g., oxytocin, promethazine hydrochloride), premature rupture of the membranes, increased weight loss after birth, delayed meconium passage, breast-feeding, and neonatal infection. Any accumulation of blood in the infant, such as a cephalohematoma related to vacuum extraction or birth trauma, increases the risk of neonatal jaundice due to increased heme degradation. Data suggest pancuronium is associated with an increased risk of hyperbilirubinemia. There is a close correlation between umbilical cord serum bilirubin level and subsequent hyperbilirubinemia. Maternal serum bilirubin level at the time of delivery, and transplacental bilirubin gradient, also correlate positively with neonatal serum bilirubin concentrations. Other factors are associated with decreased neonatal bilirubin levels including black race, exclusive formula feeding, gestational age 41 weeks, maternal smoking and certain drugs given to the mother (e.g., phenobarbital).
Neonatal Jaundice Caused by Increased Production of Bilirubin
Isoimmunization
The most common cause of severe early jaundice is fetal–maternal blood group incompatibility, with resulting isoimmunization. Maternal immunization develops when erythrocytes cross from fetal to maternal circulation. Fetal erythrocytes carrying different antigens are recognized as foreign by the maternal immune system, which then forms antibodies against them (maternal sensitization). These antibodies (immunoglobulin (Ig) G) cross the placental barrier into the fetal circulation and bind to fetal erythrocytes. In rhesus (Rh) incompatibility, sequestration and destruction of the antibody-coated erythrocytes takes place in the reticuloendothelial system of the fetus. In ABO incompatibility, hemolysis is intravascular, complement mediated, and usually not as severe as in Rh disease. Significant hemolysis can also result from incompatibilities between minor blood group antigens (e.g., Kell). Although hemolysis is predominantly associated with elevation of unconjugated bilirubin, the conjugated fraction can also be elevated.
Rhesus incompatibility problems do not usually develop until the second pregnancy or after exposure of the maternal immune system to fetal erythrocytes by another manner, such as trauma. Therefore, prenatal blood typing and serial testing of Rh-negative mothers for the development of Rh antibodies provide important information to guide possible intrauterine care. If maternal Rh antibodies develop during pregnancy, potentially helpful measures include serial amniocentesis (with bilirubin measurement), ultrasound assessment of the fetus, intrauterine transfusion, and premature delivery. The prophylactic administration of anti-D gammaglobulin (RhoGam) has been most helpful in preventing rhesus sensitization. The newborn infant with Rh incompatibility presents with pallor, hepatosplenomegaly, and a rapidly developing jaundice in the first hours of life. If the problem is severe, the infant may be born with generalized edema (fetal hydrops). Laboratory findings in the neonate’s blood include reticulocytosis, anemia, a positive direct Coombs test, and a rapidly rising serum bilirubin level. Exchange transfusion continues to be an important therapy for seriously affected infants. Intravenous gammaglobulin has been shown to reduce the need for exchange transfusions in both Rh and ABO hemolytic disease.
Clinically, ABO incompatibility usually presents with the first pregnancy. Development of ABO hemolytic disease is largely limited to blood group A or B infants born to group O mothers. Development of jaundice is not as rapid as with Rh disease and a serum bilirubin >12 mg/dL on day three of life is typical. Laboratory abnormalities include reticulocytosis (>10%), increased spherocytes, and a weakly positive direct Coombs test, although this is sometimes negative. Anti-A or anti-B antibodies may be seen in the serum of the newborn if examined within the first few days of life before they rapidly disappear.
Extravascular Blood
Extravascular blood within the body (e.g., cephalohematoma, ecchymoses, petechiae, and hemorrhage) can be rapidly metabolized to bilirubin by tissue macrophages. Although the diagnosis can often be made on physical examination, occult intracranial, intestinal, or pulmonary hemorrhage can also produce hyperbilirubinemia. Similarly, swallowed blood can be converted to bilirubin by the heme oxygenase of intestinal epithelium. The Apt test can be used to distinguish blood of maternal or infant origin because of differences in alkali resistance between fetal and adult hemoglobin.
Polycythemia
Polycythemia can cause hyperbilirubinemia because the absolute increase in red cell mass results in elevated bilirubin production through normal rates of erythrocyte breakdown. A number of mechanisms may result in neonatal polycythemia (usually defined by venipuncture hematocrits >65%). In general, polycythemia can be due to actively increased RBC production by the fetus or newborn or due to passive transfusion of RBCs into the fetal or neonatal circulation. Delayed cord clamping and twin–twin transfusions can result in polycythemia. Similarly, intrauterine hypoxia and maternal diseases such as diabetes mellitus can result in neonatal polycythemia. Therapy for symptomatic polycythemia is partial exchange transfusion, although therapy for asymptomatic polycythemia remains controversial.
Red Blood Cell Abnormalities
A number of specific abnormalities related to the red blood cell can result in neonatal jaundice, including hemoglobinopathies, red blood cell membrane and enzyme defects. Hereditary spherocytosis is not usually a neonatal problem but hemolytic crises can occur and present with a rising bilirubin level and a falling hematocrit. A family history of spherocytosis, anemia, or early gallstone disease (before age 40) is helpful in suggesting this diagnosis. The characteristic spherocytes seen in the peripheral blood smear may be impossible to distinguish from those seen with ABO hemolytic disease. Other hemolytic anemias associated with neonatal jaundice include drug-induced hemolysis, deficiencies of the erythrocyte enzymes (glucose-6- phosphate dehydrogenase (G6PD), pyruvate kinase, and others), and hemolysis induced by vitamin K (toxicity more commonly seen with vitamin K3 as opposed to naturally occurring K1 which is the preferable form for newborn vitamin K injection) or bacteria. Alpha-thalassemia can result in severe hemolysis and lethal hydrops fetalis. Gamma-beta thalassemia may also present with hemolysis and severe neonatal hyperbilirubinemia. There are a wide variety of clinical findings associated with the thalassemias, extending from profound intrauterine hydrops and death to mild neonatal jaundice and anemia, to no jaundice or anemia. Southeast Asian ovalocytosis has been associated with severe hyperbilirubinemia. These red blood cell abnormalities are more likely to result in hyperbilirubinemia in the presence of GS as described later in this chapter. Drugs or other substances responsible for hemolysis can be passed to the fetus across the placenta or to the neonate via breast milk.
Induction of Labor with Oxytocin
Induction of labor with oxytocin has been shown to be associated with neonatal jaundice. There is a significant association between hyponatremia and jaundice in infants of mothers who received oxytocin to induce labor. The vasopressin-like action of oxytocin prompts electrolyte and water transport such that the erythrocyte swells and increased osmotic fragility and hyperbilirubinemia can result.
Neonatal Jaundice Caused by Decreased Excretion of Bilirubin
Increased Enterohepatic Circulation of Bilirubin
Increased enterohepatic circulation of bilirubin is believed to be an important factor in neonatal jaundice. Neonates are at risk for the intestinal absorption of bilirubin because their bile contains increased levels of bilirubin monoglucuronide, which allows easier conversion to bilirubin; they have significant amounts of β-glucuronidase within the intestinal lumen, which hydrolyses bilirubin conjugates to bilirubin that is more easily absorbed from the intestine; they have a relative lack of intestinal flora to convert bilirubin conjugates to urobilinoids; and meconium contains significant amounts of bilirubin. Conditions which prolong meconium passage (e.g., Hirschsprung disease, meconium ileus, meconium plug syndrome) are associated with hyperbilirubinemia. The enterohepatic circulation of bilirubin can be blocked by the enteral administration of compounds that bind bilirubin such as agar, charcoal, and cholestyramine.
Breast-Feeding
Breast-feeding has been clearly identified as a factor related to neonatal jaundice. Breast-fed infants have significantly higher serum bilirubin levels than formula-fed infants on each of the first five days of life, and this unconjugated hyperbilirubinemia can persist for weeks to months. Jaundice during the first week of life is sometimes described as “breast-feeding jaundice” in order to differentiate it from “breast-milk jaundice syndrome,” which occurs after the first week of life. The former is frequently associated with inadequate breast milk intake resulting in slow intestinal motility and dehydration, whereas the latter generally occurs in otherwise thriving infants. There is probably overlap between these conditions and physiologic jaundice. There are conflicting data regarding efforts to attribute this jaundice to increased lipase activity in the breast milk, resulting in elevated levels of free fatty acids, which could inhibit hepatic UGT1A1. It has been suggested that the enterohepatic circulation of bilirubin can be facilitated by the presence of β-glucuronidase or some other substance in human milk. In a mouse model in which mouse Ugt1a1 was replaced with the human gene, human breast milk but not formula feeding appeared to suppress intestinal IκB kinase α and β, resulting in inactivation of nuclear factor-κB and loss of production of intestinal UGT1A1, thereby exacerbating hyperbilirubinemia [11]. Activation of the intestinal xenobiotic nuclear receptors PXR and CAR contribute to the induction of UGT1A1. Other factors possibly related to jaundice in breast-fed infants include caloric intake, fluid intake, weight loss, delayed meconium passage, different intestinal bacterial flora, and inhibition of UGT1A1 by an unidentified factor in the milk. It has been suggested that a healthy breast-fed infant with unconjugated hyperbilirubinemia but with normal hemoglobin concentration, reticulocyte count, and blood smear, plus no blood group incompatibility and no other abnormalities on physical examination, may be presumed to have early breast-feeding jaundice. Since there is no specific laboratory test to confirm a diagnosis of breast-milk jaundice, it is important to rule out treatable causes of jaundice before ascribing the hyperbilirubinemia to breast milk. Some infants with presumed breast milk jaundice exhibit elevated serum bile acid levels, suggesting mild hepatic dysfunction or cholestasis, although in general this is not the case. Waiting until the serum bilirubin level reaches 15 mg/dL before evaluating an otherwise well appearing breast-fed infant is an approach [9]. Breast-fed infants who are fed specific nutritional ingredients, such as L-aspartic acid, that inhibit β-glucuronidase [16] excrete more fecal bilirubin and have lower levels of jaundice than breast-fed infants who receive no supplements. No commercial preparations of these specific ingredients are presently available.
Hormones
Various hormone dysfunctions may cause development of neonatal unconjugated hyperbilirubinemia. Congenital hypothyroidism can present with serum bilirubin >12 mg/dL prior to the development of other clinical findings. Prolonged jaundice is seen in one-third of infants with congenital hypothyroidism. Similarly, hypopituitarism and anencephaly may be associated with jaundice caused by inadequate thyroxine, which is necessary for hepatic clearance of bilirubin.
Drugs
Certain drugs may affect the metabolism of bilirubin and result in hyperbilirubinemia or displacement of bilirubin from albumin. Such displacement increases the risk of kernicterus and can be caused by sulfonamides [17], moxalactam, and ceftriaxone (independent of its sludge-producing effect). The popular Chinese herb Chuen-Lin, given to 28–51% of Chinese newborn infants, has been shown to have a significant effect in displacing bilirubin from albumin. Pancuronium bromide and chloral hydrate have been suggested as causes of neonatal hyperbilirubinemia. Drugs or herbs given to someone with G6PD-deficiency that trigger a hemolytic episode (fava bean, menthol-containing substances, henna, etc.) can also induce jaundice.
Maternal Diabetes
Infants of diabetic mothers have higher peak bilirubin levels and a greater frequency of hyperbilirubinemia than normal neonates. While polycythemia is one possible mechanism, other potential reasons for this hyperbilirubinemia include prematurity, substrate deficiency for glucuronidation (secondary to hypoglycemia), and poor hepatic perfusion (secondary to respiratory distress, persistent fetal circulation, or cardiomyopathy).
Lucey–Driscoll Syndrome
The Lucey–Driscoll syndrome consists of neonatal hyperbilirubinemia within families in whom there is in vitro inhibition of UGT1A1 by both maternal and infant serum. It is presumed that this is caused by gestational hormones.
Prematurity
Jaundice is even more common in preterm infants, with the majority of these infants needing treatment with phototherapy during the first week of life [18]. The mechanism for exaggerated jaundice in this group of patients is due to an increase in bilirubin production, decreased hepatic uptake and conjugation, and increased enterohepatic circulation [19]. Hepatic UGT1A1 activity is markedly decreased in premature infants and rises steadily from 30 weeks of gestation until reaching adult levels 14 weeks after birth [20]. Preterm infants are thought to be more vulnerable to bilirubin toxicity at lower levels of unconjugated bilirubin [18, 21]. The term “low bilirubin kernicterus” refers to bilirubin encephalopathy seen in preterm infants who only demonstrated modestly elevated peak bilirubin levels [22]. Despite concerns for bilirubin toxicity at younger gestational ages (GA), there is no clear evidence to guide the clinical management of jaundice in preterm infants [18].
Hepatic Hypoperfusion and Liver Disease
Hepatic hypoperfusion can result in neonatal jaundice. Inadequate perfusion of the hepatic sinusoids may not allow sufficient hepatocyte uptake and metabolism of bilirubin. Causes include patent ductus venosus (e.g., with respiratory distress syndrome), congestive heart failure, and portal vein thrombosis. Other specific liver diseases described elsewhere in this text can result in neonatal jaundice.
In neonatal diseases with jaundice caused by increased production of bilirubin and decreased excretion, both conjugated and unconjugated bilirubin fractions can be elevated. Bacterial sepsis increases bilirubin production by bacterial hemolysin release, which promotes erythrocyte hemolysis. Endotoxins released by bacteria can also decrease canalicular bile formation.
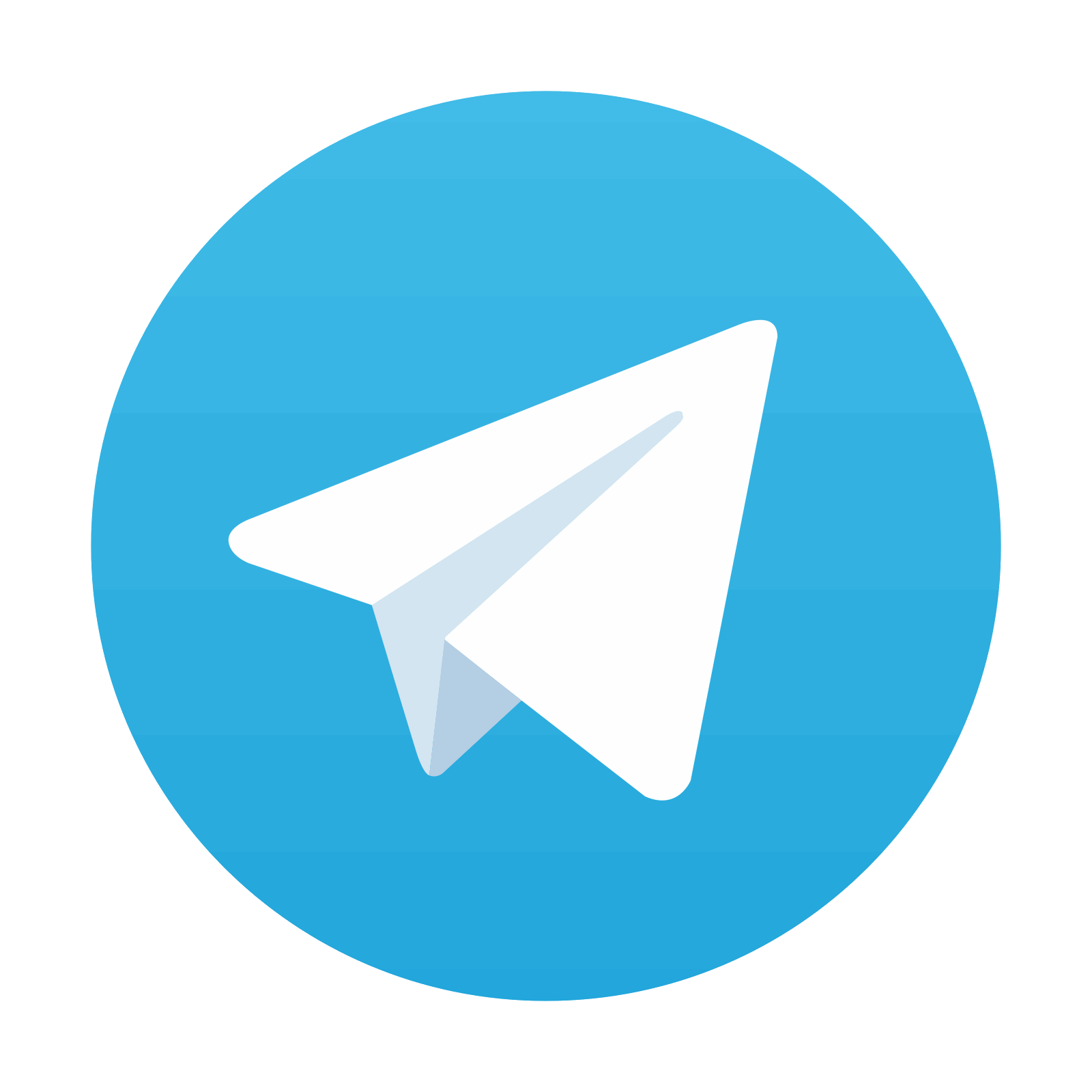
Stay updated, free articles. Join our Telegram channel

Full access? Get Clinical Tree
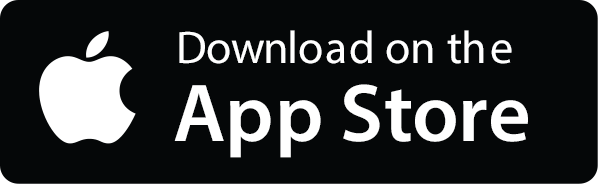
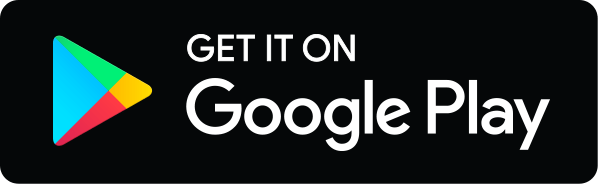
