Abstract
The liver is the largest parenchymal organ in the body and has a complicated circulatory system, with dual afferent blood supply from the portal vein and hepatic artery. Although the liver mass accounts for only 2.5% of adult body weight, it receives nearly 25% of the resting cardiac output and at any given time contains 10–15% of total body blood volume. In addition to serving as an important blood volume reservoir, the liver is also a complex metabolic organ with important functions in biosynthesis, innate immunity, metabolism and toxin/metabolite clearance. By virtue of its size, multiple metabolic functions, and prominent position in the circulatory system, the liver is frequently involved, either as an accomplice or as an innocent bystander, in a range of systemic, circulatory and inflammatory disorders. This chapter reviews hepatic involvement in common, and some uncommon, systemic diseases affecting children and young adults.
Introduction
The liver is the largest parenchymal organ in the body and has a complicated circulatory system, with dual afferent blood supply from the portal vein and hepatic artery. Although the liver mass accounts for only 2.5% of adult body weight, it receives nearly 25% of the resting cardiac output and at any given time contains 10–15% of total body blood volume. In addition to serving as an important blood volume reservoir, the liver is also a complex metabolic organ with important functions in biosynthesis, innate immunity, metabolism and toxin/metabolite clearance. By virtue of its size, multiple metabolic functions, and prominent position in the circulatory system, the liver is frequently involved, either as an accomplice or as an innocent bystander, in a range of systemic, circulatory and inflammatory disorders. This chapter reviews hepatic involvement in common, and some uncommon, systemic diseases affecting children and young adults.
Hepatic Dysfunction in the Intensive Care Unit
Hepatic dysfunction is a common occurrence in the intensive care unit, affecting up to one-third of non-cirrhotic adults [1]. Severe shock (that requiring significant pressor support), sepsis, use of mechanical ventilation (specifically positive end-expiratory pressure ventilation), and major surgery are independent risk factors for hepatic dysfunction in critically ill patients [1]. In low-flow and hypoxic states, the liver is at particular risk because selective splanchnic vasoconstriction results in decreased inflow via the portal vein. Normally the hepatic artery buffer response can partially compensate for decreased portal flow; however, this is an energy-dependent process and becomes markedly less efficient with severe or prolonged hypoperfusion [2]. In addition, high-dose systemic vasopressors (dopamine, epinephrine, and norepinephrine) reduce total liver blood flow by up to 50% and decrease the ratio of hepatic oxygen supply to consumption, delivering a second hit to the liver.
The association between hepatic dysfunction and mechanical ventilation can be ascribed to the impact of positive inspiratory pressure and positive end-expiratory pressure on portal vein and hepatic artery hemodynamics, increased right-sided heart pressure, and increased intrahepatic resistance [3]. In addition to direct effects on hepatic blood flow and metabolism, the above mechanisms of liver injury simultaneously activate the inflammatory cascade, which itself has multiple effects on hepatic function. Although the causes are multifactorial, acute hepatic dysfunction in the intensive care unit generally presents in one of two clinical patterns – sepsis-associated cholestasis or hypoxic hepatitis.
Sepsis-Associated Cholestasis
Systemic infection, even in the absence of shock, is frequently associated with hepatic dysfunction. This pattern of hepatic dysfunction in this setting is predominantly a cholestatic one, with serum bilirubin levels elevated out of proportion to changes in other biochemical markers. Multiple pathways contribute to sepsis-associated cholestasis, including increased bilirubin production (hemolysis, gastrointestinal bleeding, and resorption of hematomas), decreased intrahepatic bilirubin processing (shock, pharmacologic effects, use of total parenteral nutrition) and/or decreased bilirubin excretion (sepsis, biliary obstruction). Classic sepsis-associated cholestasis occurs in the context of gram-negative bacteremia. While lipopolysaccharide (i.e., endotoxin), the macromolecule derived from gram-negative bacterial cell walls, is a potent initiator of local and systemic inflammation, cholestasis in association with systemic infection can be triggered by any infectious or inflammatory state.
In the setting of systemic infection, the liver participates as both a perpetrator and a victim. The systemic inflammatory response syndrome, whether triggered by sepsis or another insult, prompts the liver to increase production and release of a group of circulating proteins known as acute phase proteins. The goal of these is to minimize tissue damage, promote tissue repair, isolate and neutralize invading pathogens, and prevent further pathogen entry. These proteins include fibrinogen, C-reactive protein, complement factors C3 and C9, haptoglobin, ceruloplasmin, α2-macroglobulin, CD14, α1-antichymotrypsin, α1-cysteine proteinase inhibitor, α1-antitrypsin, and lipopolysaccharide-binding protein. This acute phase response is initiated when injury at an extrahepatic site prompts local monocytes, macrophages, and other immunologically active cells to release pro-inflammatory cytokines, including interleukin (IL)-1, IL-6, and tumor necrosis factor-α (TNFα) [4]. Within the liver itself, Kupffer cells, the resident macrophages, play a critical role in protecting the organism by scavenging endotoxin from the portal circulation. However, in the process these cells themselves become a major source of pro-inflammatory cytokines that can mediate hepatocellular injury. These cytokines activate STAT3/NF-κB signaling pathways within hepatocytes resulting in increased transcription of acute phase proteins. These circulating acute phase proteins are responsible for the clinical phenomena which accompany systemic infection/inflammation, including leukocytosis, fever, altered mental status, changes in lipid metabolism, decreased gluconeogenesis, cachexia, insulin resistance, and muscle weakness.
The importance of this cascade in the control of systemic infection/inflammation is illustrated in several animal models in which production of acute phase proteins is blocked. For example, with hepatocyte specific deletion of gp130, the signaling receptor of the IL-6 pro-inflammatory family of cytokines, mortality is significantly increased in a mouse model of polymicrobial sepsis, despite normal bacterial clearance [5]. The same is true in STAT-3 knock-out mice, which experienced significantly higher mortality and demonstrated decreased levels of acute phase proteins than control littermates after cecal ligation and puncture-induced peritonitis [6]. Unfortunately, the same pro-inflammatory cytokines that activate the protective arm of the acute phase response simultaneously inhibit hepatocyte and cholangiocyte transport mechanisms, including the sodium-dependent bile-salt transporter and the multispecific organic anion transporter 2, both located at the hepatocyte basolateral membrane, and the bile-salt export pump and conjugate export pump, which transport monovalent and divalent bile acids, respectively, at the canalicular membrane [7]. The end result is stagnant bile flow leading to cholestasis.
Alterations in the hepatic microvasculature also contribute to sepsis-associated cholestasis. The release of potent vasoconstrictors and physical obstruction of the hepatic sinusoids by inflammatory cells and fibrinous microthrombi lead to endothelial cell damage and hepatocyte necrosis. Circulation to the biliary tract, dependent on the most distal branches of the hepatic arterial tree, is particularly sensitive to altered intrahepatic blood flow.
Another component of the innate immune system, the neutrophil, plays a central role in the inflammatory response to systemic infection. Once neutrophils are activated, they disseminate throughout the body and can become sequestered in the capillary networks of internal organs, such as the liver. Within the liver, neutrophils can contribute to significant hepatocellular damage; however, they may also play a protective role. Sequestration of neutrophils within liver sinusoids may enhance bacterial trapping and clearance during sepsis, thus limiting the spread of infection, albeit at a cost to the liver itself [8].
A particularly severe and ominous form of sepsis-associated cholestasis is cholangitis lenta, or subacute nonsuppurative cholangitis. This unique entity has been described in association with a variety of systemic infections, particularly intra-abdominal infections, often with an indolent clinical course. Both adults and children have been reported, many of who had recently received liver transplants [9, 10]. The diagnosis of cholangitis lenta is based on classic histologic findings, including proliferation of bile ductules at the edges of portal tracts, inspissated bile within dilated bile ductules, and absence of bile accumulation in interlobular bile ducts, all in the absence of mechanical obstruction [10, 11]. This finding is associated with a high mortality rate, and suggests the presence of severe local, or systemic infection, even in the absence of an identified organism.
Hypoxic Hepatitis
The liver is unique in possessing a dual afferent blood supply, with two-thirds of hepatic blood flow comprised of partially deoxygenated blood rich in nutrients and hormones arriving from the abdominal organs via the low-pressure, low-resistance portal venous system, and the remainder compromised of well oxygenated blood delivered via the high-pressure, high-resistance hepatic artery. Of these two systems, only the hepatic artery supplies the major bile ducts, making the biliary tree particularly susceptible to altered hepatic arterial flow. As blood enters the liver through the portal triad (zone 1) and flows through the sinusoids (zone 2) to the central vein (zone 3) the concentrations of oxygen and other nutrients decrease. This oxygen-tension gradient in the hepatic lobule accounts for the increased susceptibility of pericentral hepatocytes (zone 3) to necrosis associated with hypoxia or poor perfusion.
Regulation of hepatic blood flow occurs not only through classic arterial autoregulation (the constrictive response of an artery in the setting of increased arterial pressure), but also through the hepatic arterial buffer response. This unique mechanism represents the ability of the hepatic artery to produce compensatory flow changes in response to changes in portal venous flow. These flow changes depend on the concentration of adenosine in the space of Mall, the small fluid-filled space surrounding the terminal branches of the hepatic artery and portal vein. The secretion of adenosine into the space of Mall occurs at a constant rate, while the washout of adenosine through the portal venules is dependent on portal flow. When portal vein flow is reduced, the concentration of adenosine increases, leading to dilatation of the hepatic artery, which helps to maintain consistent hepatic perfusion. Adenosine also activates sensory nerves in the space of Mall and initiates renal fluid retention (hepatorenal reflex), in an attempt to increase circulating blood volume [12, 13].
Regulation also occurs at the level of the hepatic sinusoidal network. In the healthy liver, balanced production and release of vasoconstrictors (cyclooxygenase 1 and thromboxane A2) and vasodilators (nitric oxide) by hepatic stellate cells and liver sinusoidal endothelial cells maintains vascular tone and ensures adequate blood flow [14, 15]. Disruption of this normal balance plays a role in a variety of acute and chronic liver diseases [16, 17].
When hepatic oxygen delivery is critically insufficient for hepatocyte survival (due either to inadequate circulating blood volume, inadequate oxygen concentration, or impaired cellular oxygen uptake and utilization) the result is hypoxic hepatitis. Clinically, hypoxic hepatitis is characterized by a marked and rapid elevation of serum aminotransferases within 48 hours of the initial insult. Although some definitions require serum aminotransferases of at least 20 times the upper limit of normal, hypoxic hepatitis has been histologically confirmed in patients with enzyme levels well below this limit [18]. Classically, serum aminotransferase concentrations may reach 5,000 to 10,000 IU/L, while alkaline phosphatase levels are usually normal. Initially, aspartate aminotransferase (AST) exceeds alanine aminotransferase (ALT), but as ALT declines more slowly, a reversal of this ratio is often seen as the levels decline. Serum levels of creatinine, lactate and lactate dehydrogenase are often increased. Hepatomegaly, jaundice, and coagulopathy may be present, [18]. When coagulopathy is present, the timing mirrors that of aminotransferase elevation, while bilirubin levels tend to rise after aminotransferases have peaked, and are associated with a more severe clinical course and increased risk of complications [19]. Serum concentrations of AST and ALT can be expected to decrease by at least 50% within 72 hours of the insult and return to normal within 11 days if perfusion and oxygenation are restored and urine output is normal [20].
Hypoxic hepatitis is a common cause of severe acute liver injury in hospitalized adult patients, with an incidence of 2.5–4% in adult ICUs [21, 22]. Any clinical situation or primary disease that leads to inadequate hepatic oxygen delivery can cause hypoxic hepatitis. The most common underlying disorders are acute and chronic heart failure, respiratory failure, hypovolemic shock and septic shock; less common scenarios include pericardial tamponade, prolonged seizures, heatstroke, and cardiopulmonary bypass.
Patients with chronic liver congestion are particularly susceptible to hypoxic hepatitis when perfusion is compromised. In fact, in adults, some component of hepatic congestion is considered almost a pre-requisite for hypoxic hepatitis, as arterial hypotension itself is rarely associated with a significant increase in aminotransferases [21]. Many reports document the classic clinical and histologic picture of hypoxic hepatitis in patients with chronic congestive heart disease who experience an acute event leading to decreased perfusion, often without an obvious or significant decrease in systolic blood pressure [18, 21, 22]. The increased risk of hypoxic hepatitis in this setting is likely the result of a combination of insults: decreased cardiac output, which may lead to chronic recurrent subclinical hypoxic events; splanchnic vasoconstriction from activation of the renin-angiotensin system, which may further decrease hepatic arterial blood flow; increased sinusoidal pressure; and endotoxemia as a consequence of ischemic and/or congested bowel [18].
In septic shock, decreased arterial blood flow and oxygen delivery are often compounded by microcirculatory changes mediated by endotoxin and pro-inflammatory cytokines, leading to inability of hepatocytes to effectively extract oxygen and multiplying the hypoxic damage to the liver [23].
The histology in hypoxic hepatitis is marked by centrilobular necrosis with preservation of the periportal zone; however, liver biopsy is seldom indicated in the appropriate clinical setting.
The presence of hepatic dysfunction, whether manifest as sepsis-associated cholestasis or hypoxic hepatitis, is a significant factor in prognosticating short-term outcome of critically ill patients, as reflected by the inclusion of hepatic parameters in many adult and pediatric critical illness severity scores, including the Simplified Acute Physiology Score (SAPS) II and the Sequential Organ Failure Assessment (SOFA) score. In adult patients, the presence of hypoxic hepatitis is associated with all cause early mortality of 45–49% [21, 22]. Serum bile acid concentration is an independent risk factor for 28-day mortality in critically ill adult patients, while new-onset cholestasis accompanying hypoxic hepatitis is a risk factor for one-year mortality [19, 24]. That being said, the cause of death in critically ill patients with hepatic dysfunction is unlikely to be liver failure, but instead is the underlying insult and pathophysiology that led to critical illness.
With advances in critical care and the ability to support patients through life-threatening systemic illnesses, a number of new, ICU-associated diseases are emerging, including a particularly severe form of rapidly progressive biliary tract disease termed secondary sclerosing cholangitis in critically ill patients (SSC-CIP). This disorder evolves in patients with no history of liver or biliary tract disease who require prolonged ICU admission accompanied by mechanical ventilation and vasopressor support [25]. It is believed to be a form of ischemic cholangiopathy with sloughing of the biliary epithelium leading to the formation of protein-rich bile casts. These bile casts are pathognomonic for this disorder, and can be identified early by ribbon-like intraductal filling defects visible by either ERCP or MRCP [26]. Later changes are classic of sclerosing cholangitis, with strictures and dilatations of the intrahepatic bile ducts but relative sparing of the common bile duct. Unlike sepsis-associated cholestasis, SSC-CIP has an insidious onset as patients recover from critical illness. In contrast to hypoxic hepatitis, serum aminotransferases are only mildly elevated, while gamma-glutamyl transferase, alkaline phosphatase, and eventually bilirubin are 5 to 50 times the upper limit of normal.
Sclerosing cholangitis in critically ill patients can be complicated by acalculous cholecystitis, bacterial cholangitis, liver abscesses, and nutritional failure [26]. The clinical course is unusually rapid, with progression to cirrhosis at a mean of six months after diagnosis, and a high mortality rate without liver transplantation [25].
Congenital/Acquired Heart Disease
In addition to facilitating the development of classic hypoxic hepatitis with markedly elevated serum aminotransferases and centrilobular necrosis, chronic hepatic congestion is itself associated with a distinct liver lesion marked by a spectrum of fibrotic changes ranging from mild sinusoidal fibrosis to the appearance of broad fibrous septa to frank cirrhosis (cardiac cirrhosis) [27]. Although most frequently described in association with right-sided heart failure, this lesion can also complicate ischemic heart disease, restrictive lung disease, constrictive pericarditis, pericardial effusion, and any other entity resulting in elevated central venous pressure. Clinically, liver injury due to chronic passive congestion is often silent. Biochemical abnormalities may have an insidious onset, with a profile that is predominantly cholestatic, with elevations in serum gamma-glutamyltransferase (GGT) and alkaline phosphatase levels out of proportion to elevations in serum aminotransferases.
In children, the most common causes of heart failure are congenital heart diseases. Among these, anomalies such as pulmonary atresia, ventricular septal defect and transposition of the great arteries may lead to pulmonary hypertension and chronic passive liver congestion. In addition, as new approaches for surgical and medical management of congenital heart lesions have increased the long-term survival of infants with critical complex heart disease, the long-term impact of these therapies is becoming obvious. A prime example is the development of liver disease in patients following Fontan palliation. This procedure, used in patients with single ventricle physiology, re-establishes normal systemic oxygen saturation by directly routing systemic venous return (via the superior vena cava or right atrium) to the pulmonary arteries, bypassing the sub-pulmonary ventricle. In doing so, it creates, and in fact depends upon, chronically elevated central venous pressure.
Fontan-associated liver disease (FALD) can manifest with either acute or chronic liver dysfunction. Acute dysfunction, usually around the surgery itself, represents classic hypoxic liver injury related to hypoperfusion, while long-term liver dysfunction may reflect a combination of chronic passive congestion and decreased cardiac output intrinsic to the Fontan circulation [28, 29]. While most Fontan patients are asymptomatic from a liver disease perspective and have only mild biochemical abnormalities (an elevated GGT in 40–60%), they are at significant risk of ongoing liver fibrosis and cirrhosis, with attendant complications [30]. A long-term follow-up study of liver biopsies in adolescents and young adults >10 years post-Fontan found some degree of hepatic fibrosis in >95% of clinically stable patients [31]. The most devastating complication of FALD is hepatocellular carcinoma, which is being reported with increased frequency in long-term Fontan survivors [32–34].
While time since Fontan consistently emerges as a risk factor for FALD, additional risk factors have not been clearly elucidated [31]. The best modality for disease surveillance and prognostication is also unknown. Magnetic resonance elastography (MRE) has emerged as a popular choice, as MRE-derived liver stiffness has been associated with worse hemodynamics and clinical outcomes in Fontan patients, and simultaneously allows for hepatocellular carcinoma surveillance [35]. In the absence of specific therapy for FALD, comprehensive supportive care and close surveillance for early identification of complications is paramount. In the setting of advanced liver disease or hepatocellular carcinoma, combined heart–liver transplant may be the best treatment option.
Hepatic Venous Outflow Obstruction
Effective obstruction of hepatic venous outflow can occur at several levels. When the obstruction is at the level of the hepatic sinusoids it is termed veno-occlusive disease (VOD; discussed below). Obstruction at the level of the heart is chronic passive congestion (discussed above), while obstruction to outflow at any level from the small hepatic veins to the junction of the inferior vena cava (IVC) with the right atrium constitutes Budd–Chiari syndrome. Budd–Chiari syndrome can be classified as primary when there is intraluminal obstruction by a thrombus or web, or secondary when there is extrinsic compression by an abscess, cyst or tumor.
While membranous obstruction of the hepatic veins or IVC is a common cause of Budd–Chiari syndrome in Africa and Asia, and has been described in children as well as adults, the most common cause of Budd–Chiari syndrome in western countries is hepatic vein thrombosis [36]. Thrombosis of the hepatic veins, or, less commonly, the IVC, can occur as a consequence of pro-thrombotic disorders, anti-fibrinolytic disorders, local endothelial cell damage, or some combination of these factors. Pro-thrombotic disorders in turn can be classified as either genetic or acquired.
The most common inherited thrombotic disorders associated with Budd–Chiari syndrome are the gain of function mutations in Factor V Leiden (leading to activated protein C resistance) and prothrombin/Factor II deficiency (leading to excessive prothrombin generation), and loss of anticoagulant function due to Protein C, Protein S, or antithrombin deficiencies [37, 38]. The reported prevalence of one or more of these inherited defects in patients with Budd–Chiari syndrome is variable, and often both inherited and acquired risk factors co-exist. Investigation for thrombotic risk factors in patients with established thrombosis can be challenging, as acquired deficiencies, related to the thrombosis or secondary effects, are common, and levels of Proteins C, S, and antithrombin are interpretable only when all other coagulation factors are normal.
Hyperhomocysteinemia, frequently resulting from a polymorphism in MHTR (677TT), encoding methylene tetrahydrofolate reductase, is also a risk factor for Budd–Chiari syndrome. This is particularly true in China, where a higher frequency of MHTR polymorphisms likely interacts with lifestyle factors such as smoking and alcohol use to increase the incidence of hyperhomocysteinemia [39].
Theoretically, anti-fibrinolytic disorders might also potentiate the development of Budd–Chiari syndrome. While increased plasma levels of plasminogen activator inhibitor 1 and decreased levels of thrombin-activatable fibrinolysis inhibitor, plasmin inhibitor and apolipoprotein A1 have been described in patients with Budd–Chiari syndrome, the genetic basis for these differences is not yet understood [40].
The most common acquired cause of thrombophilia is myeloproliferative disorder. Although rare, thrombocythemia, polycythemia, and thrombocytosis may all occur in children, and may be associated with a risk for venous thrombosis. Mutations in JAK2, encoding the JAK2 tyrosine kinase, have been associated with both myeloproliferative disorder and Budd–Chiari syndrome in children and adults. JAK2 gene mutations result in the production of a constitutively activated JAK2 protein, which leads to increased red blood cell survival and thrombotic risk [41–43]. The association between myeloproliferative disorders and JAK2 mutations is so strong that the mutation itself may serve as a marker for occult or evolving myelodysplasia in the setting of Budd–Chiari syndrome [44].
Other acquired risk factors for Budd–Chiari syndrome include the presence of antiphospholipid and anticardiolipin antibodies, often associated with rheumatologic conditions; other chronic inflammatory states such as Behçet disease, inflammatory bowel disease, sarcoidosis, pregnancy and the peripartum period; paroxysmal nocturnal hemoglobinuria; use of oral contraceptives; and intra-abdominal infection. Each of these factors, genetic and/or acquired, is unlikely to lead to Budd–Chiari syndrome individually. In many cases, multiple thrombophilic risk factors have been identified, and in those for whom only one risk factor was evident, an as yet unidentified predisposition may be at play.
Budd–Chiari syndrome most often presents as chronic obstruction with hepatomegaly, ascites, abdominal distension and abdominal pain. In children, lethargy and anorexia may also be present. Often, abdominal and chest wall collaterals are prominent and distended. Serum aminotransferase and bilirubin levels are generally only mildly to moderately elevated, although hypoalbuminemia and coagulopathy are present in almost half of patients [45, 46]. The histology of chronic Budd–Chiari syndrome is microscopically indistinguishable from chronic passive congestion and VOD, with zone 3 congestion, necrosis and fibrosis [45]. A liver biopsy is rarely necessary for diagnosis, as the clinical picture and imaging findings are the key.
Acute Budd–Chiari syndrome can present with similar clinical findings but is more likely to include jaundice and coagulopathy. Acute congestion leading to massive centrilobular necrosis and fulminant hepatic failure is occasionally the presenting scenario of hepatic venous outflow obstruction, particularly if it occurs in the setting of pre-existing chronic liver disease.
The first step in evaluation of a patient with suspected hepatic venous outflow obstruction is pulsed Doppler ultrasonography of the hepatic vessels and IVC. When experienced practitioners use this modality, the presence of specific findings in the hepatic or caval veins, such as stenosis, thrombosis, fibrotic cord, or insufficient recanalization of the vessel, along with caudate lobe hypertrophy, can identify Budd–Chiari syndrome with a high specificity [47, 48]. Contrast-enhanced cross-sectional imaging is the second line of investigation, and is useful for identifying indirect signs of Budd–Chiari syndrome. These include alterations in hepatic morphology and perfusion, from the classic fan-shaped enhancement of the caudate lobe and central liver to parenchymal changes consistent with cirrhosis, as well as the presence of intra- and extrahepatic collateral vessels [48, 49]. MRI and CT also allow better visualization and anatomic orientation of the mesenteric and portal vasculature for therapeutic planning. With improvements in cross-sectional imaging, hepatic venography is rarely required for the diagnosis of Budd–Chiari syndrome and tends to be used only in those cases for which endovascular therapy is indicated.
Therapy for Budd–Chiari syndrome ranges from supportive medical management of complications to liver transplantation, and depends on the site and chronicity of the obstruction, the degree of liver dysfunction, and, in children, the size of the patient. Initial therapy is conservative, emphasizing diuresis, treatment of predisposing factors and prophylactic anticoagulation to decrease the risk of additional thromboses. In patients with long-standing obstruction and evidence of either decompensated portal hypertension or synthetic dysfunction, early evaluation for liver transplantation may be the most appropriate and expedient course. In all other cases, acute or chronic, the focus is on removing or bypassing the obstruction and re-establishing normal portal and hepatic vein pressures.
While directed thrombolytic therapy of hepatic vein/IVC thrombosis has been reported, the role in managing children, either primarily or as adjunctive therapy, is not defined. In adults, percutaneous transluminal angioplasty is a mainstay of therapy; with recent data suggesting that routine stenting with angioplasty is superior to angioplasty alone at preventing restenosis [50, 51]. While there is growing experience with angioplasty in small children, the use of stents is controversial, as placement is technically more challenging, and stents carry a risk of migration as well as obstruction, mandating long-term anticoagulation.
Transjugular intrahepatic portosystemic shunting (TIPS) is the most common endovascular procedure employed for Budd–Chiari syndrome in adults, and there is growing experience using this technology in small children [46, 52]. However, open surgical procedures, including thrombosis or web resection with pericardial patch grafting, and mesocaval, splenocaval, splenoatrial and splenojugular shunts, are still commonly employed in both children and adults. Liver transplantation remains the salvage therapy for patients with inadequate response to shunting procedures or recurrent shunt thrombosis. Importantly, in patients with underlying thrombophilia, long-term anticoagulation is necessary to prevent recurrence post-transplant.
Hematologic Disorders
Hemoglobinopathies
Hepatobiliary disorders are common in children with hemoglobinopathies, and can be related to effects of the hematologic disease itself, or complications of therapy. The most common hemoglobinopathy is sickle cell anemia, in which hepatic complications are being increasingly recognized as an important cause of morbidity and mortality in adults. In adult patients admitted for acute vaso-occlusive crises, the liver is involved in up to 39% of cases. This involvement varies from acute sickle cell hepatic crisis in 10% of patients, marked by painful hepatomegaly, fever, elevated white blood cell count, and variable increases in serum aminotransferases and bilirubin, to isolated biochemical abnormalities (predominantly cholestatic and mixed cholestatic-hepatocellular) with minimal overt symptoms [53]. In children, at least one hepatobiliary complication is present in 37%, with cholelithiasis being the most common (25%), followed by acute hepatic crisis in 4% and transfusion-related iron overload in 3% [54].
Acute hepatic sequestration is a relatively uncommon complication of sickle cell disease in both children and adults. Hepatic sequestration is believed to be caused by build-up of sickled erythrocytes in the hepatic sinusoids, leading to obstruction of sinusoidal flow. Clinically it presents with right upper quadrant pain, hepatomegaly, jaundice and a fall in hemoglobin [55]. Treatment is identical to that required for splenic sequestration, with special attention to hemoglobin and hemodynamics, as the sequestered erythrocytes may not be destroyed and, on return to the systemic circulation, may lead to hypervolemia, congestive heart failure and cerebral hemorrhage. Although rare, hepatic sequestration in children can be complicated by acute liver failure and cardio-respiratory arrest [54].
A rare, but potentially fatal complication of sickle cell disease is sickle cell intrahepatic cholestasis, or sickle cell hepatopathy. This condition is thought to represent a severe form of sickle cell hepatic crisis in which intrahepatic sickling of erythrocytes leads to sludging and congestion of hepatic vascular beds, followed by tissue ischemia, widespread microscopic infarctions, and, in severe cases, liver synthetic dysfunction and secondary renal dysfunction. Sickle cell hepatopathy is differentiated from the more common hepatic crisis by the presence of significant hyperbilirubinemia, with the conjugated fraction exceeding 50% of the total bilirubin [55]. Serum aminotransferases can be markedly elevated (>1000 IU/mL) reflecting severe ischemic injury [56]. While there are no standard diagnostic criteria, one group has proposed that, in the absence of viral hepatitis, extrahepatic obstruction or hepatic sequestration, a threshold total serum bilirubin level of >13 mg/dL is diagnostic. Up to 50% of patients who meet this criterion can present with coagulopathy and/or encephalopathy. For this subgroup, the prognosis is poor. Treatment of sickle cell hepatopathy consists of supportive therapy and exchange transfusion, which should be promptly initiated in patients with severe hepatic dysfunction [57].
Patients with sickle cell disease can develop cirrhosis even in the absence of other causes of chronic liver disease. It is hypothesized that repeated microvascular occlusion results in focal necrosis and scarring leading to stellate cell activation and progressive fibrosis. In addition, many patients with sickle cell disease have evidence of a second liver disorder which might lead to fibrosis and which likely potentiates liver damage. The most common of these are viral hepatitis and secondary hemosiderosis, both related to chronic transfusion therapy.
Although historically viral hepatitis, particularly hepatitis C, was a major cause of transfusion-related hepatitis, the implementation of universal screening of blood products in the early 1990s has practically eliminated blood transfusion as a mode of transmission, especially in developed countries. On the other hand, the increased use of transfusion therapy to prevent stroke in pediatric patients with sickle cell disease, and the heavier transfusion requirements for patients with thalassemia, have increased the risks of transfusion-associated hemosiderosis. Aggressive monitoring of iron levels, both in the blood and in the liver, and institution of chelation therapy are now the standard of care for patients with hemoglobinopathies who require transfusions.
Other unusual hepatic lesions noted in sickle cell anemia are hepatic vein thrombosis, focal nodular hyperplasia, hepatic abscesses, ischemic cholangiopathy and bilomas. Impaired hepatic microcirculation may cause areas of infarction, which then act as a nidus for infection, while sickling in the terminal arterial branches that supply the bile ducts can lead to biliary strictures and obstruction [54, 56]. Infection is further facilitated by microinfarctions in the gastrointestinal epithelium, which increase intestinal permeability and allow translocation of enteric organisms while splenic dysfunction impedes clearance of bacteria.
There is limited experience with liver transplantation in patients with sickle cell disease and acute liver failure or decompensated chronic liver disease, particularly in pediatric patients [58, 59]. Variable outcomes are reported, with a high risk of vascular and infectious complications [56, 59, 60]. Peri-operative management of these patients is complex, and requires close involvement of hematologists experienced with sickle cell disease management. Importantly, liver transplantation does not alter the course of the disease, or protect the patient from ongoing injury to the liver allograft. Given the unique challenges in this population, liver transplantation for sickle cell disease should be undertaken in a small number of centers, where expertise and experience can be built.
Coagulation Disorders
Historically, transfusion-acquired hepatotropic viruses caused the hepatic complications in patients with hemophilia and other coagulation disorders. The use of recombinant products and improved screening of the blood supply has decreased this exposure, while routine administration of Hepatitis A and B vaccines have decreased the consequences of exposure. In the modern era, non-alcoholic fatty liver disease (NAFLD) may replace chronic viral infection as the most common cause of liver injury in children with hemophilia [61].
Leukemia and Lymphoma
Hepatomegaly, jaundice, and asymptomatic elevated serum aminotransferase levels frequently occur in patients with lymphoma, leukemia, and intra-abdominal solid tumors. Multiple processes contribute to these hepatic abnormalities, including tumor infiltration, intrahepatic or extrahepatic biliary obstruction, malignancy-associated hemophagocytic syndrome, opportunistic infections, and hepatotoxic drugs.
Acute lymphoblastic leukemia is the most common hematologic malignancy in childhood and is frequently associated with hepatic involvement. At the time of diagnosis, children often have abnormal liver biochemistries and 20% will have hepatomegaly and/or splenomegaly [62]. After initiation of treatment, most hepatic abnormalities in patients with acute lymphoblastic leukemia result from medication toxicity. Early in therapy, 7–20% of children have hepatic abnormalities, with >5% requiring chemotherapy dose modification. Risk factors for treatment-related hepatotoxicity include elevated body mass index and age >10 years [63, 64]. Methotrexate and 6-mercaptopurine remain mainstays of pediatric acute lymphoblastic leukemia protocols and have well characterized hepatotoxicity. One study of 243 children with acute lymphoblastic leukemia receiving oral methotrexate therapy found that two-thirds had an abnormal serum ALT at some point during treatment, and 17.6% had one or more serum ALT values >720 IU/L [65]. In spite of this, and with no modification of therapy in the presence of elevated ALT, only 16% of patients maintained an abnormal ALT after therapy was complete [65].
Another group of drugs used for acute lymphoblastic leukemia has emerged as the most frequent cause of cholestatic liver injury among antineoplastic agents. L-asparaginase and peg-asparaginase cause a unique form of hepatocellular injury in up to 20% of treated patients, with rapid onset of marked jaundice, hypoalbuminemia, mild coagulopathy, and diffuse hepatic steatosis [66]. Risk factors include overweight/obesity and Hispanic ethnicity [67]. Although liver failure is rarely reported in this setting, hepatic encephalopathy may develop, and persistent cholestasis may be treatment limiting. While the mechanism of asparaginase-related hepatotoxicity is unclear, the role of mitochondrial stress has been postulated, and several reports have documented successful treatment with levocarnitine and vitamin B complex [68, 69].
Hepatosplenomegaly is also common in acute nonlymphocytic leukemia and chronic myeloid leukemia. The chemotherapeutic agents used for these disorders are generally not recognized as significant hepatotoxins; however, as long-term therapy with tyrosine-kinase inhibitors has essentially replaced stem cell transplant in chronic myelogenous leukemia, additional hepatic complications may emerge [70].
Pediatric lymphomas, Hodgkin and non-Hodgkin types, can also be associated with a variety of hepatic complications, including tumor infiltration, fulminant hepatic failure, and vanishing bile duct syndrome. Although rare, both idiopathic cholestasis and vanishing bile duct syndrome have been reported in association with Hodgkin lymphoma, and occur in the absence of infiltrative liver disease [71, 72]. It is unclear whether these are distinct entities, or a spectrum of the same disease. The pathophysiologic basis of both is postulated to be a paraneoplastic process, with release of cholestatic cytokines from the tumor cells themselves. While the prognosis of Hodgkin-associated vanishing bile duct syndrome is generally thought to be worse than Hodgkin-associated intrahepatic cholestasis, both conditions have been reported to resolve with prompt treatment of the underlying lymphoma [71, 72]. Unfortunately, treatment is complicated and options limited in the setting of significant cholestasis or overt liver dysfunction.
Non-Hodgkin lymphoma can also be associated with vanishing bile duct syndrome, fulminant liver failure, and an acute hepatitis presentation; however, obstructive jaundice is a more common hepatic complication of non-Hodgkin disease in children [73].
Additional hepatic complications in patients with hematologic malignancies include exposure to non-chemotherapy hepatotoxic medications, radiation therapy and opportunistic infections. Pediatric cancer survivors, both hematologic and solid tumor, may develop focal nodular hyperplasia or nodular regenerative hyperplasia of the liver, both of which are thought to be caused by alterations in local hepatic blood flow. These conditions may be found incidentally, or present with complications of portal hypertension or a liver mass, which must be differentiated from recurrent malignancy.
Allogeneic Stem Cell Transplantation
Hepatic complications are common in children undergoing stem cell transplant and can be classified based on etiology (primary disease, drug-induced liver injury, infectious, vascular, parenteral nutrition-associated, immunologic), or by timing related to stem cell transplant (pre-transplant, early post-transplant, late post-transplant). A structured approach is necessary when evaluating the patient with hepatic abnormalities following stem cell transplant, with the primary goal of identifying conditions amenable to therapy. Because of the complex clinical and treatment scenarios of these patients, more than one pathologic process is frequently operant.
Liver Disease Prior to Stem Cell Transplant
The indications for stem cell transplant in children are multiple, varied, and expanding. Many of the immunodeficiency syndromes for which stem cell transplant (SCT) is indicated are associated with liver disease independent of stem cell transplantation, for example, sclerosing cholangitis associated with lymphohistiocytic syndromes and hyper-IgM syndrome, and chronic hepatic and biliary tract infections associated with severe combined immunodeficiency and chronic granulomatous disease. Other indications for pediatric SCT, such as blood dyscrasias and relapsed leukemia/lymphoma, may be associated with hepatic complications such as chronic viral hepatitis, drug-induced liver injury, and iron overload. Identification of pre-existing conditions, regardless of their etiology, and optimization of liver health is crucial prior to SCT. Patients with chronic liver disease are at higher risk for severe hepatic complications following SCT, and occasionally advanced liver disease may itself be a contraindication to SCT.
Hepatic Veno-Occlusive Disease
Hepatic veno-occlusive disease (VOD), or sinusoidal obstruction syndrome (SOS), is a potentially life-threatening complication that is associated with standard chemotherapy, radiation therapy, or most frequently, high-dose myeloablative conditioning therapy for stem cell transplant (Table 40.1). VOD/SOS is one of a number of transplant-related, systemic endothelial diseases, including acute graft vs. host disease, engraftment syndrome and transplant-associated thrombotic microangiopathy. Veno-occlusive disease with immunodeficiency has been linked to homozygous mutations in SP110, a gene encoding a PML nuclear body-associated protein. It is caused by toxic injury to the hepatic sinusoidal epithelial cells, leading to their detachment and embolization in zone 3 of the lobule with consequent outflow obstruction. In VOD/SOS, concentric narrowing of the terminal hepatic venules occurs without associated abnormalities of the larger hepatic veins or the inferior vena cava. In addition to endothelial cell destruction, direct damage to hepatocytes resulting in necrosis, and eventually fibrosis, can further liver dysfunction and contribute to liver failure, multi-organ system failure, and death [74, 75].
Pyrrolizidine alkaloids found in plants such as ragwort and grounsel Nitrosamines |
Gemtuzumab ozogamicin 6-Thioguanine |
Azathioprine |
6-Mercaptopurine |
Actinomycin D Dacarbazine Vincristine |
Oxaliplatin Cyclophosphamide Busulfan Urethane Carmustine Cytosine arabinoside (Cytaramine, Ara-C) |
Indicine N-oxide |
Cysteamine |
Estrogens Arsenic Polysorbate Radiation |
The incidence of VOD/SOS is variable, depending upon the degree of pre-existing liver disease, indication for SCT, conditioning regimen employed, age of the patient, previous SCT, and genetic predisposition [75]. It is more common in children and adolescents, with a prevalence of 20–60% (mean 22–30%), compared to a prevalence of 10% in adults [75].
Historically, the diagnosis of VOD/SOS in adults and children was based on the Baltimore or modified Seattle criteria, both of which limit the diagnosis to 20–21 days post-SCT and require a serum bilirubin >2 mg/dL in addition to tender hepatomegaly, weight gain, and/or ascites. In 2018, the European Society for Blood and Marrow Transplantation proposed pediatric-specific criteria for VOD/SOS that recognize crucial differences in clinical presentation between children and adults (Table 40.2). Specifically, the new criteria reflect the frequency of late-onset (15–20%) and anicteric (30%) disease in children, and the challenge of identifying abdominal tenderness, hepatomegaly, and ascites based solely on physical examination [76]. Increasing the sensitivity of the diagnostic criteria for pediatric VOD/SOS will ideally allow prompt diagnosis and early intervention before the onset of multi-organ system failure with its associated high mortality rate.
Table 40.2 European Society for Blood and Marrow Transplantation Diagnostic Criteria for Hepatic VOD/SOS in Children (Modified from [76])
• No limitation for time of onset of SOS/VOD |
The presence of two or more of the following: |
• Unexplained consumptive and transfusion-refractory thrombocytopenia (⩾1 weight-adjusted platelet transfusion/day to maintain institutional target platelet count) |
• Unexplained weight gain on three consecutive days despite the use of diuretics, or a weight gain >5% above baseline |
• Hepatomegaly above baseline value (best if confirmed by comparison with pre-SCT imaging) |
• Ascites above baseline value (best if confirmed by comparison with pre-SCT imaging) |
• Rising bilirubin from a baseline value on 3 consecutive days or bilirubin ⩾2 mg/dL within previous 72 hours |
In addition to confirming clinically suspected hepatomegaly and ascites, abdominal ultrasound, including pulsed Doppler analysis of the hepatic and portal vessels, can support the diagnosis of VOD/SOS and exclude other causes of hepatomegaly and jaundice. In particular, it is critical to distinguish VOD/SOS from pericardial disease and right-sided heart failure, as these conditions share similar mechanisms of hepatic injury and may present with identical symptoms. Ultrasound findings suggestive of VOD/SOS include reversal of portal venous flow, attenuation of hepatic venous flow, gallbladder wall edema, increased periportal echogenicity, and increased hepatic artery resistive indices; however, none of these findings add diagnostic or prognostic significance [77]. The gold standard for diagnosis of VOD/SOS in adults is measurement of the wedged hepatic venous pressure gradient. A gradient above 10 mmHg is highly specific for VOD/SOS [78]. Early histologic changes in VOD may be patchy and include dilatation of the sinusoids and extravasation of red blood cells into the perisinusoidal space, particularly in zone 3. Perivenular hepatocytes may atrophy and necrose, leading to fragmented hepatocyte cords. The later stages of VOD are characterized by collagenization of the sinusoids and a variable degree of obstruction of venular lumens. These late changes are correlated with severity and outcome [74]. Despite the diagnostic yield of liver biopsy and hepatic venous pressure gradient, the invasive nature of these procedures, and the potential for significant complications, limit their utility in children.
A variety of agents have been tested as prophylaxis for VOD/SOS, including continuous heparin infusion, tissue plasminogen activator, antithrombin III, fresh frozen plasma, N-acetyl-L-cysteine, prostaglandin E1, glutamine, ursodeoxycholic acid (UDCA), and defibrotide. Of these, prophylactic UDCA has the most encouraging data [79]. Two modestly sized randomized trials of prophylactic UDCA showed a decreased incidence of VOD/SOS in treated patients vs. those who received placebo (40% vs. 15% and 18.5% vs. 3%), while a third study, which failed to identify a difference in the incidence of VOD/SOS, found a significantly better one-year survival rate in patients who received UDCA (71% vs. 55%) [80]. A Cochrane systematic review of clinical trials using prophylactic UDCA concluded that while it may reduce the incidence of hepatic VOD/SOS, all-cause mortality and mortality due to VOD/SOS, the ideal regimen is not well‐defined [79]. In spite of the relatively low quality of the evidence for UDCA, its potential benefit and favorable side-effect profile support its common use in children following SCT.
Another promising agent for the prevention of VOD/SOS is defibrotide, a mixture of porcine oligodeoxyribonucleotides. Although the exact mechanism of action against VOD is unclear, defibrotide is known to have anti-inflammatory, anti-thrombotic, and pro-fibrinolytic effects without systemic anticoagulant effects. In a large, randomized controlled trial from Europe, which included 356 pediatric SCT patients, those who received prophylactic defibrotide had a statistically significant decreased incidence of VOD at 30 days post-transplant (11% vs. 20% in per-protocol analysis). Treated patients also had a decreased incidence of graft-versus-host disease (GVHD) and renal failure than control subjects, with no increased incidence of adverse events [81]. Although additional studies to replicate these findings and define the role of defibrotide as prophylaxis for VOD/SOS are necessary, several retrospective and single-arm prospective studies support the efficacy of defibrotide for treatment of established VOD, with overall response rates ranging from 30% to 76%. Based on these results, and approval by the United States Food and Drug Administration, current guidelines advocate treatment with defibrotide at 25 mg/kg/day divided into four daily doses. Duration of treatment is recommended to be 21 days or until resolution of organ failures and/or signs of VOD/SOS [82].
Aggressive supportive management is key to prevention and treatment of VOD/SOS, with a focus on maintaining intravascular volume while minimizing accumulation of extravascular fluid. Serial therapeutic paracentesis may be necessary to prevent respiratory compromise and provide comfort. Sodium restriction and diuretics are generally required to manage fluid accumulation; however, volume depletion must be avoided to prevent compromise of hepatic and renal blood flow [83].
More than 70% of patients with VOD will recover spontaneously with appropriate supportive management [74]. Poor prognosis is associated with higher total serum bilirubin at any given point in time, rapid weight gain early post-transplant, renal failure and multi-organ system failure [84].
Graft-Versus-Host Disease
Aside from primary disease relapse, GVHD is the most serious cause of morbidity and mortality following SCT. While GVHD has been described in patients after solid organ transplantation, in infants receiving intrauterine and exchange transfusions for hemolytic disease, and in immunocompromised patients receiving non-irradiated blood products, the most frequent setting is in recipients of SCT. Acute GVHD develops when the graft contains immunocompetent T-cells, the recipient is sufficiently immunocompromised such that he/she is unable to reject the transplanted cells, and the recipient expresses tissue antigens not present in the donor [85]. Given these criteria for the development of GVHD, it is no surprise that transplant from an unrelated donor and increased donor/recipient HLA mismatch are risk factors for GVHD.
Hepatic GVHD can present clinically in three different ways. The first is the typical presentation of acute GVHD, which classically occurs in the first 100 days post-SCT and often in the first two weeks. This presentation is marked by elevated total bilirubin, gamma-glutamyl transferase, and alkaline phosphatase, with milder elevations of AST and ALT. Extra-hepatic manifestations of acute GVHD, such as skin rash and diarrhea, are often present. The second presentation of hepatic GVHD, known as the hepatitic variant, is characterized by markedly elevated aminotransferases with relatively milder increases in alkaline phosphatase and gamma-glutamyl transferase. This presentation is almost always associated with reduction of immunosuppressive therapy or donor lymphocyte infusion, and is more likely to present without evidence of skin or gastrointestinal disease. In the third presentation, slow but progressive increases in alkaline phosphatase and gamma-glutamyl transferase occur, followed by the onset of jaundice. This indolent cholestatic disease is the classic clinical presentation of chronic liver GVHD and is usually associated with skin, mouth, and eye changes of chronic GVHD [86].
The diagnosis of hepatic GVHD is frequently made on clinical grounds, particularly when there is evidence of acute or chronic skin and/or gastrointestinal tract involvement; however, the differential diagnosis for liver disease in a SCT patient is broad, and includes not only VOD/SOS and GVHD but also drug-induced liver disease, opportunistic infection, and parenteral nutrition-associated liver disease. Therefore, in the absence of classic findings of GVHD, or with a poor response to GVHD therapy, liver biopsy may be necessary to confirm the diagnosis. A recent single-center study of liver biopsies in adult patients post-SCT found that histology influenced patient management in 65% of cases, as only 34% of pre-biopsy diagnoses were confirmed by histologic findings. In this report, no adverse events occurred, and 94% of biopsies led to a histologic diagnosis, of which, GVHD was the most common [87]. However, before proceeding with liver biopsy in children, the clinical information to be gained must be weighed against the risk, as complication rates as high as 27% have been reported in pediatric SCT recipients [88].
The characteristic histologic finding of GVHD is bile duct injury. Bile duct damage, atypia and pericholangitis are sensitive histologic markers for hepatic GVHD. Unlike other epithelial cell lines, apoptotic bile duct cells are infrequent, and lymphocytic infiltrates and bile duct proliferation are rare. Most cases show varying degrees of centrilobular cholestasis; less commonly, endotheliitis of the portal or central veins, and even centrilobular necrosis, are seen. In the hepatitic variant, dense portal lymphoplasmacytic infiltrates and prominent lobular inflammation are present, in a pattern resembling acute hepatitis. These findings almost always occur in addition to classic bile duct injury, which can help distinguish hepatitic GVHD from viral hepatitis. There are no specific criteria to distinguish acute from chronic GVHD in the liver. Prolonged disease, either acute or chronic, may result in ductopenia and periportal fibrosis [86, 89, 90].
Prevention of GVHD is the first approach to management. Strategies among centers vary widely, but may include a combination of the following medications and treatments: tacrolimus, cyclosporine, prednisone, mycophenolate moffetil, methotrexate, polyclonal antibody preparations, and use of donor T-cell depletion. Treatment of established acute GVHD requires augmented immunosuppression, usually with steroids and calcineurin inhibitors as first-line therapy. Options for steroid-resistant acute GVHD include polyclonal and monoclonal anti-lymphocyte antibody preparations, anti-TNF alpha antibodies, anti-IL2 receptor antibodies, sirolimus, extracorporeal photopheresis, pentostatin, and mesenchymal stem cells [91]. Chronic hepatic GVHD is more challenging to treat. While corticosteroids and calcineurin inhibitors remain first-line therapy, there is no standard second-line therapy, and response rates are only ~30% regardless of which agent is chosen [92]. Newer therapeutic approaches to chronic GVHD include B-cell depletion (rituximab), targeting the B-cell receptor signaling pathway (fostamatinib and ibrutinib), inhibition of cytokine receptor-mediated signaling (ruxolitinib), and a variety of strategies to augment T-regulatory cell reconstitution [92].
For patients with severe GVHD resulting in chronic cholestasis and/or cirrhosis, liver transplantation may be an option, with published one- and five-year survival rates of 72.4% and 62.9% in a mixed pediatric/adult population [93, 94].
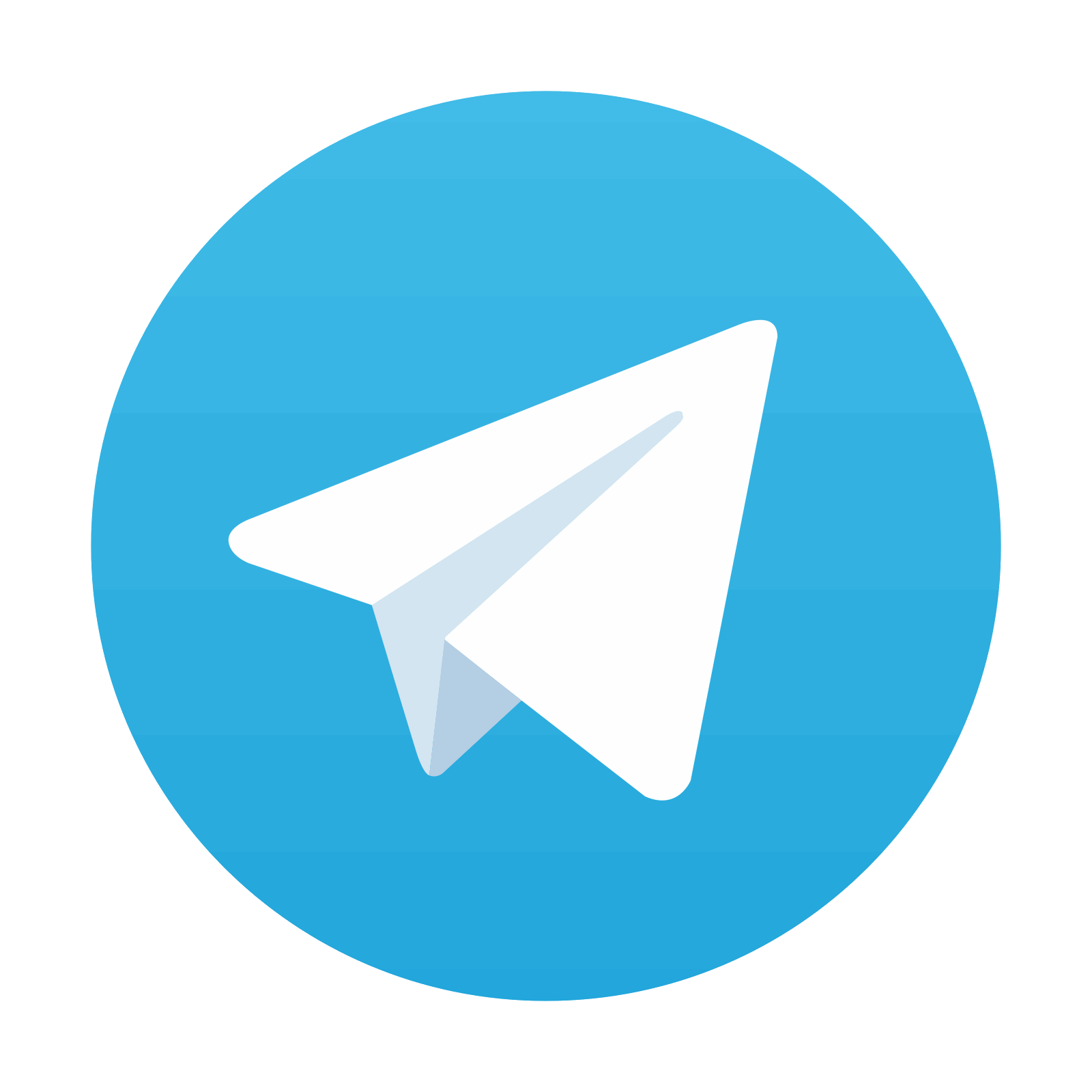
Stay updated, free articles. Join our Telegram channel

Full access? Get Clinical Tree
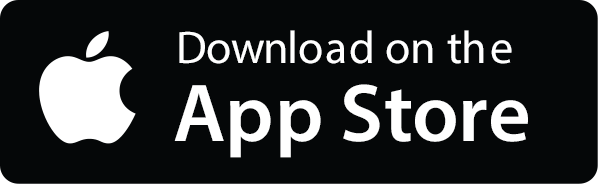
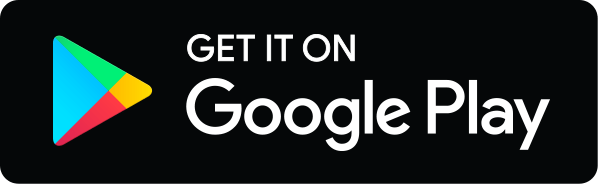
