Abstract
The urea cycle, first described in 1932 by Krebs and Henseleit, is the only metabolic pathway responsible for converting nitrogenous waste, produced by the breakdown of protein and other nitrogen-containing molecules into urea, which can be easily excreted from the body (Figure 38.1). Urea cycle disorders (UCDs) result from defects in this pathway and lead to an inability to rid excess nitrogen from the body, resulting in accumulation of nitrogen species, namely ammonia and glutamine, which are toxic in high concentrations. The incidence of these disorders is estimated to be at least one in 35,000 births [1], but partial defects, which may not manifest until later in life, may make this number higher.
Introduction
The urea cycle, first described in 1932 by Krebs and Henseleit, is the only metabolic pathway responsible for converting nitrogenous waste, produced by the breakdown of protein and other nitrogen-containing molecules into urea, which can be easily excreted from the body (Figure 38.1). Urea cycle disorders (UCDs) result from defects in this pathway and lead to an inability to rid excess nitrogen from the body, resulting in accumulation of nitrogen species, namely ammonia and glutamine, which are toxic in high concentrations. The incidence of these disorders is estimated to be at least one in 35,000 births [1], but partial defects, which may not manifest until later in life, may make this number higher.
Figure 38.1 Diagram of the hepatic components of the urea cycle. NO: nitric oxide; NOS: nitric oxide synthase; CA-Va: carbonic anhydrase Va; CPS-1: carbamoyl phosphate synthetase 1; NAG: N-acetylglutamate; NAGS: N-acetylglutamate synthase; ASS: argininosuccinate synthase 1; ASL: argininosuccinate lyase; ARG1: arginase 1; MOT: mitochondrial ornithine transporter (hyperornithinemia–hyperammonemia–homocitrullinemia syndrome).
This chapter discusses the clinical presentation, underlying molecular pathology, treatment options, and diagnostic testing for this group of diseases. The study of UCDs is of particular interest in gastroenterology because many acquired or genetic diseases of the liver have a secondary impact on the urea cycle and the processing of ammonia and nitrogen. Many of the treatment strategies employed in treating patients with rare urea cycle defects can be applied to the larger community of patients with hyperammonemia.
Nitrogen Metabolism
The bulk of the nitrogen waste comes from removal of the amino group on amino acids by gut bacteria and during the process of oxidative breakdown of nitrogen containing molecules in the body (see [2] for a review). This process occurs when there is an excess of amino acids beyond that needed for protein synthesis or during times of caloric insufficiency or stress, when the body utilizes its own protein as a source of energy. The brain also produces a significant amount of ammonia, which must be converted to glutamine for export through the blood-brain barrier. Glutamine is synthesized by the addition of nitrogen, first to α-ketoglutarate to make glutamate, and then again to glutamate to make glutamine.
Under normal conditions there is about 30 μmol/L soluble ammonia and 200 mg/L urea nitrogen in the blood. The conversion to urea in the liver is, therefore, an efficient and mostly one-way process, with a net expenditure of one ATP per urea molecule produced. Once produced, the urea is excreted primarily via the kidneys (75%), where it is used as a concentrating agent. The remaining 25% is excreted into the gut where bacteria can convert it to ammonia or use it to make their own amino acids or proteins. The remainder then re-enters the body through the portal system.
Molecular Aspects of the Urea Cycle
The urea cycle is the sole processing mechanism for nitrogenous waste. As shown in Figure 38.1, the urea cycle is composed of five primary enzymes, one enzyme producing an essential cofactor, and two transporters moving molecules across the mitochondrial membrane. The genes associated with these enzymes are shown in Table 38.1. Inborn errors of metabolism are associated with each step in the pathway and have been well described. Notably, in addition to producing urea, the urea cycle produces arginine which is needed for the synthesis of new proteins as well as a substrate for endothelial nitric oxide synthase.
Protein | Abbreviation | Gene Symbol | Chromosome | Location |
---|---|---|---|---|
Carbamoyl phosphate synthetase 1 | CPS | CPS1 | 2q34 | Mitochondria |
Ornithine transcarbamylase | OTC | OTC | Xp11.4 | Mitochondria |
Argininosuccinate synthase 1 | ASS | ASS1 | 9q34.11 | Cytoplasm |
Argininosuccinate lyase | ASL | ASL | 7q11.21 | Cytoplasm |
Arginase 1 | ARG | ARG1 | 6q23.2 | Cytoplasm |
N-Acetylglutamate synthase | NAGS | NAGS | 17q21.31 | Mitochondria |
Mitochondrial ornithine transporter (ornithine translocase) | MOT | SLC25A15 | 13q14.11 | Mitochondrial membrane |
Citrin (mitochondrial aspartate transporter) | CITRIN | SLC25A13 | 7q21.3 | Mitochondrial membrane |
Carbonic anhydrase Va | CAVA | CAVA | 16q24.2 | Mitochondrial |
The urea cycle as a nitrogen clearance system acts primarily in the human liver, and to a lesser extent the intestine. Carbamoyl phosphate synthetase and ornithine transcarbamylase are expressed exclusively in those tissues. The enzymes downstream of these enzymes, which process citrulline into arginine, are ubiquitous in their distribution, likely reflecting other roles in arginine synthesis.
Urea cycle disorders stem from deficiency or decreased function of an urea cycle enzyme or transporter. All the UCDs are inherited in an autosomal recessive manner, with the exception of ornithine transcarbamylase deficiency (OTCD), which is X-linked. Thus, in urea cycle patients, a copy of the gene inherited from both parents is affected (in the case of males with OTCD the only copy of the gene, inherited from the mother, is affected), and there is no normally functioning gene product. Mutations in the OTC gene may arise de novo during meiosis, so testing of parents is needed to give accurate recurrence risk counseling.
In severe disease, the underlying genetic alteration usually results in loss of all enzymatic activity or transport. Attenuated forms of the disease exist when the genetic variants lead to partial deficiency of the enzyme. In cases of attenuated disease, residual enzyme activity may be sufficient during normal states of health. Disease manifestations appear during circumstances of severe stress or catabolism, when the residual enzyme activity cannot compensate for the increased flux through the urea cycle.
Clinical Presentation of Urea Cycle Defects
The symptoms of urea cycle defects are primarily neurologic in origin. The acute disease presentation is often associated with cerebral edema, leading to neurocognitive dysfunction. That elevation in ammonia is responsibile for brain injury is well accepted, at least in the pediatric community, but the mechanism is not well defined and is likely complicated [3]. Both elevated glutamine, particularly those >1000 µmol/L, and ammonia concentrations are associated with poorer neurologic outcomes [4]. Depending on the age of the patient, the outward manifestations of hyperammonemia range from nausea and vomiting, disordered thinking, slurred speech, ataxia, increased agitation and/or somnolence to neurologic posturing as pressure is increased on the brainstem. The closed space of the skull magnifies the effect of the swelling and, if unchecked, progression of the edema will typically result in complete venous stasis or herniation of the brainstem. Without aggressive treatment, the prognosis for a patient with hyperammonemia and cerebral edema is poor. The exceptions to this clinical presentation are the disorders in the transporters (hyperornithinemia–hyperammonemia–homocitrullinemia (HHH) syndrome and citrin deficiency) and arginase deficiency, which are described separately, and likely reflect toxic mechanisms rather than acute cerebral edema. Although the inborn errors of urea cycle metabolism are being addressed in this chapter, it should be remembered that there are a number of conditions that affect the cycle which are not primarily genetic (Table 38.2), but may be associated with cerebral swelling and poor neurological outcome. Hyperammonemia, regardless of cause, is an acute medical emergency requiring immediate intervention.
Table 38.2 Conditions that Affect or Stress Urea Cycle Function
An increasing number of reports describe liver failure in the early stages of some UCDs [5–8]. There are no consistent pathognomonic findings on the histopathology of liver biopsies from patients with UCDs that are distinguishing, except for patients with defects in argininosuccinic acid lyase deficiency (ASL). Many patients with ASL develop significant hepatomegaly (often extending to the pelvis) with progressive fibrosis of the liver. On microscopic examination the hepatocytes may be pale and swollen [7]. Liver dysfunction also appears common in OTC deficiency, with a recent, large study describing liver dysfunction in 57% of a cohort of patients with OTCD [4].
Neonatal Onset
Severe defects in the urea cycle generally present in the neonatal period. The stress of the first few days of life, in addition to the catabolic state most newborns undergo while awaiting the mothers breast milk to come in, creates a significant ammonia burden. For those patients manifesting symptoms in the neonatal period, there is clinical evidence to suggest that they will become hyperammonemic even under ideal conditions [9].
It is often difficult to recognize UCD patients due to the similarity of symptoms to other more common problems such as neonatal sepsis (Table 38.3). Infants with a UCD usually appear normal at birth but deteriorate as the ammonia, which is no longer filtered by the placenta, accumulates. This typically occurs within the first 48–72 hours of life but may be delayed by one to two weeks. As cerebral edema develops, affected infants feed poorly, become agitated, and then progress to somnolence and lethargy. Open cranial sutures provide some protection for the neonate in slowing the increase in intracranial pressure, although it does not confer an indefinite defense. Most patients will develop some degree of dehydration from decreased intake. Although the decrease in oral intake will reduce the amount of dietary protein that can produce ammonia, the catabolism induced by poor intake and the stress of the neonatal period results in enough tissue protein breakdown to overwhelm any residual ammonia detoxification capacity. As the cerebral edema and vascular stasis progress, the patient may develop hyperventilation, resulting in respiratory alkalosis. As the pressure increases on the brainstem and CNS function fails, the patient’s respiratory rate will decrease and eventually stop. A portion of patients will develop seizures of some form, which may be sub-clinical and difficult to detect in the context of depressed CNS function without EEG monitoring. Unchecked, the patients will become comatose and eventually die from CNS failure, often compounded by brainstem herniation.
Table 38.3 Symptoms of Newborns with Urea Cycle Defects
Timescale | Symptoms |
---|---|
At birth | Normal appearance |
2–3 days of life (sometimes 1–2 weeks of life) |
|
Delayed Onset
Patients presenting with a delayed onset UCD typically have some function of their urea cycle [10, 11]. The onset of symptoms is a factor of their residual enzyme activity, diet, and environmental stressors (Table 38.4). These patients can manifest an acute hyperammonemic episode similar to that seen in the neonatal patients under circumstances of significant catabolic stress, such as during an infection or after trauma. These patients can also have episodes triggered by drugs that further decrease urea cycle function, such as valproic acid or chemotherapeutic agents [12–17]. Other reported triggers include postpartum stress and increased protein breakdown from the involution of the uterus, heart–lung transplant, short bowel, kidney disease, parenteral nutrition, gastrointestinal bleeding, and bariatric surgery [10]. Hyperammonemic episodes can occur any time, from just outside the newborn period to late in life (sixth to seventh decade).
Table 38.4 Common Clinical Features of Late-Onset Urea Cycle Defects
Features | Associations |
---|---|
Dramatic and rapid increase in nitrogen load |
|
Diet preference | Tend to avoid protein in diets |
Behavioral or psychiatric illness |
|
Many UCD patients will also experience numerous mild hyperammonemic episodes, often unrecognized, that can result in cumulative neurologic damage. It is suspected that the chronic mild elevations of ammonia and glutamine affect neurologic development in these patients as well.
The diagnosis of a UCD is often delayed in late-presenting patients because the symptoms and signs can be quite subtle. Possibly due to nausea caused by mild hyperammonemia from dietary exposure, many of these patients subconsciously avoid protein in their diet, which can be an important historic clue. Recurrent headaches, vomiting, and episodic confusion can also be presenting signs.
Classic Urea Cycle Disorders due to Single Enzyme Deficiencies
Patients with deficiencies of N-acetylglutamate synthase, ornithine transcarbamylase, and carbamoyl phosphate synthetase 1 (proximal urea cycle defects that occur inside the mitochondrion), may be indistinguishable on a clinical basis. Distal defects, including argininosuccinic acid synthase deficiency, argininosuccinate lyase deficiency, and arginase deficiency have more distinctive biochemical markers and/or clinical features. The most frequent disorders as a percentage of all UCDs are ornithine transcarbamylase deficiency (55%), argininosuccinic acid synthase deficiency (27%), and carbamoyl phosphate synthase deficiency (14%) [11].
Ornithine Transcarbamylase Deficiency
Ornithine transcarbamylase deficiency (OTCD) is unique among the urea cycle disorders in that it is an X-linked disease. Consequently, most affected individuals are males. Approximately 15% of carrier females will manifest overt symptoms of partial deficiency, particularly during pregnancy or other stressful events. Another fraction may have subtle symptoms and/or neurocognitive effects but be undetected in the absence of a family history of more severe disease. The median age of presentation of females is ten years [11].
Argininosuccinate Synthase Deficiency (ASSD or Citrullinemia Type I)
Argininosuccinate synthase deficiency is the first of the distal enzymes that are located in the cytoplasm of the cell. It is one of the more variable of the UCDs, ranging from a severe neonatal presentation that is clinically indistinguishable from OTCD to extremely mild, essentially asymptomatic forms [18] identified incidentally or, more recently, by newborn screening using citrulline as a marker. The distinctive finding is massively increased blood citrulline concentration. Therapy is similar to the proximal defects, except that arginine is used rather than citrulline. Outcomes vary, and some individuals have had significant developmental disability without severe episodes of hyperammonemia.
Argininosuccinate Lyase Deficiency
Argininosuccinate lyase deficiency (ASLD) presents similarly to the other urea cycle enzyme defects, although it is distinctive in that it has more severe chronic symptoms than other disorders, most likely due to toxicity of argininosuccinic acid, which builds up as a result of a block in this step of the cycle [19]. Patients with ASL deficiency have increased rates of developmental delay, seizure disorders, and other neurologic symptoms when compared to other UCDs. In addition, patients with argininosuccinate lyase deficiency may develop severe liver disease with hepatic fibrosis and hypertension. These individuals also have an unusual clinical finding, trichorrhexis nodosa, nodule-like fractures of hairs that lead to hair breakage, the clinical result of which is that their hair does not appear to grow. In fact, it does grow, however it breaks easily so does not get long. This finding typically improves with arginine supplementation.
Arginase Deficiency
Arginase deficiency is not typically characterized by rapid-onset hyperammonemia. These patients often present with progressive spasticity with greater severity in the lower limbs. They also develop seizures and gradually lose intellectual capacity. Growth is usually slow, and without therapy these patients usually do not reach normal adult height. Other symptoms that may present early in life include episodes of irritability, anorexia, and vomiting. Severe episodes of hyperammonemia are seen infrequently with this disorder but can be fatal. Treatment is similar to other UCDs, but targets reduction in plasma arginine to near-normal concentrations.
Non-Classic Urea Cycle Defects due to Transporter Defects
Citrin Deficiency (Citrullinemia Type 2)
Citrin is a mitochondrial membrane aspartate-glutamate transporter. Citrin deficiency has an increased incidence in Japanese and other Asian populations but is present in other populations. Citrin deficiency leads to reduction in cytosolic aspartate, specifically aspartate that serves as a substrate for the enzyme argininosuccinic acid synthase. Argininosuccinic acid synthase is the first cytosolic step in the urea cycle conjugating aspartate and citrulline to make argininosuccinic acid (Figure 38.1). Thus, in citrin deficiency, there is reduced flux through the urea cycle at the level of argininosuccinic acid synthase.
Citrin deficiency can present at any age with widely different manifestations and initial findings [20]. The earliest presentation, not seen in every affected individual, is a non-specific neonatal intrahepatic cholestasis that usually resolves after infancy [21]. This presentation may be referred to as “neonatal intrahepatic cholestasis caused by citrin deficiency” (NICCD). Newborn screen may demonstrate increased total galactose (with normal galactose-1-phosphate uridyltransferase activity) [22], hypermethioninemia, and/or hyperphenylalaninemia, in addition to increased levels of citrulline. Plasma citrulline is often, but not always, increased, and elevated plasma threonine and increased ratio of plasma threonine to serine may point to the diagnosis. The hepatic dysfunction is typically self-limited with supportive therapy, but occasionally more severe hepatic failure occurs requiring hepatic transplantation. Affected adults, in a presentation referred to as citrullinemia type II (CTLN2), may present with neurologic symptoms including abnormal behaviors, delusions, and seizures [23, 24], often with increased plasma citrulline, and with or without episodic hyperammonemia. Other complications include hyperlipidemia, hepatoma, neonatal hepatitis, and pancreatitis [22, 24–26]. An intermediate phenotype, “failure to thrive and dyslipidemia caused by citrin deficiency” (FTTDCD), presenting with poor growth and hyperlipidemia in early to mid-childhood may also be seen, often progressing later in life to a CTLN2 phenotype. An aversion to carbohydrate-rich food, with a preference for high-protein foods (paradoxical relative to other urea cycle defects), is notable in both childhood and adult onset cases.
Hyperornithinemia-Hyperammonemia-Homocitrullinuria Syndrome
Hyperornithinemia-hyperammonemia-homocitrullinuria (HHH) syndrome, due to mutations in the mitochondrial ornithine transporter, SLC25A15 (also called ORNT1), can present at any age, with a minority presenting in the newborn or early infant period [27]. Ornithine accumulation in the cytoplasm results in high plasma ornithine concentrations, whereas reduced intramitochondrial ornithine leads to impaired ureagenesis and orotic aciduria. The clinical symptoms are related to the hyperammonemia from insufficient ureagenesis and resemble those of the other UCDs.
The newborn presentation is typically that of acute hyperammonemia after initiation of protein containing feedings. It is distinguished from other defects by the metabolite profile, with increased ornithine and the presence of homocitrulline formed from the carbamylation of lysine. Where the carbamylation of lysine occurs is not completely known; possibly through a reaction that, if not catalyzed by OTC is, at least, tightly linked with OTC activity [28], which may explain why homocitrulline does not typically accumulate in OTC deficiency (although it has been seen at least once, author experience DAW). Later in life the disorder can present with developmental abnormalities, spasticity or seizures, with or without episodic encephalopathy due to modest increases of plasma ammonia. Liver dysfunction, with increased transaminases, occasionally with coagulopathy, may also be a presenting finding.
Diagnosis
The first step in diagnosing UCDs is clinical suspicion. Blood ammonia is the first laboratory test indicated to evaluate a patient with a suspected UCD. Particular care should be taken in drawing blood for ammonia measurement because there is considerable variability depending on technique and handling. Ammonia should as much as possible be drawn from a free-flowing source (venous or arterial) and the sample should be placed on ice immediately before it is sent to the laboratory. Tourniquet time should be minimized during sample collection and, if possible, removed after the needle is inserted. The sample must be spun down as soon as possible after collection in a cooled centrifuge, then either analyzed or frozen at -80°C immediately. Deamidation of amino acids, particularly glutamine, in the sample will release ammonia in a linear fashion until the sample is processed and frozen. The rate of this pre-analytical increase in ammonia is increased by increased serum GGT and ALT activity, increased glutamine concentration, and increased temperature [29, 30]. The clinician should remember that treatment of critically high ammonia should not be delayed in efforts to reach a final diagnosis. Later stages of treatment can be tailored to the specific disorder once it is established.
Laboratory data useful in the diagnosis of UCDs includes plasma ammonia levels, pH, carbon dioxide, calculation of anion gap, plasma amino acids, plasma acylcarnitine profile, and urine organic acid analyses [31, 32]. Both pH and carbon dioxide can vary with the degree of cerebral edema and hyper- or hypoventilation. In neonates, it should be remembered that the basal ammonia level is higher than that of adults. While a normal ammonia in adults is typically <35 μmol/L, a neonatal ammonia up to ~100 μmol/L is considered within normal limits [33], with the upper limit varying between laboratories. An elevated plasma ammonia of 150 μmol/L or higher, associated with a normal anion gap and a normal blood glucose level, is a strong indication for the presence of a UCD. It should be noted that in cases of significant catabolism beyond the neonatal period, ketosis may drive an anion-gap metabolic acidosis, and thus a UCD should not be ruled out if an acidosis is present.
Some clinicians may hesitate to obtain ammonia measurement in appropriate circumstances because of concern that “lab artifacts” related to sample handling will make the result difficult to interpret. This fallacy should be avoided. Significant elevations of plasma ammonia (>150 μmol/L) are rarely the result of sample handling issues, and minimal increases in plasma ammonia, especially in a neonate, need not lead to an extensive or urgent diagnostic evaluation. The risk of permanent brain injury due to delayed diagnosis of a UCD greatly outweighs the modest inconvenience of an occasional artifact.
Quantitative amino acid analysis can be used to evaluate these patients and establish a tentative diagnosis. Elevations or depressions of the intermediate amino-containing molecules arginine, citrulline, and argininosuccinate offer clues to where the defect occurs in the cycle, although moderate abnormalities should not be over-interpreted, as the amino acid profile in sick newborns without UCDs may show lower levels of arginine and citrulline and higher levels of glycine than in well children [32]. The blood concentration of the nitrogen buffering amino acid glutamine will also be quite high in patients with UCDs and can serve as confirmation of the hyperammonemia. If a defect in a proximal step of the urea cycle is suspected (N-acetylglutamate synthase, carbamoyl phosphate synthetase 1, or ornithine transcarbamylase), the presence of the organic acid orotic acid in the urine can help to distinguish the diagnosis of OTC from the two earlier steps. Orotic acid is produced when there is an overabundance of carbamoyl phosphate, which is shunted into the pyrimidine biosynthetic system. Thus, elevated orotic acid is a strong indicator of OTC deficiency. Normal orotic acid concentrations in conjunction with a plasma amino acid profile suggestive of a proximal defect would suggest either N-acetylglutamate synthase or carbamoyl phosphate synthetase 1 deficiency.
As shown in Table 38.5, biochemical testing with measurement of plasma amino acids is critical in differentiating between the various forms of UCD. Although enzyme testing is an effective means of diagnosis, it has largely been supplanted by DNA sequence analysis.
Table 38.5 Diagnosis of Urea Cycle Disorders
Deficiency Diagnosis | Biochemical Markers | Enzyme Assaya | Newborn Screeningb |
---|---|---|---|
N-Acetylglutamate synthase | Orotic acid N-↓, Cit ↓ | Liver | Low citrulline |
Carbamoyl phosphate synthetase 1 | Orotic acid N-↓, Cit ↓ | Liver | Low citrulline |
Ornithine transcarbamylase | Orotic acid ↑, Cit ↓ | Liver | Low citrulline |
Argininosuccinate synthase 1 | Cit ↑, Arg N-↓ | Fibroblasts, liver | High citrulline |
Argininosuccinate lyase | Cit ↑, ASA ↑, Arg N-↓ | Fibroblasts, liver, red blood cells | High citrulline |
Arginase | Arg ↑ |
| High arginine |
Mitochondrial ornithine transporter | Orn ↑, Homocit ↑ | Fibroblasts | Unknownc |
Citrin | Cit ↑, Thr/Ser ratio ↑ | Fibroblasts |
|
Arg: arginine; ASA: argininosuccinic acid; Cit: citrulline; Homocit: homocitrulline; N: normal; Orn: ornithine; Ser: serine; Thr: threonine.
a Not all enzyme assays may be available clinically.
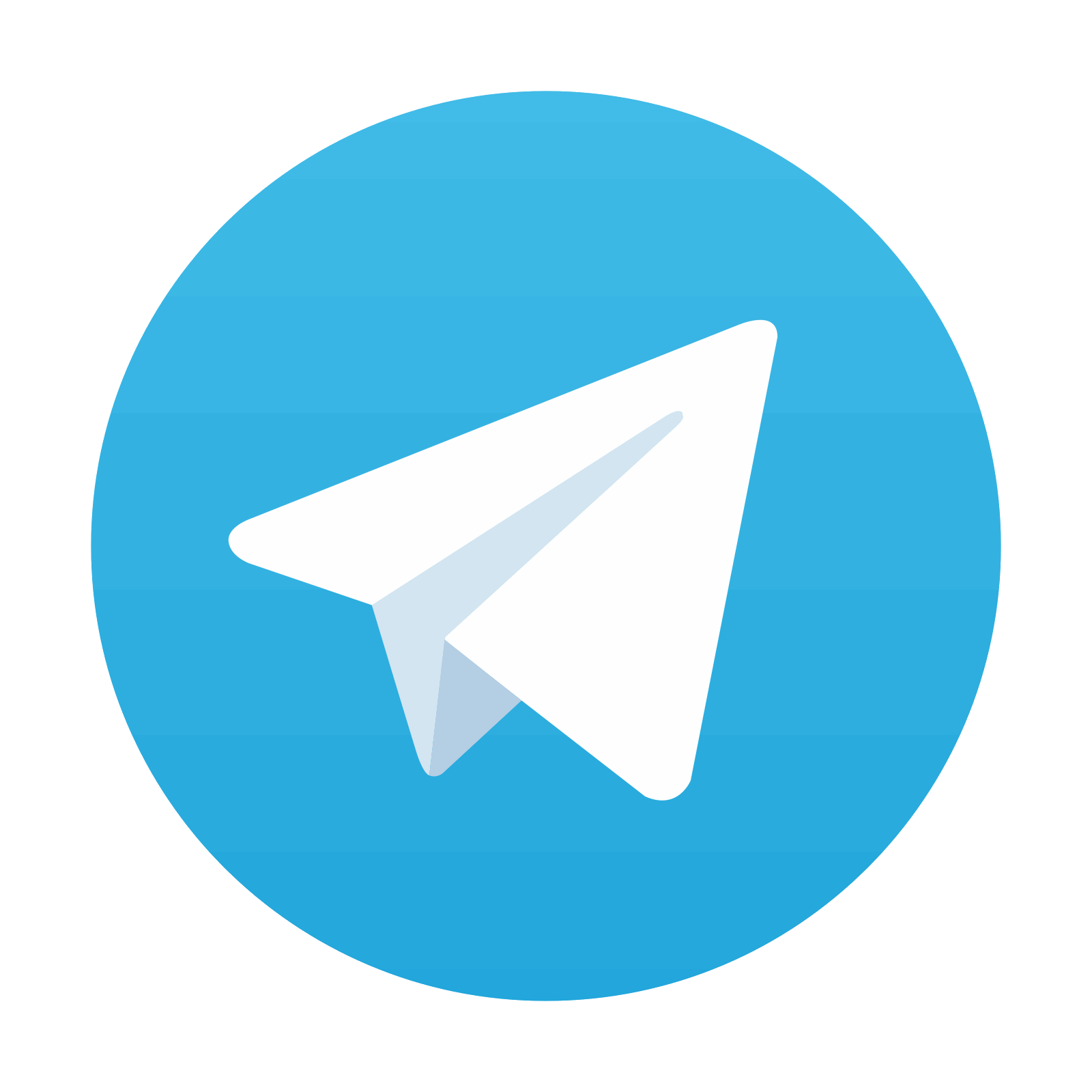
Stay updated, free articles. Join our Telegram channel

Full access? Get Clinical Tree
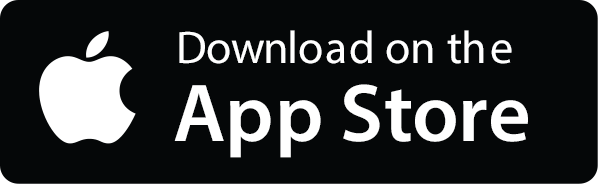
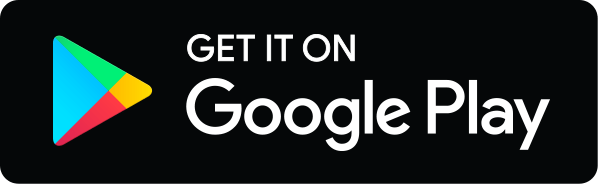
