Abstract
Lysosomes are membrane-bound cellular organelles that contain multiple hydrolases needed for the digestion of various macromolecules including mucopolysaccharides, glycosphingolipids, cholesterol esters and oligosaccharides. Moreover, the lysosome is emerging as a central hub for nutrient signaling and autophagy to maintain cellular homeostasis. A schematic of the lysosomal system enzyme trafficking and substrate accumulation is shown in Figure 32.1. The lysosomal storage diseases are a group of over 50 diseases that are characterized by defective lysosomal function, leading to an accumulation of specific substrates within the lysosomes and eventual impairment of cellular function that can progress to organ failure. The liver is involved as part of a multisystemic disease in most lysosomal disorders with a wide range of presentations from asymptomatic hepatomegaly with mild abnormalities of liver enzymes to life-threatening liver dysfunction.
Introduction
Lysosomes are membrane-bound cellular organelles that contain multiple hydrolases needed for the digestion of various macromolecules including mucopolysaccharides, glycosphingolipids, cholesterol esters and oligosaccharides. Moreover, the lysosome is emerging as a central hub for nutrient signaling and autophagy to maintain cellular homeostasis. A schematic of the lysosomal system enzyme trafficking and substrate accumulation is shown in Figure 32.1. The lysosomal storage diseases are a group of over 50 diseases that are characterized by defective lysosomal function, leading to an accumulation of specific substrates within the lysosomes and eventual impairment of cellular function that can progress to organ failure. The liver is involved as part of a multisystemic disease in most lysosomal disorders with a wide range of presentations from asymptomatic hepatomegaly with mild abnormalities of liver enzymes to life-threatening liver dysfunction.
Figure 32.1 The lysosomal system in normal (upper) and lysosomal disease (lower) cells. Lysosomal enzymes are synthesized on the rough endoplasmic reticulum, transported through the Golgi apparatus, and delivered to the lysosomes. In the rough endoplasmic reticulum, oligosaccharide chains are cotranslationally added to the nascent polypeptide. Sequential glycosidic modifications occur in the Golgi apparatus to trim terminal α-glucosidic and α-mannosidic residues, and specific glycosyltransferases add N-acetylglucosamine, β-galactose, and sialic acid. On selected high mannose chains, mannose-6-phosphates are exposed and used to target soluble lysosomal hydrolases to the lysosomes. Membrane-bound lysosomal proteins are targeted to lysosomes by specific peptides within the protein sequence. The glycosidic, proteolytic, and macromolecular processing of the lysosomal proteins occurs vectorially from cis- to trans-Golgi. The fully mature proteins and cofactors are delivered to the lysosomes after transit through the trans-Golgi network and endosomal system. Some lysosomal enzymes are lost from the cells by exocytosis into the surrounding intercellular milieu. Such enzymes, either exogenously supplied via enzyme replacement therapy or available by secretion, can be internalized by receptor-mediated endocytosis using the mannose-6-phosphate receptor or other receptor (e.g. macrophage mannose receptor) system. The intralysosomal deficiency of specific hydrolases or cofactors leads to an inability to cleave macromolecules (glycosaminoglycans, glycosphingolipids, oligosaccharides, etc.) and their resultant accumulation within distended lysosomes. The degree and rate of accumulation of such specific substrates will depend on the endogenous synthesis and/or exogenous delivery of substrates to particular cells and/or tissues. In the lysosomal storage diseases, the accumulated substrates elicit cellular reactions (pro-inflammatory, transcriptional, etc.) by mechanisms that remain undetermined.
Lysosomal storage diseases are classified by the nature of the stored material that results from the defects in cognate lysosomal enzymes, their cofactors, and/or enzyme or substrate transport (Table 32.1). The lysosomal storage diseases are heterogeneous, progressive, multisystem diseases that have a spectrum of ages of onset, severity, rate of progression, and organ involvement, thus making affected patients subject to prolonged diagnostic odyssey. Lysosomal storage diseases have significant morbidity and mortality in the absence of effective treatment. Most of these diseases are autosomal recessive and, although individually rare, the combined birth prevalence is approximately one in 7,000 live births [2]. The diseases are traditionally diagnosed biochemically, but molecular diagnosis for pathogenic mutations is playing increasingly prominent role through next generating sequencing and this information is very helpful in screening family, genetic counseling and assessing prognosis.
CoA: coenzyme A; +, ++, +++, or –: refer to progressively severe involvement from absent (–) to (→) very severe (+++).
The liver is nearly always involved in lysosomal storage diseases; this can be seen at the light or electron microscopic level. The degree of clinical involvement depends upon the disorder. In many cases, mild abnormalities in liver enzymes and/or hepatomegaly are the only manifestations. However, significant hepatic injury including advanced fibrosis and cholestasis may be present, resulting in considerable morbidity. For each of the diseases, the nature of the organ involvement will depend on the tissue-specific lysosomal enzyme content, substrate composition and turnover, and the rate of cell turnover/replacement. The resultant pathophysiological cascades being delineated involve immune dysregulation and pro-inflammatory cytokines triggered by primary accumulating substrate and/or associated metabolites.
Traditionally, these conditions were treated utilizing symptom-based management. Over the past several decades, safe and effective treatments including targeted recombinant enzyme replacement therapies for many of these conditions have been developed, with variable degrees of success. Rapid progress is being made in the realm of gene therapy and small molecule chemical chaperones and/or substrate reduction therapies. The potential for effective treatment of these conditions prior to the onset of irreversible organ damage is stimulating the addition of lysosomal storage diseases to newborn screening panels. The benefits and limitations of newborn screening for the lysosomal storage diseases have been debated, and further studies aimed at determining the utility of such testing is being assessed in several US states. The objective of this chapter is to provide clinicians with a broad overview of the lysosomal storage diseases that significantly affect the liver.
Sphingolipidoses
Sphingolipids are critical structural components of cell membranes that also function in protein sorting and in cell signaling and recognition. Mutations in the genes encoding the primary enzyme or their essential cofactors – that is, sphingolipid activator proteins (saposins) – lead to defective hydrolysis of specific sphingolipids and their abnormal accumulation within the lysosomes. Among the sphingolipidoses, Gaucher disease, acid sphingomyelinase deficiency (ASMD, previously, Niemann–Pick types A and B), Farber disease, and GM1-gangliosidosis are discussed here because of their specific effects on the liver.
Gaucher Disease
Gaucher disease, a prototype lysosomal storage disease, is due to biallelic mutations in GBA that encodes lysosomal glucocerebrosidase enzyme. The result is defective hydrolysis and accumulation of glucosylceramide and its de-acylated bioactive analogue, glucosylsphingosine that is generated via an alternative metabolic pathway involving acid ceramidase [3]. Of the several hundred known GBA mutations, only two variants account for the vast majority of disease mutations around the world. These two mutations are p.Asn409Ser, a founder mutation that arose in Eastern Europe in ~tenth century and p.Leu483Pro mutation originating from gene conversion events with closely linked and homologous pseudogene that harbors this mutation in its sequence. Presence of at least one copy of p.Asn409Ser mutation leads invariably to type 1 Gaucher disease with visceral, hematologic and skeletal disease but no early onset neurodegenerative disease, unlike type 2 and type 3. Homozygosity for p.Leu483Pro mutation leads to variable degree of neuronopathic disease in addition to severe visceral and hematologic disease. Overall population frequency of Gaucher disease is approximately one in 57,000 individuals but in the Ashkenazi Jewish population one in 855 individuals are affected due to the high prevalence of the founder mutation.
In the visceral tissues, there is accumulation of lipid-laden cells of monocyte/macrophage lineage, the eponymous “Gaucher cells.” These represent enlarged tissue macrophages, 20–100 μm in diameter, that are engorged with glucosylceramide-laden lysosomes as tubular inclusions and appear as wrinkled tissue paper (Figure 32.2). Gaucher cells are abundant in the liver, the spleen, the lungs, the bone marrow, and the lymph nodes. Pathological infiltration by glucosylceramide-laden macrophages underlies the clinical manifestations of hepatosplenomegaly, anemia, thrombocytopenia, pulmonary disease, lymphadenopathy, and destructive bone disease.
Figure 32.2 Bone marrow and liver findings in Gaucher disease. (A) The bone marrow macrophage is typical of the storage cells, that is, Gaucher cells. The typical “crinkled paper” cytoplasmic inclusions are evident. (B) Liver slice showing nodules (white/yellow) made up almost entirely of Gaucher cells. (C) By light microscopy, only Kupffer cells contain visible storage material, glucosylceramide, whereas the hepatocytes do not. Periodic acid–Schiff staining is negative in Kupffer cells. (D) Electron micrograph showing characteristic tubular storage material of Gaucher disease cellular inclusions (magnification 20,000×)
Three broad clinical sub-types of Gaucher disease have been described, based upon the absence or presence and severity of neuronopathic disease.
Gaucher disease type 1. This is the non-neuronopathic variant and accounts for approximately 90% of cases in Europe and the USA. The associated phenotypes are restricted to visceral organs. The onset of disease is highly variable and occurs from childhood to adulthood. Survival is frequently reduced, but many patients live into adulthood. A significant number of patients are discovered serendipitously in the sixth to eighth decades of life. This variant is prevalent among those of Ashkenazi Jewish heritage.
Gaucher disease types 2 and 3. These neuronopathic variants are distinguished by the age of onset of neurologic signs and symptoms and their severity. Although relatively rare in the European and North American populations, these neuronopathic variants are most frequent in Asia, Africa, and South and Central America [3]. In fact, globally, these severe phenotypes may outnumber type 1 Gaucher disease. Gaucher disease type 2 is characterized by very early onset in utero to six months of life, with fulminant progression of visceral and neurological disease. Death occurs at a mean age of nine months. Gaucher disease type 3 is characterized by slower progression of neurological disease but these patients generally have massive visceral disease. Indeed, horizontal supranuclear gaze palsy may present early without progression and with concomitant variable degrees of visceral disease. Survival is usually less than 40 years [3]. The demarcation between types 2 and 3 is blurred and these variants represent a continuum of CNS disease.
Hepatomegaly is nearly universal in patients with Gaucher disease, and there is great variation in the degree of enlargement. Massive enlargement of the liver, up to five-fold normal volume, can occur, with most severe hepatomegaly seen in the youngest and splenectomized patients [3]. Typically, the liver is enlarged 1.5- to 2.5-fold compared to normal. Clinically, elevations of serum aminotransferase and alkaline phosphatase levels are frequent. Liver dysfunction, including hepatic failure occurs, but is infrequent [3]. The most significant clinical and histological liver disease is observed in patients who have had splenectomy. Portal hypertension, with associated complications of esophageal varices, hepatic decompensation and hepatopulmonary syndrome, has been reported [4] in the most severely affected patients necessitating consideration for liver transplantation. Risk of hepatocellular carcinoma is increased and can occur in non-cirrhotic liver. There is high incidence of symptomatic gallstone disease attributed to altered bile composition related to sphingolipids.
Grossly, the liver may appear yellow-brown in the presence of severe Gaucher cell replacement, greyish red with white streaks in Gaucher cell infiltration, or dark-red to purple in areas of extramedullary hematopoiesis [3]. In more severely affected patients, the liver may have micronodules or larger nodules (Figure 32.2).
Examination of liver biopsies from patients with Gaucher disease types 1, 2, and 3 reveals a broad spectrum of histopathological features [4]. Pattern of fibrosis is atypical with dense areas of confluent fibrosis and pericellular fibrosis around Gaucher cells.
Gaucher cell accumulation and fibrosis are the most common pathological features of untreated disease. The degree of involvement varies from scattered aggregates of Gaucher cells in sinusoids with few other abnormalities to more severe manifestations. Pericellular fibrosis is commonly in a diffuse pattern, but severely atrophic and diminished numbers of hepatocytes is unusual. Central zonal (zone 3) distribution of Gaucher cells is common. The central veins are frequently compressed and obscured. Storage cell accumulation is occasionally noted in the portal zones, periportal regions, and mid-zones, but rarely to the degree observed in the central zones. Hepatocytes close to the storage cells may show degenerative changes and atrophy. Pericellular fibrosis is frequently observed and is characterized by thick fibrous septa surrounding the central vein with replacement of the normal hepatic parenchyma in some cases. Fibrotic linkage of adjacent central zones by fibrous bands, with involvement of the portal triads, consistent with micronodular cirrhosis, as well as regenerative activity, has also been reported. Additionally, extramedullary hematopoiesis has been noted in a few patients. Rare cases of neonatal cholestasis have been reported complicating type 2 Gaucher disease.
Severe liver involvement in Gaucher disease type 1 manifests as nodular hepatomegaly with extensive central, acellular fibrosis, surrounded by parenchymal nodules, frequently incorporating portal tracts. The fibrous tissue may involve the portal tracts with focal ductal proliferation and surround small parenchymal nodules, some of which exhibit canalicular cholestasis. Gaucher cells are most often found at the fibrotic margins.
One study documented neonatal cholestasis in a child with Gaucher disease type 2 [5]. The child presented at seven days of age with cholestasis and hepatosplenomegaly. The clinical course was complicated by progressive portal hypertension, worsening hepatosplenomegaly, but improvement in cholestasis. Liver histology showed advanced fibrosis, without inflammatory cell infiltrates, and Gaucher cells around the periportal space and surrounding the central hepatic veins. The child died of uncontrollable upper gastrointestinal bleeding at 4.5 months [5].
The definitive test for diagnosis of Gaucher disease is the demonstration of low acid β-glucosidase activity (less than 10–15% of control) in peripheral blood leucocytes or cultured skin fibroblasts. The enzyme is not normally present in erythrocytes or plasma/serum. Molecular diagnosis through GBA gene sequencing can provide guidance for prognostication and the risk of CNS or non-CNS involvement, and overall disease severity [3]. It should be noted that negative screening for a few common mutations does not rule out the diagnosis, as there are several hundred known disease mutations. Genotype–phenotype correlations are based on associations and are not perfect. Counseling based on risk factors is rapidly evolving and should be conducted by healthcare professionals with expertise in this area. Treatment of Gaucher disease is multidisciplinary due to its multisystemic nature. Although macrophage mannose receptor targeted recombinant enzyme therapy has become the standard of care, comprehensive treatment of patients with Gaucher disease involves supportive management for the complications of Gaucher disease, including appropriate orthopedic procedures and management of hematologic and CNS signs and symptoms. Allogenic bone marrow transplantation reverses some systemic features of Gaucher disease; however, the risks have rendered this option obsolete with availability of current safe therapies.
Enzyme replacement therapy (ERT) for Gaucher disease is the standard of care for the control and reversal of visceral, hematologic and some skeletal manifestations. Intravenous enzyme therapy has not been proven to have direct beneficial effects on the CNS involvement but plays a supportive/palliative role for type 2, and it is lifesaving for devastating visceral manifestations of type 3 disease. Enzyme therapy is administered by lifelong biweekly intravenous infusions of recombinant human acid β-glucosidase that have been modified for preferential uptake by macrophages via the mannose receptor. There is now almost 25 years of experience of enzyme therapy since the introduction of recombinant imiglucerase (Cerezyme) and it has proven remarkably effective in reversing hepatosplenomegaly, cytopenia, growth failure and numerous aspects of skeletal disease. More recently, other forms of ERT have been approved which have similar effects: velaglucerase (VPRIV, Shire Human Genetic Therapies, Cambridge, MA, USA), and taliglucerase alfa (Elelyso). The products differ in their manufacturing processes, glycosylation patterns, and at amino acid residue 495.
In a study of 1,028 patients with Gaucher disease type 1 treated with imiglucerase, a 20–30% decrease in hepatomegaly was reported within one to two years of treatment. Additional reductions to near normal were achieved by five years.
In patients with massive hepatomegaly, the rate of decrease was slower, but overall gains were substantial. Concomitantly, there was reversal of anemia and thrombocytopenia [6]. The safety profile of imiglucerase has been extensively reviewed and shows that the drug is generally safe and well tolerated. Although such long-term data are not available for velaglucerase or taliglucerase alfa, phase II and III studies demonstrate similar safety and efficacy to imiglucerase.
Orthotopic liver transplantation has been successfully performed in patients with end-stage liver disease with long-term ERT. However, with appropriate ERT regimen, even such devastating liver disease can be reversed without the need for liver transplantation.
An alternative approach to treatment of Gaucher disease is substrate reduction therapy (SRT) using inhibitor of the enzyme, glucosylceramide synthase, to decrease synthesis of glucosylceramide. Two SRTs are approved for adults with type 1 Gaucher disease: Miglustat (N-butyldeoxynojirimycin) (Zavesca), approved for limited indication in adults with mild to moderate Gaucher disease type 1 for whom ERT is not an option due to lower efficacy and adverse events and more recently, eliglustat.
A first-line oral SRT, eliglustat was approved for adults with suitable CYP2D6 metabolizer status in 2014 after an extensive clinical trial program over 12 years involving some 400 patients. Remarkably, monotherapy with eliglustat is effective in reversing visceral and hematological manifestations of even the most severely affected patients [7, 8]. In general, eliglustat tartrate is well tolerated at therapeutic doses. Indeed, most adverse events were mild to moderate in intensity, and many of the side effects noted for miglustat, especially diarrhea, were not experienced [7, 8].
Neither enzyme replacement therapy nor substrate reduction therapy in their current versions has demonstrated effectiveness in preventing or improving neurologic dysfunction in patients with Gaucher disease types 2 and 3, as these therapeutics do not cross the blood brain barrier.
Acid Sphingomyelinase Deficiency: Niemann–Pick Types A and B
Acid sphingomyelinase deficiencies are heterogeneous, autosomal recessive lysosomal disorders characterized by an accumulation of undegraded sphingomyelin and other lipids in the lysosomes of multiple cell types, particularly those of macrophage/monocyte lineage, which are termed Niemann–Pick cells. The gene SMPD1 encodes sphingomyelin phosphodiesterase-1 (acid sphingomyelinase) and maps to chromosome 11p15.4-p15.1. More than 50 mutations have been found in SMPD1 of affected patients.
The three forms, NPA, NPB, and an intermediate form, NPAB, are phenotypes within a continuum of disease that vary by age of onset, absence or presence of neurological disease, severity of features, and survival. Visceral features include growth retardation; hepatomegaly, often without liver dysfunction; splenomegaly; gastrointestinal disturbances; hyperlipidemia; pulmonary disease; osteoporosis; lymphadenopathy; pancytopenia; and ocular abnormalities, particularly cherry red maculae. The incidence of acid sphingomyelinase deficiency has not been well studied and varies depending upon the specific population. However, the combined frequency of NPA and NPB was estimated at approximately one in 248,000 in Australia [9].
Niemann–Pick type A. This is the most severe phenotype and displays progressive neurovisceral disease within the first several months of life. Survival depends upon supportive care, but usually does not extend beyond age two to three years.
Prolonged neonatal jaundice, hepatosplenomegaly, usually without liver dysfunction, and failure to thrive are among the earliest features [10]. Affected individuals experience progressive hypotonia, muscle weakness, intellectual decline, and loss of milestones that transforms to spasticity and rigidity at the end stages of the disease. It is particularly prevalent in the Ashkenazi Jewish population in which approximately one in 80 is a carrier [10].
Niemann–Pick type B. This is a heterogeneous, non-neuronopathic form with the visceral features described above. It is frequently diagnosed in childhood to adolescence with survival into adulthood. Hepatosplenomegaly is most prevalent during childhood but tends to become less conspicuous with age [10]. Within a subpopulation of patients with this subtype of disease, pulmonary disease is a significant source of morbidity. The disease is pan-ethnic in nature, with highest incidence in individuals of Turkish, Arabic, and North African descent, and less frequently among those of Ashkenazi Jewish heritage.
Niemann-Pick type AB. The intermediate variant of acid sphingomyelinase deficiency is common and is seen in over 60% of affected individuals in central Europe. The intermediate variant is defined by a cluster of visceral features and a protracted neuronopathic course. A rapidly progressive, early fatal, visceral variant without major CNS involvement has also been described.
Hepatomegaly is a common finding in acid sphingomyelinase deficiency. In 29 patients with NPB, the mean liver volume was 1.91-fold increased. Mild, stable elevations of serum aminotransferase and bilirubin were common in patients in this study [11, 12]. Hepatic fibrosis, cirrhosis, portal hypertension, and liver failure have been reported in adults and children, typically resulting in a poor outcome [11, 12]. Orthotopic liver transplantation has been successfully performed in several individuals with NPB. Overall, the liver is a major target of the disease and advanced liver disease is a major cause of morbidity and mortality.
The liver in patients with NPB is often smooth, firm, and yellow to orange in coloration [12, 13]. Hepatocytes and Kupffer cells contain vacuolated storage material within the cytoplasm, imparting a foamy appearance. This vacuolated, foamy appearance represents a common artifact of fixation and processing, whereby most sphingomyelin is dissolved and lost by routine formalin fixation and paraffin embedding procedures. (Figure 32.3A). Their distribution may initially be spotty but may become generalized in later stages of the disease. Niemann–Pick cells are approximately 25–75 μm in size and stain negative with periodic acid–Schiff (PAS) stain, but positive for lipid with Sudan black B and Oil red O. When biopsies are preserved in a glutaraldehyde-based fixative and embedded in epoxy resin, sphingomyelin is preserved and retained within the tissue. In the resulting one-micron thick, high resolution light microscopy sections, sphingomyelin storage appears dark purple when stained with a modified toluidine blue [13, 14] (Figure 32.3B). Electron microscopy shows the characteristic “fingerprints” like whorls of sphingomyelin (Figure 32.3C). During the disease course, Niemann–Pick cells initially accumulate in the sinusoids but later extend to involvement of the portal areas [12, 13]. These cells can accumulate to such a degree within the sinusoids that intrahepatic obstruction can result. Extramedullary hematopoiesis is occasionally present in liver biopsies: this is not surprising given the widely known disease involvement within the bone marrow (author’s personal communication, Dr. Beth Thurberg). Increasing degrees of fibrosis can occur and progress to cirrhosis in advanced disease (Figure 32.3D) [12, 13].
Figure 32.3 Acid sphingomyelinase deficiency. (A) Photomicrograph of a liver section from a six-year-old girl with Niemann–Pick type B. Obvious “foamy” Kupffer cells are present (hyphenated arrow). Storage in hepatocytes is also clearly observed (solid arrow). The storage material is periodic acid–Schiff–diastase negative (magnification 400×. Courtesy of M. Collins.) (B) Photomicrograph of liver from an adult with Niemann-Pick type B. In this high resolution light microscopy tissue section, epoxy resin embedding preserves sphingomyelin well, allowing its identification by modified toluidine blue staining (purple) in both Kupffer cells (K) and hepatocytes (H) (1 µm section, magnification 600×) (Courtesy of B. Thurberg). (C) Electron microscopy image of liver from an adult with Niemann-Pick type B. The fingerprint-like whorls are characteristic of the ultrastructure formed by excessive sphingomyelin storage in hepatocytes shown here (magnification 1500×. Courtesy of B. Thurberg). (D) Photomicrograph of a liver biopsy from an adult with Niemann-Pick type B. This sample demonstrates development of cirrhosis in advanced disease. Note the dense blue bands of collagen surrounding the regenerative, cirrhotic nodules throughout the specimen (trichrome stain, magnification 600×.
The diagnosis of acid sphingomyelinase deficiency is established by the deficiency of acid sphingomyelinase in nucleated cells. The type and severity of disease does not correlate well to the amount of residual enzyme activity. The enzyme is not present in significant amounts in erythrocytes or plasma/serum. The diseases may also be diagnosed by the identification of pathogenic mutations in both copies of SMPD1.
Current management of acid sphingomyelinase deficiency is symptomatic. Bone marrow transplantation (BMT) has been performed in multiple individuals with NPA and NPB, but despite evidence of engraftment, the patients exhibited progression of their CNS disease [17]. Examination of a liver biopsy from a 16-year-old, taken 6.5 years after BMT for persistent hepatomegaly, revealed only partial correction of the disease within the Kupffer cells; hepatocytes remained engorged with sphingomyelin (personal communication, Dr. Gregory Grabowski). Enzyme replacement therapy has been developed and phase Ia and Ib studies have been conducted evaluating the safety of the product in adults with NPB [14, 15, 16]. A phase 2/3 trial examining the long-term efficacy of recombinant acid sphingomyelinase began in 2016 and is ongoing.
Farber Disease
Farber disease is a rare, progressive, autosomal recessively inherited lysosomal storage disorder resulting from mutations in ASAH, the gene encoding the lysosomal enzyme acid ceramidase. The diagnosis is established by detection of markedly deficient acid ceramidase activity in plasma, leukocytes, and cultured skin fibroblasts, or by molecular evaluation. The level of in vitro residual enzyme activity does not correlate with disease severity. ASAH maps to chromosome 8p21.3-p22. Many types of mutation result in Farber disease; however, genotype–phenotype correlations are not perfect because the rarity of the disease precludes robust analysis.
Several subtypes of Farber disease are recognized, varying by phenotypic features, severity and survival. Variable degrees of pulmonary, cardiac, hepatic, splenic, reticuloendothelial, and nervous system involvement may also be present [18].
Classic Farber disease. This is the most common subtype and is characterized by the classic triad of laryngeal, subcutaneous, and joint involvement that develops within the first months of life. Affected individuals experience failure to thrive. Swallowing dysfunction and pulmonary compromise develop as a result of granulomas in the epiglottis and larynx, requiring gastrostomy tube feedings and tracheostomies in the most severe cases [18]. These granulomatous lesions also occur in connective tissue, the heart, spleen, reticuloendothelial system, liver, bone, and the nervous system, contributing to the disease process [18].
Hepatomegaly is observed in approximately 40% of affected individuals, but liver dysfunction is uncommon 18]. Death occurs in the first few years of life, often from pulmonary disease [18]. An attenuated variant displays similar, albeit milder, features of the classical disease. The age of onset is later in childhood and survival is frequently into adulthood [18].
Neonatal-visceral subtype. This severe form has neonatal or early infantile onset and is characterized by liver dysfunction, with death occurring in early infancy, often before the development of classical features of Farber disease [18]. In the most severe cases, it may present as hydrops fetalis.
Progressive neurologic subtype. This is manifested by progressive, generalized, neurologic deterioration and seizures within the first few years of life, followed by death in early childhood. Although subcutaneous nodules and joint disease have been described in these patients, those features do not predominate and the visceral organs are not typically affected.
Pathologic features include an accumulation of storage material within macrophages or histiocytes, termed foam cells. Granulomas can be present and contain a central core of foam cells surrounded by macrophages, lymphocytes, and multinucleated cells, as well as fibrosis in older nodules [18]. Ultrastructurally, the foam cells contain distended lysosomes filled with curvilinear tubular structures, Farber bodies [18].
The liver in Farber disease is firm, nodular in texture, and yellow in coloration [18, 19]. The variable liver histopathology spans a spectrum from microscopically normal to minimal fatty infiltration. In more severe disease, granulomatous lesions are present [18, 19]. The liver in neonatal-visceral Farber disease can exhibit sinusoidal fibrosis, with weakly eosinophilic, vacuolated storage cells filling the sinusoids [18, 19]. Mild intracellular and canalicular cholestasis can be present. Mild biliary ductal proliferation has been noted in the expanded portal zones [18, 19].
Symptomatic management has been the therapy for the disease. Several individuals with classic Farber disease, including neurologic dysfunction, have received BMT. A significant improvement was observed in their visceral features, but neurological deterioration continued until death [20]. Recently, BMT in two patients with the attenuated phenotypes of Farber disease and normal neurological function led to significant reductions in the disease features and improvement in their quality of life [21]. Recombinant enzyme replacement therapy for Farber disease is in pre-clinical stage of development.
GM1-Gangliosidosis
GM1-gangliosidosis is a heterogeneous, progressive neurovisceral disorder due to inherited deficiency of β-galactosidase that results in GM1-ganglioside, asialo-GM1-ganglioside, oligosaccharides, and keratin sulfate accumulation in a variety of tissues. It is an autosomal recessive disease caused by mutations in GLB1, encoding the β-galactosidase. GLB1 maps to chromosome 3p21.33. It has been well characterized and dozens of disease-causing mutations have been identified, including missense, nonsense, duplication, insertion, and splice defect mutations.
Three broad phenotypes are recognized (types 1, 2, and 3), based upon age of onset, severity of neurological involvement, presence or absence of visceral disease, and survival (Table 32.2). The prevalence is approximately one in 384,000 individuals. The disease is allelic to another condition, Morquio type B disease (a mucopolysaccharidosis lacking neurologic or hepatic features), but only GM1-gangliosidosis is discussed here. The condition has a similar phenotype to galactosialido- sis, as β-galactosidase exists as a multi-enzyme complex with N-acetylgalactosamine-6-sulfate sulfatase and protective protein/cathepsin A and defects of the last (as seen in galactosialidosis) result in secondary deficiencies of β-galactosidase and N-acetylgalactosamine-6-sulfate sulfatase.
Table 32.2 GM1 Gangliosidosis Variants
Early infantile-onset (type 1) GM1-gangliosidosis. This presents within the first six months of life with significant neurodegenerative changes, feeding difficulties and failure to thrive, coarse features, cherry-red maculae, and dysostosis multiplex. Hepatomegaly is usually present at or shortly after birth, and is often associated with other features including generalized peripheral edema, ascites, or abnormalities in liver studies; the last may occur independently of hepatomegaly. Hydrops fetalis may be observed. Overt liver dysfunction is not usually present.
Late infantile/juvenile-onset (type 2) GM1-gangliosidosis. This presents from around six months to three years of age and is associated with neurodegenerative disease. The patients may or may not exhibit coarse facies, cherry-red maculae, and hepatosplenomegaly [22]. Death frequently occurs by five years of age.
Adult-onset/chronic (type 3) GM1-gangliosidosis. This is a slowly progressive CNS disease. Dementia, ataxia, speech disturbances, dystonia, and parkinsonism are common neurological features in this subtype. Hepatosplenomegaly may or may not be observed.
In hepatocytes, neurons, glomerular cells, and myocytes, storage material accumulates, stains pink with hematoxylin and eosin and is PAS positive [22]. Cells of monocyte/ macrophage lineage, including Kupffer cells, also accumulate storage materials within affected organs [22]. Ultrastructurally, affected cells exhibit numerous, pleomorphic storage material-laden lysosomes that appear empty or contain fibrillar and granular material. The liver can appear normal under microscopic examination, particularly in individuals with later onset disease [22].
GM1-gangliosidosis is diagnosed by demonstrating severely decreased acid β-galactosidase activity in lymphocytes, fibroblasts, or plasma. The disease can be confirmed molecularly by the detection of GLB1 mutations. Well-defined genotype– phenotype correlations have not been recognized; therefore, mutation analysis cannot be used to predict the clinical phenotype. Although the disease phenotype does not correlate well with the level of residual enzyme activity, individuals with greater degrees of residual enzyme activity have generally been noted to have later onset and milder disease [22]. The detection of complex carbohydrates in the urine, including keratin sulfate, adds further support to the diagnosis, but is not diagnostic.
The treatment for this disease is symptomatic management. Bone marrow transplantation is generally unsuccessful in stabilizing/reversing the neurological deterioration in individuals with GM1-gangliosidosis.
Niemann–Pick Disease Type C
Although originally classified with NPA and NPB variants as an acid sphingomyelinase deficiency, the two forms of NPC (NPC1 and NPC2) result from lipid-trafficking defects unrelated to SMPD1 mutations. The NPC1 and NPC2 phenotypes are essentially identical [23, 24, 25]. However, the NPC nomenclature is engrained in the literature and will be used here. The prevalence of NPC has been estimated to be approximately one in 120,000–150,000 in Western Europe; however, it is pan-ethnic in nature [25, 26]. Based on age of onset, CNS features, and survival, three clinical forms have been delineated: neonatal/infantile, childhood, and adolescent/adult [25]. NPC is an important diagnosis to keep in mind in pediatric hepatology as hepatic presentation is prominent in children.
Niemann-Pick disease, type C1 and NPC2 are autosomal recessive neurovisceral diseases with highly variable ages of onset, pathological features, and survival [25, 26]. The primary defect is failure to traffic free cholesterol out of the lysosome after hydrolysis of LDL-derived cholesterol ester. This primary defect also involves storage of lipids other than unesterified cholesterol including phospholipids, sphingomyelin, and glycolipids in lysosomes of affected cells, as well as secondary sphingomyelinase deficiency that results in progressive visceral disease manifesting as hepatic and splenic involvement and neurodegenerative disease [25, 30]. Secondary effect of NPC metabolic defect on the lysosome can cause much diagnostic confusion and it has been misdiagnosed as Gaucher disease due to low leucocyte acid lysosomal glucocerebrosidase activity. Although the onset and degree of progression is variable among the phenotypes, visceral disease (when present) may occur before or after the development of neurological symptoms [25]. However, the visceral findings may be absent or minimal, particularly in adult-onset disease, particularly at the time of diagnosis [25].
The neonatal/infantile subtype accounts for around 20–30% of NPC. The disease is a rapidly progressive neurovisceral disease, presenting within the first few months of life as developmental delay. Severe hepatic dysfunction can manifest perinatally, as ascites or hydrops fetalis. In early infancy, liver disease/dysfunction (i.e., infantile cholestatic disease or idiopathic neonatal hepatitis) often develops, frequently in association with progressive hepatosplenomegaly [25, 29]. In approximately 10% of such patients, hepatic failure develops and death occurs [27]. Those surviving show resolution by the second to fourth months of life. Hepatosplenomegaly may remain, but splenomegaly is usually predominant [27]. However, survivors may “grow into their organs” such that hepatosplenomegaly may not be detected later in childhood [25].
Of those who recover from the liver dysfunction, some immediately demonstrate neurological symptoms whereas others have resolution of symptoms only to present at a later age with progressive neurodegenerative disease, including hypotonia, developmental delay, loss of acquired skills, spasticity, dystonia, dysphagia, seizures, and pyramidal signs [23, 24, 25].
Vertical supranuclear gaze palsy is classically observed in individuals with NPC but is not as common in those with neonatal/infantile presentations as in other subtypes [25]. Generalized failure to thrive and growth retardation occur.
In some infants, particularly those with mutations of NPC2, a significant infiltrative pulmonary disease may also occur as a primary feature or accompanying hepatic involvement, which may act as a significant source of morbidity and mortality [25, 28]. Death usually occurs by five years of age.
The childhood NPC1 variant accounts for about 50–70% of affected individuals. Children with this variant may initially have normal development, but by early to late childhood, they exhibit neurocognitive deterioration, initially presenting as clumsiness, behavioral problems, and poor school performance [25]. Psychiatric disturbances, including psychosis, may develop around the time of puberty. Other signs of neurodegenerative disease gradually appear, including ataxia, vertical supranuclear gaze palsy, dystonia, seizures, cataplexy, dysarthria, and dysphagia [25]. Dysphagia is progressive and eventually makes oral feeding impossible. The individuals eventually become dependent on caregivers for all of their needs. Survival into teenage years and adulthood can occur, and death is usually the result of pulmonary disease [25]. Significant liver dysfunction is unusual.
The adolescent/adult-onset NPC2 variant accounts for around 5–10% of cases and presents with psychiatric and/or neurological symptoms. Indeed, these findings frequently overshadow the visceral findings of the disease [25]. The psychiatric symptoms often mimic depression or schizophrenia and can be quite debilitating. Dementia often occurs, but there is a slower rate of progression than in other subtypes of the disease. Vertical supranuclear gaze palsy may be subtle and difficult to recognize. Hepatomegaly and splenomegaly are observed in approximately 13% and 50% of patients, respectively [25].
Histopathologically, NPC exhibits accumulation of free cholesterol and sphingolipids within cells of monocyte/macrophage origin, in the visceral organs (i.e., hepatocytes), and neurons and glial cells of the nervous system [25, 28]. The storage cells exhibit a foamy appearance and stain positively with PAS and filipin. Because of their microscopic appearance, storage cells of monocyte/macrophage origin are often referred to as “foam cells.” Ultrastructurally, these lipid-laden cells contain numerous, pleomorphic membrane-bound lipid inclusions with structures varying from crystalline to electron-dense laminated inclusions [28].
At autopsy, the liver is enlarged and firm, with a surface that is pale yellow to green in appearance [27, 28]. The neonatal liver disease may resemble idiopathic neonatal hepatitis with severe giant cell transformation of the hepatic cells and/or cholestasis [27, 29]. Liver biopsies from 25 individuals with a history of neonatal liver disease showed cellular and canalicular cholestasis with biliary rosette formation with variable degrees of parenchymal damage, little hepatocellular necrosis, but evidence of previous cell loss in the form of reticulin collapse. The last was severe enough to produce bridging collapse and/or prominent fibrosis-induced fragmentation of the specimen core in half of the individuals. In addition to lobular inflammation, four patients had hematopoietic activity and multinucleated giant hepatocytes. Portal edema and ductal proliferation were also observed. In early biopsy specimens, the identification of storage cells was difficult because of the presence of activated Kupffer cells loaded with ceroid and bile pigment [27, 29].
Liver biopsy specimens of 15 patients with a history of mild, persistent liver disease one to eight years after resolution of symptoms showed that inflammation and cholestasis had vanished (12 of 15), but variable degrees of inactive, post-necrotic fibrosis remained, with progression to cirrhosis in five. Storage material was noted in some hepatocytes and storage cells were identified in the parenchyma and to a lesser extent within portal and fibrous areas [27, 29]. In patients without a history of liver dysfunction, normal hepatic architecture was present, but there was obvious storage material in hepatocytes and Kupffer cells [27, 29].
Mutations of two genes, NPC1 and NPC2, are present in NPC. The NPC1 and NPC2 genes map to chromosomes 18q11-q12 and 14q24.3, respectively. The NPC1 gene product functions as an integral membrane transport protein in the lysosomal/late endosomal system for cholesterol, glycolipids, and other materials [23, 24, 25]. The NPC2 gene product is a soluble lysosomal cholesterol transporter. Abnormal function of the NPC1 and NPC2 proteins leads to accumulation of free cholesterol and secondary accumulation of sphingolipids within the lysosomes as well as a secondary sphingomyelinase deficiency. Additionally, delayed low density lipoprotein-mediated regulatory responses appear to contribute to the disease process [23, 25]. NPC1 mutations account for approximately 95% of NPC; NPC2 mutations account for the remaining 5%. Although mutations in both genes result in remarkably similar phenotypes, severe pulmonary disease is a unique complication in patients with NPC2 mutations and is a cause of early demise.
Because of the variability in phenotypes within this disease, NPC is not always initially suspected. The diagnosis is often delayed because of the absence of hepatosplenomegaly or storage cells in the bone marrow at initial presentation. However, NPC should be considered in all patients with idiopathic neonatal hepatitis [29]. A biochemical diagnosis can be made by the demonstration of impaired cholesterol esterification and positive filipin staining in cultured fibroblasts. Despite accumulation of sphingolipids in the lysosomes of affected cells, sphingomyelinase activity is normal. Molecular studies may also be employed for diagnosis but this method is often somewhat challenging, as many disease-causing mutations are private.
The treatment of patients with NPC relies upon symptom management, particularly the neurologic and psychiatric symptoms. Although BMT has been studied, this had no impact on the neurologic dysfunction and ultimate disease course. Likewise, liver transplantation improved hepatic function but did not appear to ameliorate CNS disease progression.
Various pharmacologic agents have also been studied as potential disease-specific therapies for NPC, including cholesterol-lowering therapies, sterol-binding agents, and neurosteroids, with varying degrees of success. Recently miglustat has been studied as a treatment for the neurologic manifestations observed in NPC. Miglustat is an N-alkylated imino sugar that functions as a competitive inhibitor of glucosylceramide synthase. Pre-clinical studies showed that miglustat is capable of crossing the blood–brain barrier and reducing excess storage of neurotoxic glycolipids, which are thought to contribute to the neurologic manifestations in NPC [32]. Clinical trials of miglustat in children and adolescents/adults indicated that the agent might have some efficacy at stabilizing the neurologic manifestations of NPC [31]. Indeed, based upon these studies, miglustat was approved by the European Union in 2009 for the treatment of neurologic manifestations in adults and children with NPC. However, a recent study of miglustat in a mouse model of Sandhoff disease (a lysosomal storage disease with CNS and visceral manifestations) showed unanticipated elevations in glycosphingolipids in the CNS despite delayed loss of motor function and coordination and extended lifespan [27]. This finding is contradictory to the expected finding of diminished levels of glycosphingolipids in the CNS. The significance of this finding is unclear, but it warrants further investigation to ensure the long-term safety of this agent, with particular emphasis on CNS disease, in patients with similar conditions.
Miglustat has not been demonstrated to have an effect on the visceral manifestations of the disease. Additionally, certain side effects, including gastrointestinal signs (e.g., osmotic diarrhea, weight loss, bloating, and belching), and neurological signs (e.g., tremor and peripheral neuropathy) are frequently reported in patients taking miglustat, similar to patients with Gaucher disease treated with miglustat.
Additionally, cyclodextrin has been explored as a potential therapeutic agent for NPC1.
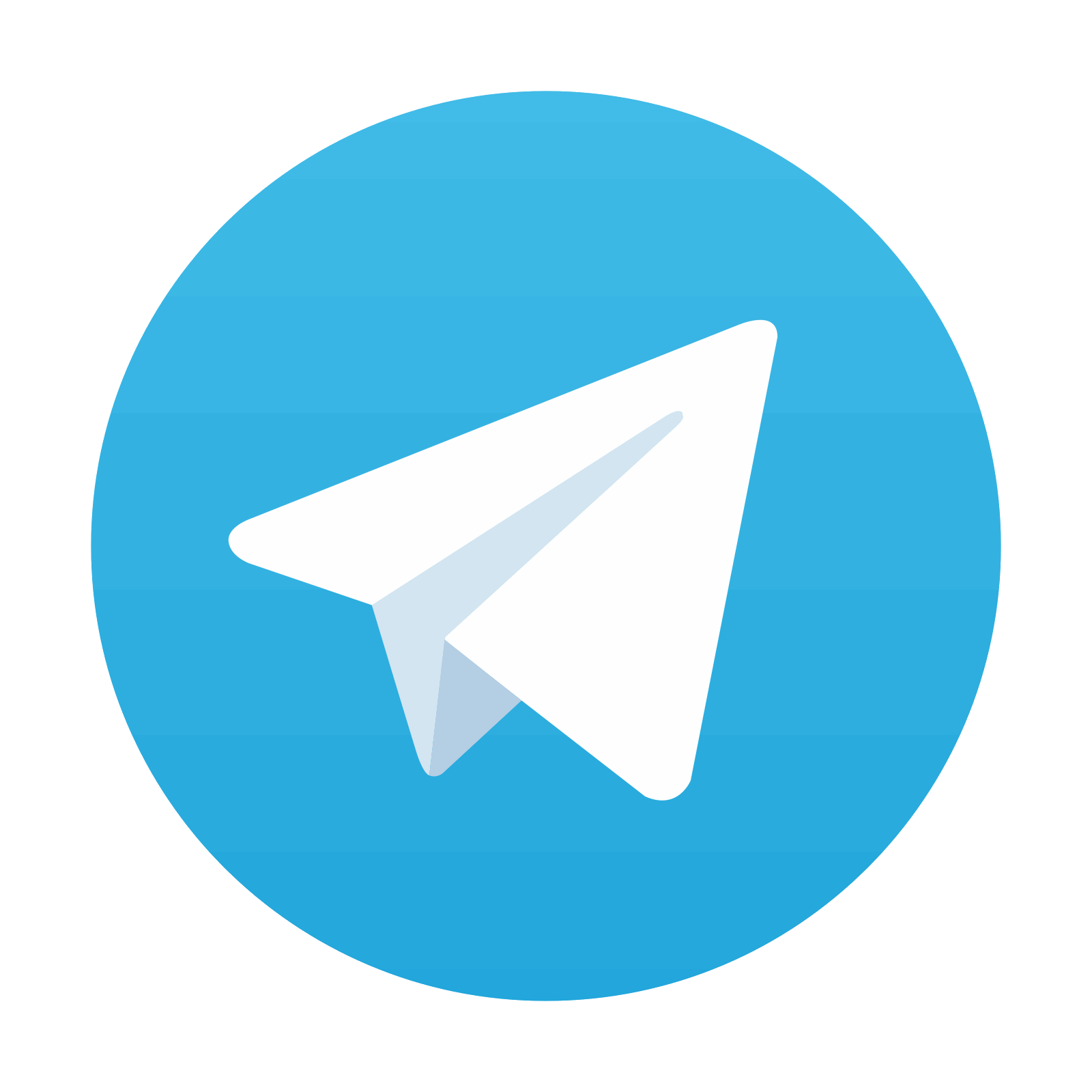
Stay updated, free articles. Join our Telegram channel

Full access? Get Clinical Tree
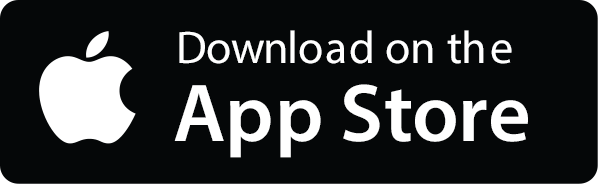
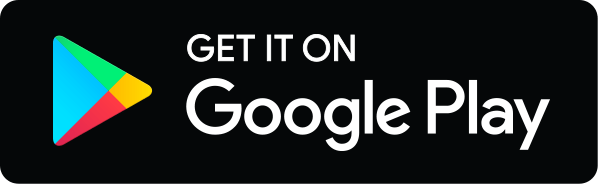