Abstract
Hepatorenal tyrosinemia1 is a severe inborn error of metabolism that can affect numerous organs, particularly the liver, kidneys, and peripheral nerves. In the first accounts of patients with features typical of tyrosinemia in the 1950s, almost all died of liver disease in infancy and childhood [1]. However, tyrosinemia is highly variable and rare case reports described surviving affected adults. Since the identification of tyrosinemia, its clinical course has been improved successively by the introduction of diet therapy, neonatal screening, liver transplantation and treatment with nitisinone (NTBC, 2-(2-nitro-4-trifluoromethyl benzoyl)-1,3-cyclohexanedione) [2]. Tyrosinemia raises questions in liver biology, biochemical and population genetics, cell biology, oncology, and public health.
Introduction
Hepatorenal tyrosinemia1 is a severe inborn error of metabolism that can affect numerous organs, particularly the liver, kidneys, and peripheral nerves. In the first accounts of patients with features typical of tyrosinemia in the 1950s, almost all died of liver disease in infancy and childhood [1]. However, tyrosinemia is highly variable and rare case reports described surviving affected adults. Since the identification of tyrosinemia, its clinical course has been improved successively by the introduction of diet therapy, neonatal screening, liver transplantation and treatment with nitisinone (NTBC, 2-(2-nitro-4-trifluoromethyl benzoyl)-1,3-cyclohexanedione) [2]. Tyrosinemia raises questions in liver biology, biochemical and population genetics, cell biology, oncology, and public health.
Throughout this chapter, we distinguish three clinical categories of patients. The first distinction is whether patients receive nitisinone treatment. Non-treated patients are at risk for classical acute hepatic and neurologic decompensations. Nitisinone-treated patients are distinguished as either early treated, i.e., before development of clinical hepatic signs, for example soon after newborn screening, or late treated. The amount of organ damage at the beginning of nitisinone treatment is felt to be a major risk determinant for chronic complications in liver and elsewhere. For deeper coverage, readers are directed to a detailed chapter [1] and a recent volume of reviews [3] and the references therein.
Pathophysiology
Tyrosinemia is caused by deficiency of the last enzyme of tyrosine degradation, fumarylacetoacetate hydrolase (FAH; enzyme classification [EC] 3.7.1.2, Figure 31.1A). This was deduced by Lindblad in 1977 [4] and subsequently confirmed directly [5]. FAH is a 419–residue cytosolic homodimer expressed in liver and to some extent in kidney, lymphocytes, erythrocytes, fibroblasts, and chorionic villi [1]. Early studies of tyrosinemic livers showed marked reduction of other enzymes of tyrosine degradation, particularly 4-hydroxyphenylpyruvate dioxygenase (4HPPD) [1], subsequently shown to be secondary to FAH deficiency.
(A) Degradation of phenylalanine and tyrosine. The metabolic intermediates, the degradative enzymes, and their associated inborn errors are indicated. Quantitatively minor pathways of tyrosine metabolism, not shown, include the biosynthesis of catecholamines and the biosynthesis of melanin pigments. These pathways are felt to be normal in tyrosinemia but have not been exhaustively studied.
(B) Succinylacetone, origin and effects. Succinylacetone is derived from intermediates that accumulate upstream from the primary enzyme block. Succinylacetone inhibits the synthesis of porphobilinogen from δ-aminolevulinic acid (δ-ALA). The resulting accumulation of δ-ALA is thought to cause the neurologic crises of tyrosinemia. Each solid arrow represents a single enzymatic step. In each panel, the site of inhibition by nitisinone is indicated..
The mechanism underlying the hepatic and renal symptoms of tyrosinemia is not perfectly understood, but biochemical and clinical observations suggest a toxic effect of the final compounds of tyrosine metabolism. Tyrosine and its early metabolites (4-hydroxyphenylpyruvate and homogentisate; Figure 31.1A) are present at high levels in other hereditary diseases that have no hepatic or renal symptoms; thus, these compounds are unlikely to cause the hepatorenal manifestations of tyrosinemia. In contrast, the substrate of FAH, fumarylacetoacetate, and its derivatives succinylacetoacetate and succinylacetone, have potent biologic activity. For example, fumarylacetoacetate and its precursor, maleylacetoacetate, resemble maleic acid, a toxin that can induce renal Fanconi syndrome, and histologic changes in renal tubules like those of tyrosinemia [6]. Maleylacetoacetate and fumarylacetoacetate are reactive, unstable compounds. Fumarylacetoacetate can form glutathione adducts [4], and free glutathione concentration is low in tyrosinemic liver [7]. Free sulfhydryl groups protect against free radicals and other toxic compounds. Nitisinone, an effective treatment, potently blocks tyrosine degradation upstream of maleylacetoacetate and fumarylacetoacetate.
Fumarylacetoacetate and maleylacetoacetate are undetectable in blood, suggesting that they are so reactive that they are transformed within their cell of origin before they can escape, i.e., a cell autonomous process. This concept leads to important predictions for tyrosinemia: a FAH-deficient cell, even if surrounded by cells with normal FAH activity and exposed to normal concentrations of tyrosine, will continue to produce fumarylacetoacetate, exposing itself to self-produced toxicity. A surprising phenomenon illustrates the toxic environment within tyrosinemic hepatocytes. Discrete nodules with normal FAH activity (“revertant nodules”) frequently arise in the livers of tyrosinemic patients [8]. These nodules result from back mutations in one FAH allele in individual cells, restoring enzyme function. In 25 tyrosinemic livers from French Canadian patients, 20 (80%) had revertant nodules that occupied up to 36% of the cross sectional area. Fumarylacetoacetate is a mutagen [8]. The high prevalence of a normally rare event (i.e., mutation of a single nucleic acid in the genome) suggests an extremely mutagenic environment within tyrosinemic hepatocytes. The expansion of the revertant nodules suggests a marked growth advantage of revertant cells over tyrosinemic cells.
Succinylacetone (SA), formed by reduction of fumarylacetoacetate or maleylacetoacetate, is stable and detectable in blood and urine. It is a sensitive and specific marker for tyrosinemia. It underlies the neurological crises of tyrosinemia (Figure 31.1B), by potently inhibiting the porphyrin synthetic enzyme δ-aminolevulinic acid dehydratase. δ-aminolevulinic acid is markedly elevated in non-nitisinone-treated tyrosinemic patients [9], as in acute intermittent porphyria, hereditary deficiency of δ-aminolevulinic acid dehydratase, and in lead poisoning [9], all of which feature painful neuropathies. All available evidence suggests that the neurologic crises of tyrosinemia result from blockage of hepatic porphyrin biosynthesis. They may represent the most severe hepatic porphyrin synthetic disorder in humans.
Several animal models of FAH deficiency develop hepatic and renal damage, including pigs [10], rabbits [11] and mice. FAH-deficient mice have a fulminant liver and kidney course causing neonatal death [12]. Nitisinone treatment permits growth to adulthood, but hepatic failure develops if nitisinone is withdrawn [13]. FAH-deficient mice are a frequently used model for studying gene therapy because of the growth advantage of hepatocytes with active FAH over deficient cells [14]. FAH-deficient mice are useful for rapid studies of tyrosinemia but mice and humans have numerous physiological differences including a high baseline risk of hepatocarcinoma in mice.
Genetics
Tyrosinemia is an autosomal recessive trait [15]. Therefore, for each pregnancy of a couple in which both partners are heterozygotes, there is a 25% risk of an affected child. Heterozygotes for hepatorenal tyrosinemia are asymptomatic and have normal levels of tyrosine-related metabolites.
A high prevalence of tyrosinemia is described in two regions: the province of Quebec, Canada, and northern Europe. In Quebec, tyrosinemia is prevalent due to a well-described founder effect [15] in the Saguenay-Lac St-Jean area of northern Quebec, where the carrier rate for tyrosinemia is one in 20. Measured birth incidence there was one in 1,846 and the theoretical birth incidence in Quebec is calculated to be 1 in 16,786 births. This is higher than recent observations, perhaps reflecting genetic counselling, identification of at-risk couples by carrier screening and availability of prenatal/preimplantation diagnosis for such couples. This compares to an estimated one in 100,000 to one in 120,000 elsewhere, including Scandinavia [16]. Tyrosinemia can occur in any ethnic group. Lack of French Canadian or Scandinavian ancestry does not exclude the diagnosis.
Many variants in FAH cause tyrosinemia [17]. The French-Canadian founder allele is a splice mutation, c.1062+6G>A, formerly designated IVS12+5g>a, accounting for ~90% of causal FAH alleles in the Saguenay, and is also frequent in patients of other origins [18].
Knowledge of FAH genotype does not usually explain clinical severity. One family with three affected children illustrates this: two died in infancy, one of a hepatic crisis, the other of a neurologic crisis; the surviving sibling is an adult with stable. renal tubulopathy and mild liver disease [19]. Clearly, environmental and/or genetic factors unrelated to the causal mutations are major determinants of clinical severity.
Conversely, mutations with detectable residual activity include variants associated with tyrosinemia with low excretion of SA [20] and also the pseudodeficient p.R341 W allele, which reportedly suffices to protect against liver pathology (see “Diagnosis” and “Differential Diagnosis,” below).
Newborn Screening
Newborn screening for tyrosinemia has been performed in Quebec since 1970, using samples of dried blood on filter paper. Because early treatment is effective (Figure 31.2), tyrosinemia screening is increasingly included in newborn screening panels around the world.
Figure 31.2 Liver pathology in tyrosinemia. Acute and chronic courses. (A) Macroscopic appearance of liver from a three-month-old girl with the acute form of tyrosinemia. The liver was firm, shrunken, and vaguely nodular. (B) Histologic examination of the liver revealed massive parenchymal collapse and fibrosis. There is cholangiolar proliferation, and surviving hepatocytes are frequently arranged in a pseudoglandular pattern. There is intracellular cholestasis and hemosiderin deposition as well as a mild, nonspecific chronic inflammatory infiltrate. (Hematoxylin-phloxine-saffron (HPS) stain, original magnification 125×.) (C) Enlarged, cirrhotic liver from a two-year-old boy with a chronic form of the disease reveals a coarsely nodular external and cut surface. (D) Low-power histologic examination reveals a mixed macro- and micronodular cirrhosis. The nodules appear histologically heterogenous because of variable degrees of fat content in the hepatocytes. (HPS stain, original magnification 30×.)
Succinylacetone is a specific and sensitive marker for tyrosinemia, and is the preferred marker for newborn screening. It is elevated at birth and even prenatally [1]. In contrast, elevated blood tyrosine levels are nonspecific, occurring in several conditions including hepatic dysfunction in general (see “Differential Diagnosis”), leading to false positives. Conversely, false-negative results (i.e., affected children with normal tyrosine level) increasingly occur in this era of low neonatal protein intake (breast-feeding, humanized formulas) and early hospital discharge. Both factors reduce the mean level of plasma tyrosine at screening.
Diagnosis
The diagnosis of tyrosinemia is established in a patient with consistent clinical findings, who has markedly elevated succinylacetone levels and/or both of whose FAH alleles contain a known pathogenic mutation. Elevations of tyrosine and of alpha-fetoprotein (AFP) are less specific. They were formerly used as diagnostic markers and are briefly discussed.
Succinylacetone
Succinylacetone is the most discriminating diagnostic marker. Marked elevations in urine or blood are present in the vast majority of tyrosinemic patients who are not treated with nitisinone. In nearly all cases, there is a clear difference between the reference range (plasma values up to 24 nmol/L are observed in normal individuals) and tyrosinemic patients (levels of 16,944 to 74,377 nmol/L were seen in 15 tyrosinemic patients identified by screening and taken before nitisinone treatment) [21, 22]. Such an increase is pathognomonic for hepatorenal tyrosinemia.
The discovery that succinylacetone is detectable in normal individuals is relatively recent [23]; the upper limit of the normal reference range is about 24 nanomol/L in plasma and 34 µmol/mol creatinine in urine [21, 22]. Many diagnostic laboratories do not perform specific testing for SA and report values below their threshold, often about 1 µmol/L in plasma, as “undetectable.” The concentration range defined by these two limits recently revealed two types of mild hypersuccinylacetonemia with normal liver function (see “Differential Diagnosis”). Also “Succinylacetone-negative” tyrosinemic patients are reported. Explanations include metabolism of SA within revertant nodules [24] and metabolic diversion to SA-like compounds such as 4-oxo-6-hydroxyheptanoate [20]. We have not observed a non-nitisinone-treated tyrosinemic patient who did not have clearly elevated levels of succinylacetone in blood and urine measured by specific testing. We speculate that some reported “succinylacetone-negative” tyrosinemic patients [20, 24], with typical liver dysfunction and “mild” pathogenic FAH mutations, may have levels of SA within this interval. All patients with values in this interval should be evaluated clinically, by specific SA assay and mutation analysis.
Tyrosine
Elevated plasma tyrosine is a nonspecific finding of little diagnostic use despite the name of this disease. Plasma tyrosine levels are initially elevated to a variable degree in almost all symptomatic tyrosinemic patients at clinical presentation, although older patients with a chronic course and patients treated with low-protein diets may have normal plasma tyrosine levels [16]. Hypertyrosinemia occurs in liver failure and in other inborn errors of tyrosine catabolism (see “Differential Diagnosis,” below, and Figure 31.1).
Plasma methionine levels also may be markedly elevated in tyrosinemia, and phenylalanine levels may be mildly elevated at diagnosis. Neither of these two amino acids are reliable diagnostic markers for tyrosinemia.
Alpha-Fetoprotein
Elevation of AFP levels is consistent with tyrosinemia, but is nonspecific. In non-nitisinone-treated patients, AFP levels are markedly elevated at screening. Normal AFP levels decrease greatly during the weeks after birth and accurate age-specific reference ranges must be consulted. With dietary therapy alone, AFP levels decrease variably but usually remain above normal. In a small fraction of non-nitisinone treated tyrosinemic patients, typically adolescents or young adults with mild hepatic disease and chronic renal disease, AFP can be normal; such patients remain at risk for hepatocarcinoma. AFP levels can increase acutely during hepatic crises, and then fall afterwards. In most but not all patients with hepatocarcinoma, AFP levels usually rise insidiously and progressively.
Molecular Diagnosis
Molecular diagnosis is useful for carrier detection in families and for prenatal diagnosis. In founder populations, molecular testing for common alleles is an appropriate first step. Even in such regions, rare mutant alleles exist. Complete sequencing of FAH exons and deletion-duplication analysis are available clinically. Knowing the mutations of both parents provides them with the option of molecular prenatal diagnosis.
For symptomatic patients, succinylacetone assay of a sample obtained prior to the administration of nitisinone is preferable to molecular diagnosis. Negative molecular testing, i.e., that lack of sequence variants or of identifiable copy number variation in the FAH gene, does not suffice to rule out the diagnosis of tyrosinemia, because some pathogenic alleles may not be detected.
Fumarylacetoacetate Hydrolase Assay
Fumarylacetoacetate hydrolase assay is no longer widely used for diagnosis. False positive and negative results are possible. FAH is expressed in lymphocytes and erythrocytes as well as in liver [5, 25]. In liver, the level of FAH activity can be falsely high if a revertant nodule is sampled. Conversely, low but measurable activity is sometimes sufficient to protect the individual from liver injury, as with the pseudodeficient allele, p.R341 W. Carrier detection by FAH assay is imperfect and molecular testing is preferred when the mutation is known. An important role for FAH assay is to determine the activity of uncharacterized missense FAH variants. These can be inserted into a human FAH cDNA, expressed in parallel with a normal FAH cDNA, and then the variant and normal FAH activities are compared.
Prenatal Diagnosis
Non-judgmental genetic counselling and, if the couple so requests, prenatal diagnosis, should be supervised by a team experienced in prenatal genetic testing. If the pathogenic mutant allele of each parent is known, molecular diagnosis is performed, permitting early prenatal or preimplantation diagnosis. Prenatal diagnosis is also possible by measurement of amniotic fluid succinylacetone and by FAH assay in amniocytes or chorionic villi [1, 25].
Differential Diagnosis
Clinical Differential Diagnosis
Tyrosinemia enters the differential diagnosis of any infant or child with unexplained hepatocellular lysis, cirrhosis, or decreased synthetic function (prolonged coagulation studies). Rare patients present with coagulopathy without overt liver disease [26]. Rarely, hepatocellular carcinoma may be the presenting sign [27]. Rickets or characteristic renal or neurologic findings, especially if associated with abnormal hepatic function, also suggest this diagnosis [16]. Renal tubular dysfunction in patients with hepatocellular failure is suggestive of tyrosinemia but also occurs in other hereditary metabolic diseases involving the liver, including galactosemia, hereditary fructose intolerance, Wilson disease, mitochondrial diseases and glycogen storage disease type 1 [28]. Typical neurologic crises in a patient with liver dysfunction, or a family history suggestive of tyrosinemia, also suggest tyrosinemia.
Hypertyrosinemia
Several inborn errors (Figure 31.1) and some acquired conditions cause hypertyrosinemia [16]. Moderate hypertyrosinemia occurs in hepatic failure and in normal subjects postprandially, with elevations of several other amino acids.
Tyrosinemia type II (oculocutaneous tyrosinemia, Figure 31.1) is caused by the autosomal recessive deficiency of tyrosine aminotransferase [1]. Patients present with hyperkeratosis of the palms and soles and painful lesions of the ocular cornea resembling ocular herpes infection. Untreated patients are at risk for intellectual deficiency. Hepatic and renal functions are normal.
Three disorders relate to 4HPPD. First, genetic deficiency of 4HPPD causes tyrosinemia type III [1]. Presentations vary from asymptomatic to intellectual deficiency and neurologic signs. It is not formally proven that this is a true clinical phenotype and may reflect an ascertainment bias (i.e., fortuitous discovery in patients evaluated for clinical signs caused by unrelated disorders). Second, transient tyrosinemia [1] occurs mainly in premature newborns with immaturity of 4HPPD, who receive large amounts of protein (e.g., cow’s milk), or who are deficient in vitamin C, the cofactor of 4HPPD. Unnoticed unless plasma amino acids are studied, hypertyrosinemia disappears within days to weeks. This can be hastened by vitamin C administration (50–100 mg/day) or by dietary restriction of phenylalanine and tyrosine. Transient tyrosinemia is generally felt to be benign although mild developmental delay is reported [29]. Third, Hawkinsinuria is a very rare autosomal dominant trait resulting from a specific dysfunction of 4HPPD. Affected infants may develop acidosis and hypertyrosinemia after weaning from breast milk and excrete an unusual urinary metabolite (hawkinsin) when symptomatic. Hepatic function and overall prognosis seem to be normal [1].
The two other inborn errors shown in Figure 31.1 cause neither liver disease nor hypertyrosinemia. Phenylketonuria, detected by high blood levels of phenylalanine at neonatal screening, can cause intellectual deficiency that is preventable by early dietary restriction to lower phenylalanine levels. Alcaptonuria causes arthritis in adults, darkening of urine when exposed to air and high urine homogentisate levels. Treatment of alcaptonuria with low-dose nitisinone is under study [30].
Hypersuccinylacetonemia
Marked hypersuccinylacetonemia is pathognomonic for tyrosinemia. Sensitive newborn screening has identified groups of asymptomatic newborns with mild hypersuccinylacetonemia [21, 22]. Two causes are known:1. a pseudodeficient FAH allele (p.R341 W) opposite a severely deficient allele leading to partial FAH deficiency and 2. deficiency of the enzyme preceding FAH, malonylacetoacetate isomerase. Plasma levels of succinylacetone have ranged from 59 to 1,282 nmol/L [21, 22]. Several fold less than in tyrosinemic patients at screening (see “Diagnosis”), these elevated levels fall in a zone reported as “negative” by many diagnostic laboratories. To date, these individuals have had normal liver function including coagulation tests.
Liver function is carefully assessed because rare cases of “succinylacetone-negative” tyrosinemia fall into this category and have typical liver dysfunction of tyrosinemia (see “Diagnosis”). For patients with mild hypersuccinylacetonemia and normal coagulation testing, we inform the parents of the currently normal clinical state, of the natural course of classical tyrosinemia and describe the implications of treatment with nitisinone and diet. To date, no families have chosen treatment, and all patients have retained normal liver function. Mild hypersuccinylacetonemia defines a borderline zone between disease and asymptomatic biochemical variation, recently identified and incompletely explored. All such patients should be evaluated for molecular cause and followed for hepatic function and general health.
Clinical Course in Non-Nitisinone-Treated Patients
This section describes the natural history of tyrosinemic patients not treated with nitisinone. Nitisinone therapy markedly improves the course (see “Treatment”). Liver, peripheral nerves and kidneys are the main organs affected by tyrosinemia.
The Liver
General Clinical Course
Before developing decompensation, patients can have mild to moderate hepatomegaly, synthetic failure with prolonged coagulation, elevated AFP and variable, often mild elevations of transaminases and normal bilirubin. Patients have traditionally been classified clinically as “acute” or “chronic.” Clinicians will realize that one patient may have both acute and chronic features. For instance, older children with a “chronic” course remain at risk for acute liver and neurologic crises.
Liver Crises
Liver crises are acute episodes of liver dysfunction, sometimes evolving rapidly to liver failure. They typically present before two years of age and decrease in frequency and severity thereafter. Acute episodes are often heralded by a viral infection, with anorexia, irritability, and vomiting. An odor resembling that of boiled cabbage may be detected. Acute crises carry a high risk for the development of liver failure with rapidly increasing hepatomegaly, ascites, anasarca, and marked coagulopathy. In contrast, jaundice is usually a late event and is absent in most patients. Historically, over 80% of tyrosinemic patients died from an acute liver crisis before two years of age, some presenting six to ten episodes during their first year [16, 31].
The first laboratory indication of an impending liver crisis is prolonged coagulation times [26, 31]. Prothrombin and partial thromboplastin times may be alarmingly prolonged despite normal or mildly elevated serum aminotransferase levels [1, 16]. These abnormalities also may be seen in clinically stable infants. They are generally unresponsive to oral or parenteral vitamin K supplementation but correct following infusion of fresh frozen plasma. Interestingly, factor V levels, frequently used in other liver diseases as a marker of liver synthetic function, may be normal or nearly normal in tyrosinemic infants in crisis, as are factor VIII levels. In contrast, factors XI and XII, and the vitamin K–dependent factors II, VII, IX, and X, may be exceedingly low (<15%).
The amino acids tyrosine and methionine are elevated. Serum AFP may be extremely high (up to 400,000 ng/mL), declining over weeks to months after the crisis.
Chronic Liver Disease
A chronic course occurred in less than 40% of Quebec patients but may have been more prevalent in northern Europe [16, 31]. Before nitisinone treatment, all surviving patients in Quebec eventually developed cirrhosis despite newborn screening and dietary management starting by three to four weeks of age [1], although at highly variable rates. After two years of age, hepatocarcinoma risk was a major preoccupation [1].
About 70% of chronic patients have clinical hepatomegaly, sometimes with splenomegaly, and rarely other signs of liver dysfunction like spider hemangiomas or clubbing. Rickets may be apparent in patients with renal disease.
Forty percent of our patients with a chronic course had abnormal coagulation parameters without overt bleeding [32]. Serum AFP was elevated in virtually all, ranging from 100–400,000 ng/mL (normal, <10 ng/mL). Serum aminotransferases were normal or mildly elevated, usually with normal levels of albumin and bilirubin and mildly increased serum γ-glutamyltransferase.
Box 31.1 describes the course of an 11-month-old patient with chronic liver disease and incipient liver failure.
This case history illustrates many points discussed in this chapter.
An 11-month-old boy whose family emigrated from a region without newborn screening for tyrosinemia, presented with a three-month history of failure to thrive. A community physician ordered abdominal ultrasound (showing hepatomegaly with diffuse hyperechogenicity and bilateral nephromegaly (9cm)) and a skeletal survey (osteopenia). At hospitalization, he was alert but irritable and his liver edge was palpated 6 cm beneath the costal margin. International normalized ratio (INR) was 1.53 (reference, 0.93–1.15), ALT 29 (5–25), AST 79 (5–70), GGT, 143 (3–43), total bilirubin 45 (0–14), direct bilirubin, 25 (0–4), phosphate 1.0 mmol/L (1.4–2.2), plasma tyrosine 319 µmol/L (43–308), and methionine 75 (14–38). Repeated hypoglycemia occurred, requiring frequent feedings. Urine revealed glycosuria and generalized aminoaciduria. Succinylacetone was markedly elevated in urine (356,000 µmol/mol creatinine, reference 0–34) and blood (28,000 nanomol/L, reference 0–24), diagnostic for tyrosinemia. Nitisinone and diet treatment were prescribed.
Renal tubular function normalized within days. Kidney size was normal six months later (7.5 cm). Routine liver function tests normalized over weeks to months. Adherence to diet and nitisinone was excellent. He resumed normal growth, had good general health and obtained high marks in elementary and high school, excelling as well in sports and music.
Alpha-fetoprotein (AFP), initially 129,000 µg/L, reached <10 µg/L after 45 months of treatment. Abdominal imaging was normal. At 11 years of age, mild elevation of AFP appeared (14 µg/L) and slowly progressed. Ultrasound and MRI imaging were normal. Between 15 years 10 months (AFP, 51 µg/L) and 16 years 1 month of age (AFP, 264 µg/L), a 5 mm diameter nodule appeared in segment VIII on abdominal magnetic resonance imaging. At transplantation at 16.5 years, AFP was 3,459 µg/L. Segment VIII contained a well-differentiated hepatocarcinoma, 15 mm in diameter and enclosed in a fibrous capsule, with thickened plates of cancer cells in pseudoacinar formations, filled with eosinophilic material. The surrounding parenchyma showed grade F2–F3 fibrosis.
Risk of Hepatocarcinoma
The risk of hepatocarcinoma is high, and it can occur before two years of age [33]. The incidence has been difficult to estimate because systematic autopsies were not performed in all children dying of the disease. Even at autopsy, livers have not always been examined in detail.
Hepatocarcinoma or high grade dysplasia is reported in 28–75% of livers at transplantation. In 1976, Weinberg et al. [34] reviewed all 43 published and personal cases of tyrosinemia published, finding hepatocarcinoma in 37%. In 14 of these from the older literature, the presumed diagnosis of tyrosinemia was not always documented pathologically. A recent Brazilian series of non-screened patients reported hepatocellular carcinoma in 12 of 16 transplanted patients (75%) and cirrhosis in all [35]. At CHU Sainte-Justine, since 1986, 32 tyrosinemic livers have been studied at autopsy or transplantation (Table 31.1). In 24 (75%), nodules had been identified by imaging. Five (15%) showed one or more foci of hepatocarcinoma and four had high-grade dysplasia. The series differ in several respects. The incidence of hepatocarcinoma in in earlier-diagnosed and treated tyrosinemia patients with evidence of nodules on imaging will be less than reported in these series. Not all nodules evolve to malignant or premalignant lesions, which may have implications when considering the indication of liver transplantation for children with clinically stable disease. However, the presence of nodules on imaging calls for close follow-up of serum AFP levels, serial imaging to document stability and possible biopsy of accessible nodules.
Table 31.1 Liver Transplantation of Tyrosinemic Patients, Prior to the Availability of Nitisinone Treatment
Patient | Age at OLT (years) | Nodules/HCC or Dysplasia* | Outcome |
---|---|---|---|
1 | 1⅔ | −/Dysplasia grade 1 | Death, primary nonfunction |
2 | 8 | −/− | A&W |
3 | 9 | −/− | A&W |
4 | 3½ | +/HCC and dysplasia grade 3 | A&W |
5 | 2 | +/Dysplasia grade 3 | A&W |
6 | 10 | +/− | A&W |
7 | 1½ | +/Dysplasia grade 1 | A&W |
8 | 12 | +/− | A&W |
9 | 1½ | −/Dysplasia grade 1 | A&W |
10 | 17 | −/− | Liver/kidney, A&W |
11 | 6 | +/Dysplasia grade 2 | A&W |
12 | 3 | −/Dysplasia grade 2 | A&W |
13 | 2 | −/Dysplasia grade 1 | A&W |
14 | ⅓ | +/Dysplasia grade 2 | Death, primary nonfunction |
15 | 9½ | +/Dysplasia grade 3 | A&W |
16 | 1 | +/Dysplasia grade 3 | A&W |
17 | 2½ | +/Dysplasia grade 1 | A&W |
18 | 2½ | +/Dysplasia grade 1 | A&W |
19 | 11 | +/HCC and dysplasia grade 3 | A&W |
20 | ½ | +/Dysplasia grade 2 | A&W |
21 | 3 | +/Dysplasia grade 1 | A&W |
22 | 1112![]() | +/Dysplasia grade 3 | A&W |
23 | 2 | +/Dysplasia grade 2 | A&W |
As in other liver diseases, serial serum AFP levels in tyrosinemic patients are useful but do not always predict the presence of carcinoma. AFP levels often increase following liver crises, possibly reflecting regeneration or fatty nodules (Figure 31.3). Some tyrosinemia patients with hepatocarcinomas have normal AFP levels [32]. A high degree of suspicion of hepatocarcinoma must be maintained if AFP levels sequentially increase in a clinically stable patient in the absence of another explanation (Box 31.1). If a nodule is visualized radiologically or by ultrasonography, imaging characteristics cannot reliably rule out malignancy (see “Imaging in Tyrosinemia”).
Figure 31.3 Dysplasia, carcinoma, and regenerative nodules. (A) Histologic examination of native liver from a one-year-old patient undergoing a liver transplantation revealed multiple foci of dysplasia. This photomicrograph shows the small cell variant, composed of fetal hepatocyte-like cells with increased nucleocytoplasmic ratio and hyperchromatic nuclei. (HPS stain, original magnification 200×.) (B) In the liver from the same patient, foci of large cell dysplasia are shown, characterized by irregular hyperchromatic nuclei and a preserved nucleocytoplasmic ratio. Distinction from microscopic foci of hepatocellular carcinoma may be very difficult. (HPS stain, original magnification 200×.) (C) Native liver from a four-year-old hepatic transplantation patient showing cirrhosis and one nodule that clearly stood out from the rest of the parenchyma. (D) Histologic examination of the nodule in panel C, revealing hepatocellular carcinoma with extensive nuclear irregularity and many mitoses. (HPS stain, original magnification 200×.) (E) Native liver from an 11-year-old liver transplant recipient with extensive macronodular cirrhosis; one large focus of hepatocellular carcinoma was noted (pale staining nodule, lower portion of figure). (HPS stain, original magnification 20×.) (F) Revertant nodules. This section is adjacent to that in (E). Immunostaining with an antibody to FAH revealed a positive reaction in many of the regenerating nodules, indicating reacquisition of the missing enzyme by the regenerating hepatocytes. Note that the carcinomatous nodule remains negative, indicating absence of reversion. (Avidin-biotin-peroxidase technique with hematoxylin counterstaining, original magnification 20×.)
Hepatoblastoma is also reported in tyrosinemia [36]. Because its treatment differs from that of hepatocarcinoma, this rare possibility is considered when evaluating liver masses in tyrosinemia.
Neurologic Crises
Suspected neurological crises should receive immediate treatment with nitisinone (see “Management”). This section describes their natural history prior to availability of nitisinone.
Neurologic crises are a hallmark of non-nitisinone-treated tyrosinemia. They can occur in patients who stop taking nitisinone (see “Complications”). Crises have two phases: (1) an active period of painful paresthesias, autonomic signs such as hypertension [9], tachycardia, and sometimes progressive paralysis; and (2) a period of recuperation, seen after crises with weakness or paralysis. In a series of 48 French Canadian patients, 20 (42%) had crises, higher than previous reports [9]. Crises may truly be less frequent elsewhere, or perhaps they were underreported in earlier series.
Pain is the most frequent sign. During the prodrome, often following a minor infection, the child is irritable and less active than usual. The mildest symptoms are nondescript discomfort or pain in the abdomen or legs. Fully developed crises cause severe pain, often in the legs. Patients frequently adopt a position of extreme hyperextension of the trunk and neck, which can be mistaken for opisthotonus or meningismus. Older patients have claimed that this alleviates the pain somewhat. This hypertonia is distinguished from tonic convulsions, because the patients are conscious. True convulsions also may also occur, for instance in association with severe hyponatremia secondary to inappropriate antidiuretic hormone secretion. The dramatic nature of painful neurologic crises is conveyed by Box 31.2, written by the mother of a patient and reproduced from the previous edition [37].
Box 31.2 Neurologic Crises, Described by a Parent
For two or three days before the crisis, P would sleep fitfully with increasing crankiness. No position seemed comfortable, and he became more unsteady on his feet. His face was drawn and pale. His appetite was poor, and he was always nauseous. His belly became bloated. He lost interest in playing and was very sensitive to the slightest touch. This was followed by periods of intense pain for about three days, which decreased in frequency over the next few days. When he felt the pain coming, he would place his forearms under his chin, and would tense up and tremble. He would screw up his face and wring his hands to the point of causing bruises. He would arch his back until his head touched his heels, tear out his hair, pull out his teeth, and bite his cheeks and lips despite all the bandages we had placed to prevent him from hurting himself. He would shriek a lot but was drowsy most of the time. He would throw up a lot, and he usually had some fever. He would lose about two to three pounds with every crisis. It would take two to three weeks for him to get back to his usual state. When I asked him what the pain felt like, he would say there were two types: one was like he had large needles in his muscles and bones everywhere and the other as if he were being squeezed in a vice.
Weakness or paralysis occurred in about one-third of crises in our series. In eight of 104 crises, mechanical ventilation was necessary because of respiratory weakness, in one case for more than three months. Electrophysiologically, patients showed evidence of axonal degeneration, with normal nerve conduction velocity but decreased wave amplitude, and an increased threshold of stimulation, progressing to absence of peripheral nerve function. Recuperation from paralytic crises is possible, although patients with repeated severe crises may have chronic weakness. Patients in whom oral anesthesia develops as part of a crisis may seriously lacerate their tongue and develop severe bruxism, to the point of dislodging their teeth.
Hypertension and sustained tachycardia are common during the initial phase of crises, as are electrolytic imbalances, especially in children with tubulopathy. Vomiting and ileus occur frequently and may complicate nutritional management. The active phase of crises usually lasted for one to seven days.
Of note, the neurologic crises of tyrosinemia are not usually associated with worsening of plasma transaminases, prothrombin time, bilirubin and succinylacetone levels during crises when compared with values observed between crises. Urinary levels of δ-aminolevulinic acid tended to be higher during crises than between crises, but in our retrospective series, this had little value for diagnosis of a neurological crisis or for predicting its severity. Routine cerebrospinal fluid analyses are normal during neurologic crises [9]. In some cases, catecholamine excretion is increased.
Neurologic crises are a major pathology in non-nitisinone-treated patients. There is an appreciable risk of death, particularly in paralytic crises. In our series of 20 children who had experienced at least one crisis, 11 of 14 deaths occurred during crises, and all 11 were associated with the complications of respiratory insufficiency [9]. All tyrosinemic children who are not receiving nitisinone therapy should be observed closely during intercurrent illness for the signs of neurologic decompensation, particularly respiratory insufficiency, because it may develop rapidly, thus children with signs suggestive of an impending neurologic crisis should be hospitalized.
Importantly, coma is not a feature of isolated neurologic crises. The level of consciousness remains normal except if suppressed by analgesics or sedatives. Coma in a tyrosinemic patient should evoke other causes requiring treatment, particularly liver failure with encephalopathy. Conversely, patients with paralysis who require intubation may be misdiagnosed as being comatose.
Renal Disease in Tyrosinemia
Renal involvement is frequent in non-nitisinone-treated tyrosinemic patients [1, 16]. Both renal tubular dysfunction and glomerular involvement may occur. The spectrum ranges from normal renal function to overt renal failure. Even patients with no evidence of renal disease, including an intact glomerular filtration rate (GFR), may show fibrosis on biopsy (see “Pathology”). Renal dysfunction may worsen during periods of decompensation. Generalized aminoaciduria and glycosuria are usually asymptomatic. Rickets is the main clinical sign of tubular dysfunction in tyrosinemia and figured prominently in early clinical descriptions [38]. At least three mechanisms may be implicated: urinary phosphate loss, impaired hepatic hydroxylation of vitamin D, and impaired renal hydroxylation of vitamin D. Rickets occurred in some patients in the pre-nitisinone era.
Clinical evidence of proximal tubular dysfunction was present in ten of 37 patients (27%) evaluated at CHU Sainte-Justine. Over 80% of tyrosinemic children evaluated at CHU Sainte-Justine had detectable nephromegaly on ultrasonography, and 33% had mild to moderate nephrocalcinosis (Table 31.2). Chronic renal failure may occur in adolescent and young adult tyrosinemic patients. In the CHU Sainte-Justine series, assessment of glomerular filtration by diethylenetriamine pentaacetic acid (DTPA) clearance generally showed low values, but with a wide range of severity (Table 31.2). All patients received a phenylalanine- and tyrosine-restricted diet and some received nitisinone which may have affected this incidence. Nitisinone often corrects tubular dysfunction (Box 31.1), but some may be permanent. An adult patient in Quebec, who had chronic tubular and glomerular dysfunction before starting nitisinone at age 21 years, has persistent tubular dysfunction, but GFR has not declined under treatment. Liver transplantation may correct the renal metabolic abnormalities in many cases, but some biochemical anomalies of renal tubular function may persist [39].
Table 31.2 Renal Evaluations of 37 Tyrosinemic Patients
Patient | Age (years) | Nephromegaly | Nephrocalcinosis | GFR Pretransplantation, (mL/min/1.73/m2*) |
---|---|---|---|---|
1 | 0.7 | + | + | 40 |
2 | 8 | + | − | 69 |
3 | 9 | + | − | 137 |
4 | 3.5 | + | + | 142 |
5 | 1.5 | + | − | 105 |
6 | 9.7 | + | − | 47 |
7 | 1.5 | + | − | 64 |
8 | 18 | + | + | 34 |
9 | 8 | + | + | 36 |
10 | 12 | + | − | 83 |
11 | 2.0 | + | − | 108 |
12 | 18 | + | + | 27 |
13 | 6 | + | − | 52 |
14 | 2.0 | − | − | 173 |
15 | 2.5 | − | − | 73 |
16 | 2.8 | + | − | ND |
17 | 3.5 | + | − | ND |
18 | 1.9 | − | − | ND |
19 | 8 | + | + | ND |
20 | 1.8 | + | − | ND |
21 | 9 | + | + | 117 |
22 | 0.3 | + | − | 82 |
23 | 4 | + | + | 93 |
24 | 6 | + | + | 65 |
25 | 2.0 | + | − | 86 |
26 | 0.7 | + | − | 182 |
27 | 0.9 | + | + | 155 |
28 | 2.0 | − | − | 147 |
29 | 2.0 | + | + | 125 |
30 | 0.5 | − | − | 141 |
31 | 2.0 | + | − | 107 |
32 | 9 | − | − | 124 |
33 | 2.0 | + | + | 90 |
34 | 2.5 | − | − | 116 |
35 | 4 | + | − | 128 |
36 | 1.0 | − | + | 120 |
37 | 3.0 | − | − | 128 |
* GFR measured by the DTPA method.
GFR: glomerular filtration rate; ND: not determined; −: absent; +: present.
Other Clinical Manifestations
Hypoglycemia
Some infants have episodes of hypoglycemia that require treatment. Monitoring from birth of some patients known prospectively to have tyrosinemia has shown instability of blood glucose levels sometimes requiring intravenous glucose infusion for several days. Although we cannot eliminate a persistent tendency to hypoglycemia in some patients, this is unusual and calls for evaluation for causes unrelated to tyrosinemia. For instance, a Quebec patient with recurrent fasting hypoglycemia proved also to have genetic deficiency of glycogen synthetase. One tyrosinemic child was reported to have insulin-dependent diabetes mellitus; the relationship of this patient’s diabetes to tyrosinemia is uncertain, and pancreatic histology in this case was normal (see “Pathology”).
Cardiomyopathy
Clinically significant hypertrophic cardiomyopathy is reported to be frequent in tyrosinemia and to respond to nitisinone treatment [40]. We have not observed this complication despite systematic evaluations of more than 100 patients, but physicians should be alert to this possibility.
Imaging in Tyrosinemia
Liver
This section describes our experience before and after the availability of nitisinone. It is important to note that not all nodules are detectable by imaging. In cirrhotic livers examined directly after transplantation or at autopsy, only a minority of the nodules were detected in previous ultrasonograms or scans.
In 30 patients evaluated before nitisinone treatment, ultrasonography was more sensitive than CT in the detection of liver changes in tyrosinemia [41]. The sensitivity is highly dependent on the experience of the ultrasonographer. Ultrasonographic examination is more difficult to standardize than are CT or MRI scans. The earliest ultrasonographic change, which may be subtle, is an inhomogeneity of the liver parenchyma without identifiable nodules. Inhomogeneity is often the first sign of micronodular cirrhosis but also may be a transient finding. We speculate that transient ultrasonographic inhomogeneity (e.g., [42]) represent multiple small foci of fatty change, which we have observed in some biopsies. Subsequently, in the development of cirrhosis, hypoechogenic micronodules (<5 mm) appear, followed by macronodules (≥5 mm) that may be either hyper- or hypoechogenic. In our pre-nitisinone series [41], the echodensity of nodules was not predictive of the presence of dysplasia or malignancy. If portal hypertension is suspected, Doppler ultrasonography should be performed.
Computed tomography scans reveal most macronodules. Of technical note, some nodules are obscured by contrast studies whereas the detection of others is enhanced.
Our experience with MRI of tyrosinemic nodules is limited because systematic MRI evaluation was introduced when nitisinone became available, and cirrhotic changes have been rare thereafter. MRI can provide useful information about regenerative, dysplastic or hepatocarcinomatous nodules. Sensitivity of detection is increased when a paramagnetic contrast agent such as mangafodipir is used. This liver-specific contrast agent is taken up by hepatocytes and excreted into bile. Nodules of functioning hepatocytes, such as in regenerative nodules, show homogeneous enhancement, whereas some hepatocellular carcinomas show reduced enhancement with this agent. This technique has been applied to one reported tyrosinemic patient who had multiple hepatic nodules and in whom the hepatic nodularity regressed and AFP levels fell when adequate nitisinone treatment was instituted [43]. The nodules enhanced normally and were felt to be benign. The positive predictive value of this method has not been estimated. Diffusion-weighted imaging has been reported to be more sensitive than T2-weighted imaging for the detection of hepatocarcinoma [44], but no imaging method can eliminate hepatocarcinoma with certainty. Our approach to monitoring for hepatic nodules in tyrosinemia is discussed later (see “Monitoring Medical Treatment” and “Chronic Liver Disease”).
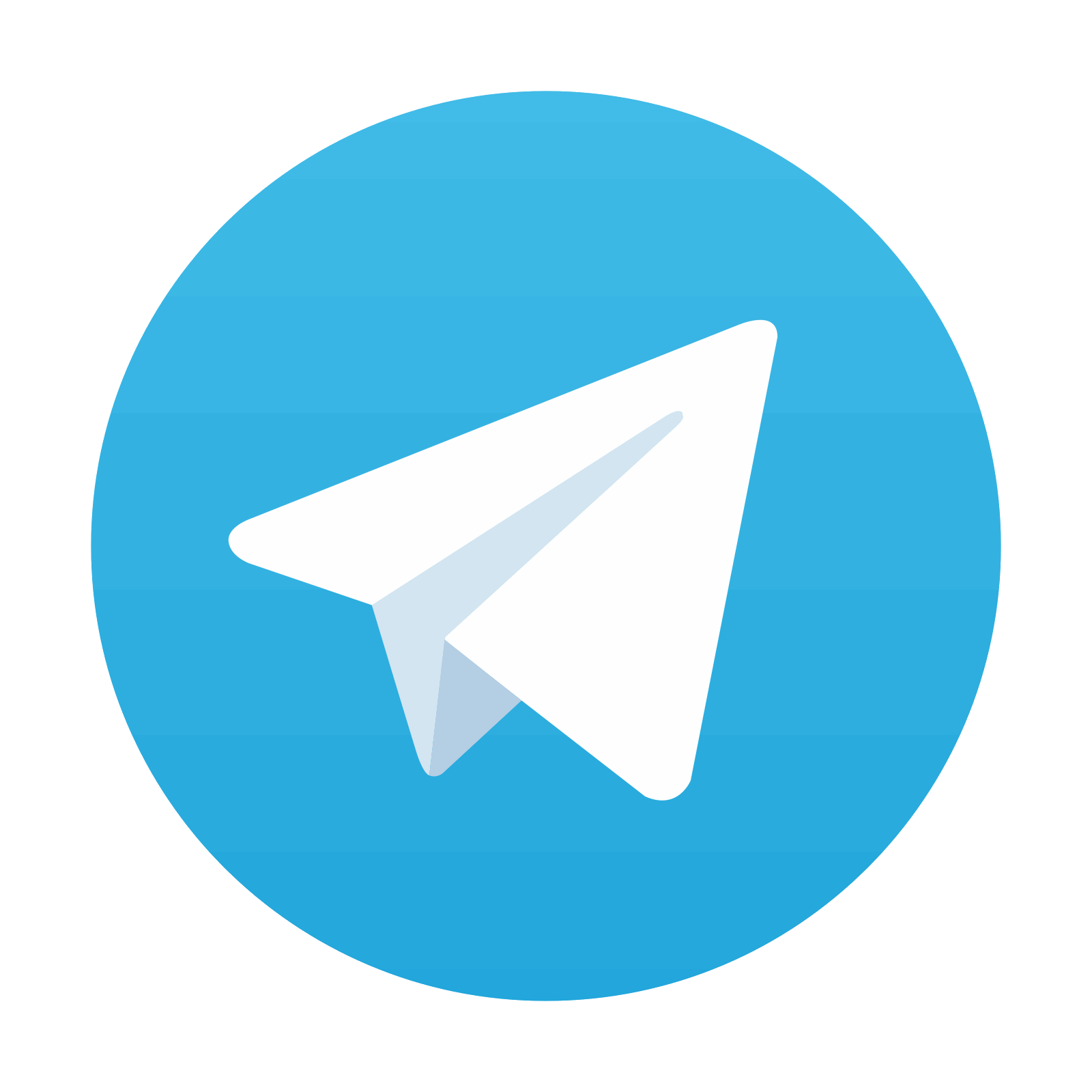
Stay updated, free articles. Join our Telegram channel

Full access? Get Clinical Tree
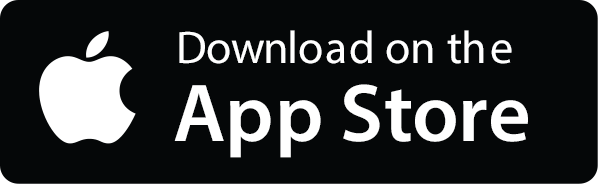
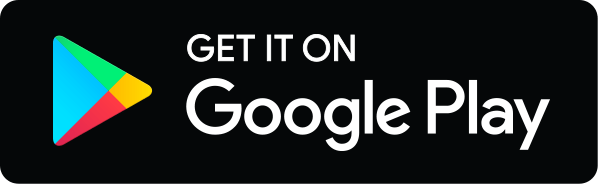
