Abstract
Iron overload states can be classified as primary or secondary. There are many disorders that can lead to iron overload (Table 29.1) [1, 2]. This chapter focuses on hereditary hemochromatosis (HH), juvenile hemochromatosis (JHH), and secondary iron overload (primarily transfusion associated) in the pediatric patient and in neonatal hemochromatosis (NH).
Iron Overload Disorders
Iron overload states can be classified as primary or secondary. There are many disorders that can lead to iron overload (Table 29.1) [1, 2]. This chapter focuses on hereditary hemochromatosis (HH), juvenile hemochromatosis (JHH), and secondary iron overload (primarily transfusion associated) in the pediatric patient and in neonatal hemochromatosis (NH).
Type | Disorders |
---|---|
Primary | Hereditary hemochromatosis |
HFE-associated | |
C282Y/C282Y homozygotes | |
C282Y/H63D compound heterozygotes | |
Other HFE mutations | |
Non–HFE-associated | |
Transferrin receptor-2 (TFR2) Juvenile Hemojuvelin (HJV) | |
Hepcidin (HAMP) | |
Ferroportin (SLC40A1) (autosomal dominant) | |
Secondary | Iron-overload anemias |
Thalassemia major | |
Sideroblastic anemia | |
Hemolytic anemias (spherocytosis, sickle cell disease, pyruvate kinase deficiency) | |
Aplastic anemia | |
Parenteral iron overload | |
RBC transfusions | |
Iron dextran injections | |
Long-term hemodialysis | |
Chronic liver diseases | |
Hepatitis B and C | |
Non-alcoholic fatty liver disease | |
Porphyria cutanea tarda | |
Other: Cystic fibrosis, tyrosinemia | |
Gestational alloimmune liver disease | |
Miscellaneous | |
Aceruloplasminemia | |
Congenital atransferrinemia |
Physiology and Pathophysiology of Iron Overload
Iron is one of the more tightly regulated nutrients in the body. Humans have no significant excretory pathway for iron. Therefore, body iron stores are normally controlled at the level of absorption, matching absorption to physiologic requirements. Under normal circumstances, only about 1 mg of elemental iron is absorbed each day (Figure 29.1), in balance with gastrointestinal losses. Intestinal iron absorption is increased by low body iron stores (storage regulation), increased erythropoiesis (erythropoietic regulation), anemias associated with ineffective erythropoiesis (thalassemias, congenital dyserythropoietic anemias, and sideroblastic anemia), and acute hypoxia. Both dietary iron intake (dietary regulation) and systemic inflammation can temporarily decrease iron absorption and availability, even in the presence of iron deficiency [3].
Duodenal crypt cells sense body iron status and are programmed for iron absorption as they mature. Duodenal and proximal jejunal enterocytes are responsible for iron absorption. Low gastric pH helps to dissolve iron, which is then enzymatically reduced to the ferrous form by ferrireductase. Divalent metal transporter 1 transfers iron to the enterocyte, where it is either stored as ferritin or moved across the basolateral membrane to reach the plasma, where it is rapidly oxidized to the ferric form by hephaestin and bound to transferrin. Divalent metal transporter 1 levels are altered in response to body iron stores [3].
Hepcidin and ferroportin are the key regulatory proteins for total body iron status. Hepcidin is produced in the liver, and its expression and secretion in iron sufficiency is elevated. Hepcidin acts to downregulate the cell surface expression of ferroportin, a transmembrane iron transporter that acts to transfer iron out of intestinal epithelial cells and macrophages [4]. During times of low iron status, hepcidin is low and ferroportin expression on the basolateral membrane of the enterocyte is maintained. As a consequence, intestinal epithelial cells transport more dietary iron across their basolateral membranes, leading to increased iron absorption. Mutations in the genes for both hepcidin (JHH) and ferroportin (autosomal dominant hemochromatosis) have been described [1, 2].
Hepcidin expression is regulated by the HFE protein (mutated in HH), transferrin receptor 2 (TFR2), and hemojuvelin (mutated in JHH). Disruption of iron regulation by hepcidin is the central mechanism for iron overload in the various forms of hemochromatosis (Figure 29.2). In animal models and in humans with hemochromatosis, the normal increase in hepcidin expression with iron loading is lost, leading to lower hepcidin levels and continued iron absorption in the face of iron overload [5].
Figure 29.2 Proposed roles of hepcidin, HFE, transferrin receptor 2 (TFR2), and ferroportin in iron absorption. In states of high iron stores and inflammation, signaling from HFE, TFR2, and hemojuvelin (HJV) leads to increased hepcidin synthesis. Hepcidin results in phosphorylation and internalization of ferroportin in both enterocytes and macrophages, reducing transport of iron. In contrast to hepatic TFR2, expression of TFR1 is regulated by iron-binding proteins in response to dietary iron intake.
In humans, iron in the circulation is tightly bound to transferrin, which can bind up to two molecules of ferric iron (diferric transferrin). In homeostasis, about 30% of the binding sites on transferrin are normally occupied by ferric iron. Diferric transferrin binds to the transferrin receptor 1 (TFR1) on the cellular plasma membrane. This complex is then endocytosed into the cell, where iron is released by the acid environment of the endocytic vesicle. The iron is then transported across the endosomal membrane to the cytoplasm. Therefore, in the setting of a highly saturated transferrin (e.g., HH), more of the transferrin is in the diferric state and more readily absorbed.
The uptake of iron is primarily regulated by the expression of TFR1 on the cell surface. In iron deficiency, iron regulatory proteins are increased and bind to the TFR1 iron-responsive elements in the 30-untranslated region of the TFR1 mRNA. This stabilizes the TFR1 transcript, leading to increased TFR1 expression on the cell surface, increasing iron uptake. Simultaneously, these same iron regulatory proteins bind to the 50-untranslated region of ferritin (the iron storage and intra-cellular sequestration molecule), decreasing its synthesis. In states of iron repletion or excess, there is a reduced level of iron-binding proteins, leading to less TFR1 production and an increase in ferritin and hepcidin synthesis. In addition, the HH protein HFE has been shown to associate with TFR1 on the cell surface and decrease the affinity of TFR1 for diferric transferrin in the setting of iron overload. In the intestine and at other sites, iron is also transported by at least one transporter that is independent of transferrin and TFR1. An active transporter for divalent cations, including iron, called the divalent cation transporter 1, has been reported. Its mRNA is strongly expressed in the enterocytes at the villous tips and is increased in states of iron deficiency.
The second receptor TFR2 binds holotransferrin/diferric transferrin and mediates the uptake of transferrin-bound iron. This protein is predominately expressed in the liver, where, in contrast to TFR1, it is not downregulated by dietary iron overload nor is it in the mouse model for HH. Hepatic TFR2 provides an explanation for the continued hepatic iron uptake in HH despite the downregulation of TFR1. Hepcidin synthesis in the liver is also regulated by TFR2.
In HH and JHH, net iron absorption is increased above endogenous losses. In addition, in both disorders, there is loss of the normal downregulation of iron absorption as iron accumulates in the body. This is due to mutations affecting hepcidin regulatory proteins that normally promote hepatic hepcidin expression [5, 6]. The result is a net gradual increase in total body iron. In HH, the net increase in total body iron has been estimated at 4–7 mg/day. The increased iron absorption in HH is the result of inappropriate transfer of iron at the basolateral surface of the enterocyte because of suppression of hepatic hepcidin secretion [6]. In JHH, iron accumulates more rapidly than in HH, in part because of the more significant role of hemojuvelin in the regulation of hepcidin [7]. Excessive iron intake in the diet can accelerate the accumulation of iron in both HH and JHH. In a similar manner, increased iron losses (most commonly in menstruating females) can slow the accumulation of iron. In secondary iron overload caused by the hemoglobinopathies, overload is related to both the anemia and increased iron absorption and the excess iron provided by transfusions. In contrast, in aplastic anemias, iron overload is primarily related to frequent red blood cell transfusion.
Hereditary Hemochromatosis (OMIM 235200)
Genetics
Hereditary hemochromatosis has long been recognized as an autosomal recessive disorder. In 1976, it was linked to the human leukocyte antigen (HLA) region of the short arm of chromosome 6. In 1996, using positional cloning in a defined cohort of patients with HH, the candidate gene for HH, called HFE, was discovered [8]. HFE encodes a novel major histocompatibility (MHC) class I-like molecule. Two principal missense mutations of HFE have been identified. One results in a change from a cysteine to tyrosine at position 282 (C282Y). A second mutation results in a change from a histidine to aspartate at position 63 (H63D). Upward of 85–90% of patients with classic HH are homozygous for C282Y, and another 5% are compound heterozygotes (C282Y/H63D). The H63D mutant is quite common (15–20% heterozygote state in the general population), but H63D homozygotes generally do not have significant iron loading. It is also important to note that, in the majority of studies, 10–15% of patients with a clinical syndrome of typical HH do not have either the C282Y or H63D mutation. Of these, 55% of patients were found to have previously unrecognized causes for secondary iron overload. However, there remains a subgroup of patients with typical HH that lack either of the two common mutations. Some of these patients have mutations in other hepcidin regulatory proteins and others have different mutations in HFE.
Both common mutations of HH result in alteration to the protein complex that regulates hepcidin expression. The C282Y mutant protein does not associate with β2-microglobulin (β2 m), which is essential for correct intracellular trafficking and transport of HFE to the plasma membrane. This results in reduced cell surface expression of HFE–β2 m complex and failure to associate with TFR1. The C282Y mutant protein also fails to undergo late Golgi processing and is retained in the endoplasmic reticulum, undergoing accelerated degradation. In contrast, the H63D mutation has no effect on the binding of β2 m or cell surface expression of HFE. Instead the H63D mutant does associate with TFR1 but its binding decreases the affinity of TFR1 for diferric transferrin less than when bound to wild-type HFE.
HFE is widely expressed throughout the body; the highest levels are found in the liver and small intestine. HFE is prominently expressed in the deep crypt cells of the duodenum. It is now clear that HFE is involved in a complex regulation of hepcidin secretion, with subsequent lack of hepcidin mRNA expression and hepcidin secretion in the face of excessive total body iron stores [9]. Evidence for the role of HFE in iron metabolism comes from various animal models. Studies in the β2 m knockout mouse have shown that these mice accumulate iron in a pattern similar to that of HH. The murine HFE homologue has been disrupted and exhibits a phenotype very similar to HH, with an elevated transferrin saturation and iron accumulation in the parenchymal cells of the liver and abnormal regulation of hepcidin. Liver-specific HFE disruption leads to a phenotype similar to a complete knockout. Both HFE and TFR2 are also necessary for induction of the bone morphogenic/SMAD pathway that induces transcription of the hepcidin gene [9]. Hepcidin transcription is also stimulated in response to inflammation and in particular the cytokine interleukin 6 [9].
Epidemiology
Hereditary hemochromatosis is one of the most common genetic diseases in the white population, with a prevalence of the C282Y homozygous state of 1 in 200–400 [10]. The disease is most common in individuals of northern European descent. The frequency of the C282Y mutation is highest in subjects from northwest Europe (10–20%), less frequent in southern and eastern European populations (2–4%), and rare in natives of Africa, Central or South America, Eastern Asia, and the Pacific Islands [11]. The H63D mutant has a distribution similar to that of C282Y, but it is more common in European groups (15–40%). In a large population-based study of 3,011 unrelated white adults in Australia, 14% were heterozygous for C282Y and 0.5% were homozygous [11]. Overall estimates are that about 6.8% of the US white population is heterozygous for C282Y and 0.5% are homozygous. This is fairly similar to the incidence estimates of 1 in 200 for iron overload in the worldwide white population [12]. Therefore, although HH is a disease with worldwide distribution, it remains predominately a disease of individuals of northern European descent. The distribution of genotypes in this population is shown in Figure 29.3 [13].
Figure 29.3 Distribution of genotypes in patients with hemochromatosis. Combination of 19 studies encompassing 1,618 patients; wt: wildtype.
Clinical Features
In adults, HH may present with the clinical syndrome of diabetes, cirrhosis, and increased skin pigmentation, as initially described in 1865. Although the genetic defect is present at birth, years of increased iron absorption and tissue accumulation (usually >5 g of excess total body iron) are required for the development of clinical symptoms (Figure 29.4). Consequently, the true prevalence of symptoms was uncertain. Since the discovery of HFE, it is recognized that many adults who are homozygous for C282Y mutation are asymptomatic [11]. The disorder is classified into four stages:
Figure 29.4 Typical progression of iron accumulation and pathology in hereditary hemochromatosis.
The four stages comprise: (1) a genetic predisposition with no abnormality other than a possible elevation of serum transferrin saturation; (2) iron overload (2–5 g) without symptoms; (3) iron overload with early symptoms (lethargy, arthralgia); and (4) iron overload with organ damage. For further data on the clinical manifestations in adults, the reader is referred to comprehensive reviews [9, 14].
Penetrance of the HH genotype is low such that disease expression depends on the mutation as well as other genetic and environmental factors, such as sex, age, dietary iron, and intestinal disease. For example, males with HH typically present earlier than females, presumably because of the ongoing iron losses in menstruating females.
As iron accumulates in the tissues, there is progressive fibrosis and injury. As organ failure results, the classic clinical consequences of hemochromatosis are recognized. The organs involved and pathologic findings are listed in Table 29.2. A recent meta-analysis of genotype-phenotype correlations in HH revealed that cardiomyopathy, hypogonadism, diabetes, and skin discoloration were less common and arthritis/ arthropathy were more common in HFE-associated HH compared to non-HFE HH (specifically HJV-associated JHH) [14].
Table 29.2 Organ Involvement in Hereditary Hemochromatosis
Organ | Histology |
---|---|
Liver | Periportal iron deposition, fibrosis, cirrhosis |
Pancreas | Fibrosis with normal exocrine and beta-cell function; abnormal beta-cell function. |
Skin | Bronzing secondary to increased melanin |
Heart | Dilated and restrictive cardiomyopathy |
Joints | Hip, shoulder, knee, metacarpophalangeal joint involvement, chondrocalcinosis |
Pituitary | Fibrosis leading to hypogonadotropic hypogonadism |
Most children and adolescents with HH are asymptomatic. Although most will have abnormal transferrin saturation, a normal ferritin is common. Reports of elevated ferritin and death from congestive heart failure in children have not been confirmed by genotype analysis and may represent JHH, a truly distinct disorder [15]. A study screening the children of 179 homozygotes found that even at a mean age of 37 years, most affected children of parents with HH were asymptomatic [16]. Several studies have shown that heterozygotes do not develop significant iron overload unless another illness is present. However, heterozygotes do have slightly higher transferrin saturations than those in normal individuals (men 38% vs. 30%; women 32% vs. 29%), suggesting that the heterozygote state may be protective for iron deficiency in women.
Diagnosis and Screening
Prior to the discovery of HFE, the diagnosis of HH required the documentation of iron overload in the liver or HLA linkage to an affected individual. Criteria for iron overload consistent with HH include (1) grade 3 or 4 stainable iron on liver biopsy; (2) hepatic iron concentration >4,500 μg/g dry weight (80 μmol/g); (3) a hepatic iron index (iron concentration in micromoles per gram liver dry weight divided by age in years) of >1.9; or (4) evidence of iron overload of >5 g. These criteria are rarely encountered in children because in the absence of other contributing factors (high dietary intake, hepatitis C viral infection, alcohol etc.), children less commonly develop significant iron overload. Indeed, these criteria are probably not acceptable for individuals identified by family screening, who may be early in the course of iron overload. Some investigators have suggested that any individual with an abnormal hepatic iron concentration (>30 μmol/g (1,500 μg/g) dry weight) who has no other reason for iron overload should be suspected of having HH. However, a variety of disorders can lead to secondary hepatic iron overload, including chronic liver diseases and hemolytic disorders (Table 29.1). There is no association with an increased prevalence of HFE mutations in either alcoholic liver disease or viral hepatitis [17]. However, in both non-alcoholic steatohepatitis and porphyria cutanea tarda, a higher frequency of the C282Y mutation has been observed [18].
In adults, the transferrin saturation is used for screening, with the threshold for further investigation being 45% in men and 42% in premenopausal women. Abnormal transferrin saturation has been reported in children as young as two years of age. However, fasting transferrin saturation and ferritin level in affected children can be normal, even in known homozygous subjects [19]. Indeed, as many as 30% of women with HH who are under 30 years of age have normal transferrin saturation [19]. Therefore, transferrin saturation is helpful in phenotypic screening in children when it is abnormal, but does not exclude HH when normal. In contrast to the utility of transferrin saturation, ferritin may be elevated in many inflammatory liver diseases such as chronic viral hepatitis and non-alcoholic steatohepatitis in the absence of HH and is less helpful in phenotypic screening for HH in children and adults.
Liver biopsy has been primarily studied in adults. Increased hepatic iron can be demonstrated by Prussian blue staining. Hepatic iron quantification with the determination of the hepatic iron index (micromoles of iron per gram dry weight liver divided by age in years) has been considered one of the more sensitive and specific tests for HH. Several studies have demonstrated that a hepatic iron index >1.9 in the absence of secondary iron overload is indicative of HH. However, 10–15% of patients with HH identified by genetic testing will have a hepatic iron index <1.9, calling into question the use of this test for diagnosis in children [20]. In children with HH, an abnormal hepatic iron index has been reported in those as young as seven years. Liver biopsy has given way to MRI quantification of hepatic iron content. Liver biopsy is now reserved for assessment of hepatic fibrosis in HH [20]. However, in the era of transient elastography to measure liver stiffness, which reflects liver fibrosis, liver biopsy in HH will likely rarely be necessary for diagnosis and management.
Genetic testing is available on a commercial basis. The distribution of genotypes in patients with HH is shown in Figure 29.3. Eighty-four percent were C282Y homozygotes and 3% were C282Y/H63D compound heterozygotes. Not all C282Y/H63D compound heterozygotes will develop the HH phenotype. None of the H63D homozygotes had an HH phenotype. A few individuals were heterozygous for one mutation and had iron overload. Heterozygosity may contribute to iron overload with an associated condition, but it should not be considered the sole cause of iron overload. Therefore, testing for HFE mutations should include the C282Y and H63D genes and only C282Y/C282Y and C282Y/H63D should be considered indicative of HH. Liver histology and quantitative liver iron or MRI may be necessary to aid in the diagnosis when iron overload is suspected yet C282Y and/or H63D mutations are not identified.
Approach to the Child with a Parent with Hereditary Hemochromatosis
The preferred clinical assessment of a child whose parent has HH is open to debate. Because symptomatic end-stage organ disease from HH is easily preventable with early therapy, screening of potentially affected children has been advocated. However, the majority of patients present with clinical disease after the age of 20 years. With the advent of genetic mutation testing, Adams et al. [16] have shown that it is cost-effective to screen the spouse of the affected parent with mutation analysis (genetic strategy, Figure 29.5). If the unaffected parent is either heterozygous or homozygous for C282Y, the potentially affected children are then screened with mutation analysis following appropriate counseling and consent [16]. Subsequently, at-risk children are followed with fasting transferrin saturation, ferritin, and liver blood tests. This strategy was found to be more cost-effective when compared with the phenotypic strategy [21]. When the serum ferritin is >200 μg/L or aminotransferases increase, consideration could be given to MRI hepatic iron quantification or direct initiation of phlebotomy therapy.
Figure 29.5 Genotypic strategy for screening for HH in a child with a parent with HH.
In the rare occurrence where a child has a parent with HH, but without C282Y/C282Y or C282Y/H63D genotypes, the screening for the HH phenotype is as follows: (1) fasting transferrin saturation and ferritin every two to three years starting at five to ten years of age, then annually at 20 years of age; (2) if the fasting transferrin saturation is >45–55% with an abnormal ferritin or either abnormal transferrin saturation or ferritin with abnormal liver tests, then obtain MRI for quantitative iron; (3) if excess iron on MRI, then initiate phlebotomy treatment.
Iron Overload in Children with Liver Dysfunction
In children, iron overload should be considered in the differential diagnosis of liver disease and testing for transferrin saturation and ferritin should be considered in the evaluation of hepatic dysfunction (Figure 29.6). If liver biopsy is performed as part of the evaluation for the underlying cause of the liver disease, staining for iron should be done and quantitative hepatic iron determination should be considered. Children with evidence of iron overload (elevated transferrin saturation of >45%, elevated ferritin, increased stainable iron or hepatic iron concentration) would be candidates for HFE mutation analysis. HFE mutation analysis also may be helpful in patients with other diseases that can lead to iron overload (non-alcoholic steatohepatitis, porphyria cutanea tarda).
The role for liver biopsy has not been established in children. Data from a large adult study have shown that 50% of patients with HH with a serum ferritin >1,000 μg/L, abnormal aspartate aminotransferase, or hepatomegaly had significant fibrosis on liver biopsy [22]. In contrast, none of the patients with HH without those factors had significant fibrosis [22]. Even before genotyping was available, it was shown that patients diagnosed in the pre-cirrhotic stage who were treated with phlebotomy had a normal life expectancy.
Because HH is a common genetic disorder whose effects can be prevented by pre-symptomatic intervention, screening of the general population has been considered. However, until the cost of testing is reduced and implications of early management are clarified, newborn testing has not been recommended [23]. In adults, screening strategies have been suggested using a combination of transferrin saturation and confirmation with HFE testing.
Treatment
The mainstay of treatment for HH is phlebotomy and the goals are to reduce total body iron overload and prevent re-accumulation of iron. When instituted before end-organ injury, successful treatment ideally prevents the development of cirrhosis and other end-organ disease and reduces the incidence of hepatocellular carcinoma. A standard adult treatment schedule is shown in Box 29.1 [2, 24]. One unit of blood contains ~200–250 mg of iron. Current recommendations are that phlebotomy is indicated if serum ferritin is >300 μg/L in males or 200 μg/L in females [25]. The goals of therapy are to maintain a ferritin level 50–100 μg/L. There are few published data on phlebotomy therapy in children, as most children with HH do not meet the preceding criteria. Initial therapy in symptomatic children should include phlebotomy of 5–8 mL/kg (maximum 500 mL) every other week until the ferritin has decreased to 50–100 μg/L. Thereafter, three to four sessions per year will probably be required. In our experience, therapeutic phlebotomy may need to be less frequent in children with HH who have asymptomatic iron overload, probably because of their lower total body iron load. Careful attention to the development of iron deficiency is required when treating children. Once identified, children with HH should be counseled to maintain a low-iron diet and avoid ascorbic acid and vitamin supplements containing iron.
Box 29.1 Standard Adult Hereditary Hemochromatosis Treatment Schedule
Remove excess iron:
Phlebotomy sessions: 1–2x/week, 500 mL/session
Check hemoglobin prior to each session
Do not allow hemoglobin to drop more than 20% of previous levels
Check iron, transferrin saturation, ferritin after every 10–12 sessions
Decrease frequency of sessions when ferritin reaches 50–100 μg/L
Avoid vitamin C supplements
Avoid iron deficiency.
Prevent re-accumulation of iron:
Phlebotomy every two to three months on average
Maintain ferritin 50–100 μg/L
Screen for hepatocellular carcinoma if cirrhotic.
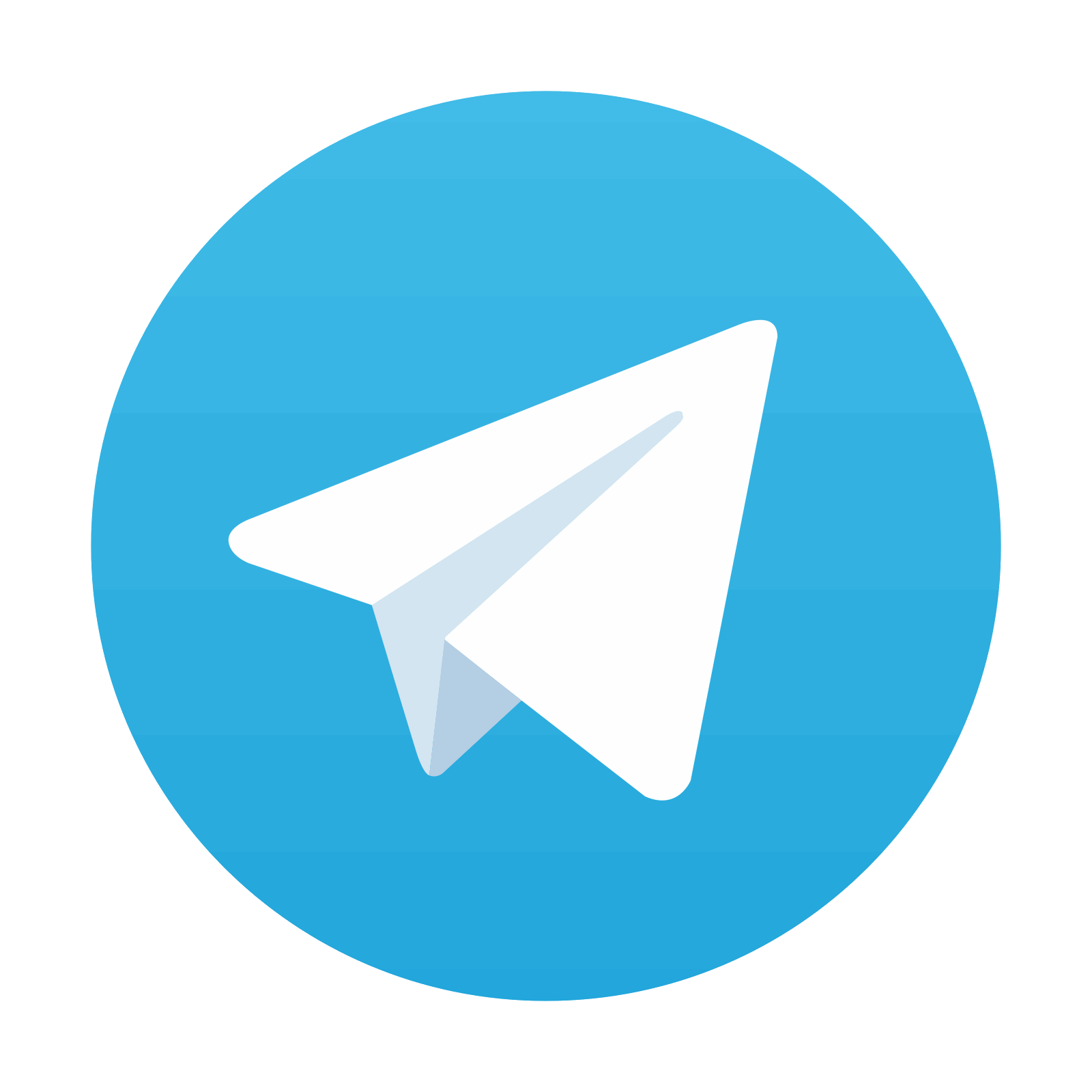
Stay updated, free articles. Join our Telegram channel

Full access? Get Clinical Tree
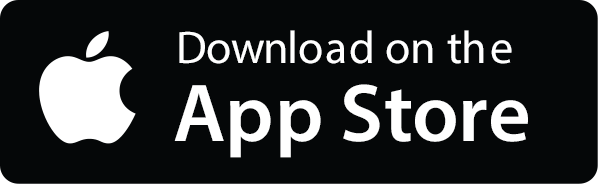
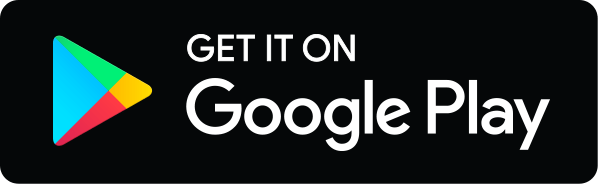
