Abstract
Drug-induced liver disease has been regarded as rare in children. Large surveys have generally failed to detect drug hepatotoxicity as a major problem in children [1], although adverse drug reactions (not necessarily hepatotoxic) are somewhat more frequent in preschool children and in children of any age with cancer. A study examining deaths from adverse drug reactions in children found that approximately one-sixth of such deaths involved acute liver failure, usually associated with antiepileptic or anti-neoplastic drugs [2].
Drug-induced liver disease has been regarded as rare in children. Large surveys have generally failed to detect drug hepatotoxicity as a major problem in children [1], although adverse drug reactions (not necessarily hepatotoxic) are somewhat more frequent in preschool children and in children of any age with cancer. A study examining deaths from adverse drug reactions in children found that approximately one-sixth of such deaths involved acute liver failure, usually associated with antiepileptic or anti-neoplastic drugs [2]. Drug hepatotoxicity is recognized as an important cause of acute liver failure in children, as in adults [3]. Why childhood drug hepatotoxicity is otherwise relatively uncommon remains unclear. Failure to diagnose drug hepatotoxicity in children is a likely explanation. Failure to report it is another. However, most children take relatively few medications. Recent reports of drugs most frequently causing clinically evident hepatotoxicity in adults show that a drug has to be in broad general use to end up a major offender. Although trends may change, children uncommonly take the cardiovascular, antihypertensive, or antidepressant medications typically associated with hepatotoxicity in adults. Despite increasing prevalence of childhood overweight/obesity, most children have a lean body mass, and most do not abuse ethanol or smoke cigarettes. Therefore, children are usually free of factors predisposing to drug hepatotoxicity in adults. Hepatic drug metabolism in children may be sufficiently different from that in adults to shield against drug hepatotoxicity. Indeed, old age is a risk factor for more severe hepatotoxic reactions, perhaps because the aging liver metabolizes some drugs more slowly. Capacity for hepatocellular regeneration may be greater in children than in adults. Nevertheless, drug hepatotoxicity definitely occurs in children; adolescents are probably no different from adults in their risk for drug-induced liver disease.
Drug-induced liver disease is challenging because it encompasses a wide spectrum of clinical disease. Cytotoxic processes, presenting as hepatitis, are most common, but almost every major type of hepatic pathology can occur. Hepatic drug metabolism plays an important role in hepatotoxicity in children. With many hepatotoxic drugs, a critical imbalance between generation of a toxic metabolite and detoxification processes can be identified. Focal defects in detoxification may be inherited. Toxic metabolites may alter cellular proteins to produce haptens that provoke an untoward immune response. The complicated, highly variable mechanics of the immune response influence the actual manifestation of drug-induced liver disease. Therefore pharmacogenetic and immunogenetic features are important mechanistically in most pediatric drug hepatotoxicity. Individual variations in cellular metabolic processes may also be important. Developmental changes in drug disposition and biotransformation further complicate drug hepatotoxicity in children. Making the diagnosis of drug hepatotoxicity depends largely on including it in the differential diagnosis. At the very least, drug-induced or environmental xenobiotic-induced hepatotoxicity should be considered when other etiologies of childhood liver disease are excluded. Children and adolescents taking medications known to be potentially hepatotoxic need close monitoring.
The recently introduced term “drug-induced liver injury” (DILI) may be preferred terminology, although “injury” proves difficult to define adequately. A detailed characterization of DILI has been formulated to serve as a basis for research relating to DILI in adults [4]. Since it is often difficult to prove causation, “drug-associated liver injury” (DALI) might be more accurate in many cases. Importantly, the discussion relating to children differs from an overview of drug hepatotoxicity/DILI in adults. For many of the more frequent drug-induced liver injuries in children, the mechanism of the hepatotoxic process has been hypothesized and elucidated. This achievement has been important for understanding drug hepatotoxicity in patients of any age. However, our appreciation of the spectrum of possible drug hepatotoxicity in children reflects the more extensive experience in adults. Readers seeking an all-inclusive discussion of drug hepatotoxicity should consult references [5, 6] or hepatotoxicity databases (such as ) focusing almost exclusively on this adult experience.
Hepatic Drug Metabolism
Drug metabolism, or biotransformation, is one of the most important functions of the liver. These complex biochemical processes can be divided into two broad aspects: activation (phase I) and detoxification (phase II). Different families of enzymes perform phase I and phase II drug metabolism. With respect to hepatotoxicity, the balance between phase I and phase II processes is critical. Factors that influence this balance include age or stage of development, state of nutrition (mainly fasting or undernutrition; possibly obesity resulting in hepatic steatosis), co-administered drugs, and immunomodulators resulting from viral infection, for example, with influenza viruses or HHV-6. Inducers (chemicals that increase the amounts of functional enzymes involved in biotransformation) may affect both phase I and phase II processes, but not necessarily equally. Co-administered medications may act as inducers or inhibitors of specific drug-metabolizing enzymes. The pharmacokinetics of the drug, particularly its absorption from the gastrointestinal tract or other organs and its mode of excretion, affects hepatic biotransformation. Whether the drug is taken as a single dose or as many doses on a chronic basis may also change its hepatic metabolism. Sporadic recurrent exposure to some drugs may enhance their toxicity. Genetically determined polymorphisms of cytochromes P450 and various phase II enzymes also influence this balance.
The hemoprotein cytochromes P450, found in most body tissues, are extremely important in the liver, notably for bile acid synthesis and hormone/drug biotransformation [7, 8]. They are associated with phase I reactions such as hydroxylation, dealkylation, and dehalogenation. The common feature is that one atom of molecular oxygen is inserted into the substrate. Hence these enzymes are monooxygenases. Unlike most enzymes, many cytochromes P450 have overlapping substrate specificity: each is not absolutely restricted to a unique substrate. Another important feature of many hepatic cytochromes P450 is inducibility. In hepatocytes various cytochromes P450 are found in the endoplasmic reticulum, mitochondria, and peroxisomes.
The cytochromes P450 are classified in families and subfamilies, which have been distinguished on the basis of primary amino acid sequence identity. With respect to human hepatic drug metabolism, cytochromes P450 in the 1A, 2B, 2C, 2D, 2E, and 3 A subfamilies (abbreviated at CYP plus subfamily name) are particularly important. The CYP1A subfamily includes two major cytochromes induced by polycyclic aromatic hydrocarbons. Apart from various carcinogens and xenobiotics, caffeine and theophylline are metabolized by these cytochromes. Induction of CYP1A1 is regulated through a cytoplasmic protein, the aromatic hydrocarbon (Ah) receptor. The member of this subfamily that is exclusively hepatic is CYP1A2: it is expressed constitutively, and its induction is also regulated by the Ah receptor. The constitutive expression of CYP1A2 shows substantial interindividual variation, but the basis for this variation is not known. The CYP2B subfamily includes cytochromes induced by phenobarbital. Some members of the CYP2B subfamily are regulated by the constitutive androstane receptor (CAR) that dimerizes with the retinoid X receptor (RXR) and then interacts with a regulatory site in the 5′-upstream region of the structural gene. CYP2E1 is the ethanol-inducible cytochrome P450, whose regulation is complex and mainly post-transcriptional. The CYP3A subfamily includes cytochromes induced by pregnenolone, glucocorticoids, rifampicin, and also phenobarbital. The most abundant cytochrome P450 in the liver is CYP3A4, which plays an important role in the biotransformation of many drugs. CYP3A4 is regulated via the pregnane X receptor (PXR), which interacts with RXR to produce the functional transcriptional regulator. Various members of the nuclear receptor family (CAR, PXR, and others such as hepatocyte nuclear factor-4α) play important roles in regulating expression of hepatocellular P450s and also in regulating expression of these very receptors. Cross-talk among receptors adds a further level of complexity.
Polymorphisms for certain cytochromes P450, relating to differences in the rate of associated enzyme activities, have been identified in humans [9–11]. The first of these polymorphisms for drug oxidation identified involves CYP2D6. Debrisoquine 4-hydroxylation, an enzyme activity associated with this cytochrome, was found to vary significantly in the Caucasian population: some individuals are “extensive metabolizers” of debrisoquine and others are “poor metabolizers” of the drug. Other drugs that are substrates for CYP2D6 show the same pattern: these include antiarrhythmic agents such as encainide, beta blockers such as metoprolol, various psychoactive agents, and codeine and dextromethorphan. The difference in metabolism between extensive and poor metabolizers appears to result from changes in the catalytic site of cytochrome CYP2D6 in poor metabolizers. Poor metabolizers are at increased risk of toxic drug concentrations, possibly relevant to ecstasy hepatotoxicity. Mephenytoin, an obsolete anticonvulsant, is notable for polymorphism in its metabolism, which is associated with the CYP2C subfamily. The specific isozyme involved is likely CYP2C18. The CYP3A subfamily (mainly CYP3A4 and CYP3A5) plays an important role in hepatic drug metabolism of such drugs as nifedipine, erythromycin, cyclosporine, and diltiazem; CYP3A5 is polymorphic and tends to be expressed at a much lower level than CYP3A4. CYP1A2 has some minor polymorphisms of uncertain functional importance. Polymorphisms affecting the function of proteins regulating P450 expression may also influence hepatic drug biotransformation. Variability in the P450 oxidoreductase, common to all P450s, might be important.
The outcome of most phase I biotransformation reactions is to make the substrate a more polar chemical with a substituent available for further modification via a phase II reaction. Phase II detoxifying reactions are performed by a variety of enzyme types, including glutathione S-transferases, UDP-glucuronosyltransferases, epoxide hydrolases, sulfotransferases, N-acetyltransferases, and enzymes responsible for glycine conjugation. These reactions complete the conversion of a hydrophobic chemical to a hydrophilic one, which can be excreted easily in urine or bile. Certain phase II enzymes, such as some glucuronosyltransferases, are inducible. Some are polymorphic. An important example of a phase II polymorphism involves N-acetylation. Arylamine N-acetyltransferase 2 (NAT-2) is polymorphic: individuals are either rapid or slow acetylators; a related enzyme, arylamine N-acetyltransferase 1, is monomorphic. These enzymes are encoded by two separate genes, and the slow acetylator phenotype of NAT-2 relates to mutations in the gene for NAT-2 causing reduced concentrations of NAT-2 in human liver. The phase II enzyme involved in metabolism of 6-mercaptopurine, thiopurine methyltransferase, is polymorphic. Certain polymorphisms are more or less prevalent in specific ethnic groups; for example, more than 50% of Caucasians are slow acetylators. The UDP-glucuronosyltransferases are diverse and include two large subfamilies that are involved in drug metabolism, UGT1 and UGT2. Genetic abnormalities in UGT1A1 are associated with Gilbert syndrome. In Gilbert syndrome drug metabolism may be affected: individuals homozygous for the common Caucasian genotype (UGT1A1* 28) have further abnormalities in the UGT1A1 gene such that its transcription and hence UGT1A1 production is decreased [12]. This lack is known to contribute to drug hepatotoxicity of certain anti-neoplastic drugs for which UGT1A1-mediated glucuronidation is an important mode of drug disposition [13] and may extend to other drugs. Additionally, glutathione S-transferases M1 and T1 are significantly polymorphic. In some metabolic diseases the activity of phase II enzymes may be abnormal. Hereditary oxoprolinemia is associated with low glutathione S-transferase activity. In hereditary tyrosinemia type 1, glutathione S-transferase activity is abnormally low on an acquired basis because intermediates in the abnormal tyrosine pathway consume glutathione.
Hepatocellular mitochondria are increasingly recognized as playing a critical role in drug hepatotoxicity [14] since mitochondria produce energy for the cell, play an important role in fat metabolism, and mediate apoptosis. Failure to generate enough ATP may eventuate in cell death. In addition to the oxidative phosphorylation chain, mitochondria retain certain enzymes which combat oxidative stress, including superoxide dismutase 2 and glutathione peroxidase 1. Polymorphisms in the genes for these enzymes may also predispose to drug hepatotoxicity [15]. Mitochondrial dysfunction may play a role in drug hepatotoxicity characterized by hepatocellular steatosis [16].
Phase I reactions can involve generation of a reactive or “toxic” metabolite. A toxic metabolite is a short-lived chemically reactive species that usually cannot be detected outside of the cell. Phase II reactions usually inactivate such chemicals before they damage the hepatocyte. Conjugation with glutathione is particularly important in detoxifying electrophilic toxic metabolites and free radicals. Whether a reactive metabolite actually damages a cell depends on how much metabolite actually binds to cellular components, whether these organelles are critical to cell survival, and whether they can be repaired. If the toxic metabolite binds to intracellular proteins or membranes that are vital to cellular integrity, the hepatocyte may die. If it binds to a cellular protein and alters its structure, it may create a neoantigen which elicits an immune response. Damage to proteins within the bile canalicular membrane typically interferes with production of bile and thus causes cholestasis. Changes in the function of P-glycoprotein, the bile salt excretory pump, MDR3 [17] and other bile canalicular transporters which may excrete drugs and drug metabolites is another mechanism of drug-induced liver injury. If the toxic metabolite binds to cellular DNA, mutagenesis or carcinogenesis may eventually ensue. In fetal tissues, binding of a toxic metabolite can also result in teratogenesis (Figure 22.1).
Hepatic drug metabolism displays complex developmental changes [18, 19]. In the newborn infant, hepatic biotransformation dependent on cytochromes P450 is typically much less active than that in adults. Decreased hepatic drug metabolism is particularly severe in premature infants. Metabolism and elimination of caffeine, theophylline, phenobarbital, and phenytoin are notably slow. In general, however, in early infancy different P450s display different developmental patterns of expression. Some (CYP2A6, CYP2C9, CYP2D6, CYP2E1, and CYP3A4) are expressed and active relatively soon after birth, but others (CYP1A2, CYP2B6, and CYP2C8) take months [20]. In childhood, hepatic drug metabolism, and thus clearance of many drugs, is more rapid than in adults. Prominent examples again include theophylline, phenobarbital, and phenytoin. By puberty, adult patterns of hepatic drug metabolism appear to be well established. Caffeine, which is metabolized in part by CYP1A2, exemplifies these changes. The elimination half-life, which is very long in the newborn period, drops to approximately three to four hours around six months of age [21]. Until puberty, caffeine metabolism remains somewhat more rapid than in adults. In extensive metabolizers, CYP2D6 rises progressively over the first five years of life, and this developmental pattern appears to be independent of gestational age [22].
Phase II biotransformation processes also display developmental changes. Among phase II processes, an important example of late maturation of a detoxifying enzyme is the glucuronosyltransferase for bilirubin conjugation, which is usually deficient for a short time after birth. Sulfation tends to predominate over glucuronidation, for example, in the metabolism of acetaminophen. Hepatic bile acid metabolism also shows maturational changes in the first months of life: in neonates, conjugation to taurine is quantitatively more important than conjugation to glycine. Bile acid sulfation is quantitatively less important than in adults. Glutathione metabolism is also subject to developmental changes, particularly in the neonatal period. Developmental changes in the proportions of various biliary glutathione-derived thiols reflect increasing bile production over this time, as do changes in γ-glutamyltranspeptidase activity and other metabolic processes related to hepatic bile production. Strong associations of hepatic injury with drugs for treating HIV infections in neonates have been reported, but it is not clear that this represents a developmental susceptibility [1].
Patterns of Drug Hepatotoxicity
Because the liver is anatomically and physiologically complex, drug hepatotoxicity presents clinically as a broad spectrum of biochemical, histologic, and clinical abnormalities. Most drug-induced liver disease is cytotoxic, and most often the hepatocyte is the target cell. The exact mechanism of hepatocyte death differs depending on the specific hepatotoxin. Hepatocyte damage may be zonal, reflecting metabolic specialization in the hepatic lobule. Hepatocytes in zone 3 of the Rappaport acinus have the highest concentration of cytochromes P450 and thus the greatest potential for producing toxic metabolites. Zonal hepatocellular necrosis suggests that production of toxic metabolites plays an important role in the pathogenesis of the hepatotoxicity. The cellular diversity of the liver also contributes to the diversity of drug-induced liver disease. Drug-induced injury may involve cells in the liver besides hepatocytes. Cytotoxic damage may predominate in bile duct cells (as with chlorpropamide), hepatic stellate cells (in vitamin A toxicity), or endothelial cells (with pyrrolizidine alkaloid poisoning from certain herbal teas). Damage to bile duct epithelial cells or to larger bile ducts is likely to interfere with bile flow, resulting in cholestasis.
Cytotoxicity may be modified by robustness, or inadequacy, of the cytoprotective processes or regenerative capacity of an individual’s hepatocytes. It can have other effects besides cell death. Non-lethal damage to certain subcellular elements may interfere with specific metabolic functions such as protein or lipid synthesis or energy production. Accumulation of fat or other substances in hepatocytes may then occur. Fatty liver associated with tetracycline hepatotoxicity is an example. Cholestasis can also result from injury to hepatocytes. Many drugs associated with cholestatic hepatotoxicity are substrates for the bile canalicular enzymes. They can interfere directly or indirectly with the action of the bile salt export pump or other transporters. Genetic variations in those enzymes may increase their susceptibility to drug-induced injury [23]. Whenever hepatocellular damage is sufficiently severe, some degree of cholestasis will develop, evident as clinical jaundice. Cytotoxicity may have more extensive consequences. Cirrhosis can develop. Vascular perfusion of the liver may be altered, as in veno-occlusive disease (VOD). Finally, hepatotoxicity may lead to neoplastic transformation. In some cases, toxic metabolites have been identified that are capable of binding to DNA, thus initiating carcinogenesis.
Drug hepatotoxicity is also classified in terms of the duration of the process. Acute hepatotoxic injuries develop over a relatively short time and cause a lesion without any histologic features of chronicity. Subacute hepatotoxicity refers to lesions that have developed over weeks to months, as indicated by areas of fibrosis and possible regeneration. Chronic hepatotoxic lesions include those with fibrosis or cirrhosis, small bile duct paucity (ductopenia), vascular changes, and neoplasia.
A practical and widely used classification of drug-induced hepatotoxicity is based on clinical features. Drug-induced liver disease most often presents as a hepatitic process, sometimes accompanied by symptoms associated rather non-specifically with hepatitis (fatigue, anorexia, nausea, or vomiting). Drug-induced hepatitis is frequently asymptomatic, with isolated elevations in serum aminotransferases. Some drug-induced liver disease is predominantly cholestatic. Clinically, there is jaundice, pruritus, prominent elevation of serum alkaline phosphatase, and mild elevations of serum aminotransferases. Cholestasis associated with contraceptive steroids is a classic example of this “bland cholestasis.” Some drug-induced liver disease presents a mixed picture, with elements of both hepatitis and cholestasis. This may be due to injury both to hepatocytes and bile duct epithelial cells, or hepatocellular injury mainly involving the bile canalicular membrane. This mixed hepatitic-cholestatic process (sometimes called hepatocanalicular jaundice) is characteristic of hepatotoxicity caused by chlorpromazine and erythromycin. This broad clinical classification has been codified in terms of biochemical features. The operative concept is the ratio of ALT to ALP. This ratio is normalized by building in the ratio of ALT and ALP to their respective upper limit of normal (ULN). Thus R = (ALT/its ULN)/(ALP/its ULN). For the hepatocellular >2 and <5 pattern, R ≥ 5; for cholestatic, R ≤ 2; for mixed hepatitic-cholestatic, R > 2 and <5 [4]. It is not entirely obvious that this characterization can be extended uncritically to the pediatric age-bracket, although the normalization is an attractive feature. A simpler categorization has been utilized for pediatric DILI [24].
In addition, these three basic clinical types (hepatitic, cholestatic, mixed hepatitic–cholestatic) may be associated with specific systemic syndromes. The “drug hypersensitivity syndrome” includes fever, inflammation of various organ systems (hepatitis, morbilliform rash or Stevens–Johnson syndrome, renal dysfunction, or myocarditis), lymphadenopathy, eosinophilia, and atypical lymphocytosis: hepatitis is the most frequent systemic manifestation, and acute liver failure can occur. (This constellation of findings is called DRESS syndrome – drug rash with eosinophilia and systemic symptoms – in the dermatologic literature, but the concept predates this acronym [25, 26].) These features indicate an immunoallergic component, but they typically accompany drug hepatotoxicity associated with production of a toxic metabolite. Eosinophilia may not be particularly striking. In some cases intercurrent viral infection may contribute to the development of the “drug hypersensitivity syndrome.” Notably, human herpes virus 6 and 7 infection, or reactivation, may sometimes play a mechanistic role. The contention that presence of a drug hypersensitivity syndrome in a child presages a favorable outcome [27] requires detailed analysis. If so, it may be due to earlier recognition of this clinical presentation and effective intervention. Notably, fatalities due to phenytoin hepatotoxicity ran at 40–50% in the 1960s to 1970s but are now estimated at 13% overall.
Another classic syndrome resembles autoimmune hepatitis (so-called “chronic active hepatitis”): best nomenclature is “AIH-like.” Findings in AIH-like drug hepatotoxicity include subacute or chronic course, fatigue, anorexia, variable extrahepatic findings (lupoid rash, arthralgias), elevated serum IgG, and detectable non-specific autoantibodies such as anti-nuclear antibody. In some cases, it may be difficult to exclude concurrence of drug administration and underlying autoimmune hepatitis. Drugs that have been associated with AIH-like hepatotoxicity include oxyphenisatin and α-methyldopa (both obsolete), nitrofurantoin and minocycline. In adults, drug-induced hepatotoxicity has been associated with certain anti-liver-kidney-microsomal (anti-LKM) antibodies, similar to those associated with autoimmune hepatitis type 2, directed against apoproteins of specific cytochromes P450. The putative reactive metabolite may bind or damage the P450 which generated it. Some P450 apoproteins can be detected on liver cell membranes.
For clinical purposes, drug-induced hepatotoxicity can be described in terms of the clinical liver disease (hepatitic, cholestatic, or hepatitic-cholestatic), with or without associated systemic syndromes (drug hypersensitivity, AIH-like) (Table 22.1), and the time course (acute, subacute, or chronic). There is no good theoretical basis for doubting that some drug-induced liver injury could be chronic. Nodular regenerative hyperplasia (NRH) and ductopenia are examples of chronic drug hepatotoxicity. Secondary sclerosing cholangitis is another example [28].
Table 22.1 Important Clinical Features of Drug-Induced Hepatotoxicity
|
|
Mixed = hepatitic-cholestatic | Hepatitis + cholestasis |
| Clinical and biochemical cholestasis |
Systemic syndrome: fever; inflammation of other organ systems (morbilliform rash or Stevens-Johnson syndrome/toxic epidermal necrolysis, renal dysfunction, myocarditis); atypical lymphocytosis; eosinophilia | |
AIH-like syndrome (mimicking autoimmune hepatitis): variable (acute→chronic) course + fatigue; anorexia; non-specific autoantibodies; increased serum IgG; variable involvement of organ systems (lupoid rash, arthralgias) |
Most drug hepatotoxicity is characterized in terms of predictability. This criterion combines mechanistic and clinical considerations. One objective is to separate chemical poisons from toxins involving host susceptibility. Intrinsic hepatotoxicity is differentiated from host idiosyncrasy. With intrinsic hepatotoxicity the agent causes predictable hepatic damage in anyone. The toxicity is dose-related, and laboratory animal models can easily be developed that exhibit the same type of hepatotoxicity. Few instances of hepatotoxicity associated with medications fit this description. Instead, most are idiosyncratic: unpredictable, infrequent, and apparently capricious. If such a reaction is accompanied by systemic features such as fever, rash, eosinophilia, atypical lymphocytosis, and possibly other major organ involvement, then classically it has been regarded as an idiosyncratic hypersensitivity reaction, with the manifest connotation of an allergic etiology.
An alternate explanation, put in its most dogmatic form, is that all instances of drug hepatotoxicity have a biochemical basis. The problem is broadly “metabolic” – mainly relating to drug metabolism. Abnormal drug biotransformation leads to increased production of toxic metabolites or inadequate provision of appropriate cytoprotective defenses or both. In some cases, such abnormalities are acquired, for example, by drug interaction. In many cases, the abnormality of drug biotransformation is genetic – an abnormal or defective drug-metabolizing enzyme – inherited as a genetic trait. This pharmacogenetic defect becomes apparent only if actuated by the appropriate drug. Without being strictly dose-dependent, higher doses of the drug may load the biotransformation pathway so that more toxic metabolite is produced. The target of the toxic metabolite determines the clinical features of the drug’s hepatotoxicity. Damage to subcellular organelles may cause cytotoxicity directly and additionally activate immune mechanisms leading to an immunoallergic response. According to this view of drug hepatotoxicity, drug hepatotoxicities whose mechanisms we understand are predictable, even if these toxicities are rare. Animal models for such hepatotoxicities can be developed but not necessarily in conventional laboratory animals, although extensive panels of inbred strains or chimeric mice with “humanized” livers may prove informative. The importance of this biochemical concept of drug hepatotoxicity is that it provides a basis for research into, and potentially for treatment of, drug hepatotoxicity. In the case of pharmacogenetic disorders of hepatic drug biotransformation, prospective diagnosis (without in vivo drug challenge) may be possible based on pharmacogenomic assessment. This concept lends itself to precision medicine. The importance for pediatric hepatology is that these definable abnormalities in hepatic drug biotransformation predominate among the drug hepatotoxicities most often found in children.
Biotransformation of drugs is not always the whole story, even though it is critical to generating toxic metabolites that alter cellular proteins. With many drugs, hepatocellular damage seems to be disproportional to the amount of toxic metabolite that might be formed. Therefore, it makes sense to look for amplifiers of the damage caused by the toxic metabolite. Both intracellular and extracellular processes may amplify the liver cell damage. This conceptual model is illustrated in Figure 22.2. As an extracellular amplifier, the immune response also plays an important role [29]. For many drugs, whether an individual develops liver injury – as well as its pattern of injury and severity – depends not only on the hepatic processes of drug metabolism but also on that individual’s immune reactivity, which is itself dependent on genetic complexion and specifics at the time of drug exposure, such as transient stimuli like endotoxinemia. Adaptive or innate immunity may be involved. A direct connection between immune-mediated mechanisms and hepatic damage occurs when autoantibodies are expressed against specific components of hepatocytes, usually those involved in drug biotransformation. The targets may be cytochromes P450 or phase II enzymes such as epoxide hydrolase. The target cytochrome P450 varies with the drug: CYP1A2 for dihydralazine, CYP2E1 for halothane, and CYP2C9 for tienilic acid. Toxic metabolites may alter other hepatocellular proteins to produce neoantigens. Immune-mediated damage to hepatocytes may involve either apoptosis or necrosis. Bile acid-associated hepatocyte injury leads to apoptosis via FAS (CD95) activation. When toxic metabolites or reactive oxygen species or cytokines stimulate Kupffer cells, specific mechanisms of cell damage are set into motion involving tumor necrosis factor-α or nitric oxide produced by Kupffer cells. Nitric oxide elaborated by Kupffer cells and hepatocytes plays a role in acetaminophen hepatotoxicity. Kupffer cells can activate natural killer (NK) and natural killer T (NKT) cells in the liver. Cytokines such as interleukin-8 [30] and other CXC chemokines regulating leukocyte action may become involved. Kupffer cells also elaborate various factors that are cytoprotective to hepatocytes [31]. The vigor of the immune response in general, an individual’s polygenic trait, most likely determines how extensively immune mechanisms contribute to drug-induced liver injury. For example, genetic polymorphisms affecting extent of cytokine production may be relevant to diclofenac hepatotoxicity [32]. Specific HLA genotypes are associated with certain drug hepatotoxicities, thus involving the adaptive immune system. Some components of the innate immune system, such as interleukin-10 and certain prostaglandins, are hepatoprotective. Thus, in addition to pharmacogenetics, immunogenetics must be considered to explain drug hepatotoxicity. There are several junctures (detection of hepatocellular stress; reaction to neoantigens; generation of cellular danger signals, mobilization of innate and adaptive immune systems) where individual differences in immune response may influence the features of drug hepatotoxicity.
Figure 22.2 A general mechanism of severe drug hepatotoxicity. Most drugs and xenobiotics that cause liver injury elaborate a toxic metabolite within the hepatocyte or nonparenchymal liver cell. The quantity of toxic metabolite is dependent on multiple factors including features of phase I and phase II biotransformation and the balance between them. The toxic metabolite can damage the cell itself or initiate various amplifying processes within the cell or external to the cell, such as immune response. Direct toxins typically injure the liver without metabolism, but in principle amplification pathways could also be involved.
Moreover, given the impressive extent of the human metabolome, it makes sense to anticipate that “metabogenetic” factors will influence individual patterns of drug hepatotoxicity; however, this dimension has thus far received comparatively little attention. Developments in the field of pharmacometabolomics will likely advance detailed concepts of drug hepatotoxicity, capable of taking into account growth and developmental changes typical of childhood [33]. While the gut microbiota influence hepatic drug metabolism [34], their role in drug hepatotoxicity requires further investigation. The relationship between disordered hepatic metabolism presenting as non-alcoholic fatty liver disease and drug hepatotoxicity also requires further investigation, notably in children [35].
Classification of chemicals that cause liver injury has to account for inevitable toxicity or for idiosyncrasy, whether from biochemical toxicity or an immune process, or some combination of the two (Table 22.2). Hepatotoxic agents can be categorized as follows: intrinsic hepatotoxin, contingent hepatotoxin, and hepatotoxin eliciting an immunoallergic response. The intrinsic hepatotoxin is the true, predictable poison. Environmental xenobiotics usually belong in this category. The contingent hepatotoxin causes hepatotoxicity only when hepatic biotransformation is abnormal so that toxic metabolites are more likely to be generated or detoxification pathways are deficient. Hepatic biotransformation may be abnormal on an acquired or pharmacogenetic basis. This category encompasses the category denoted as “metabolic idiosyncrasy” by others. A hepatotoxin eliciting an immunoallergic response is identified when hepatotoxicity is accompanied by fever, eosinophilia, and atypical lymphocytosis or is characterized histologically by hepatic granulomatosis. Elaboration of autoantibodies, such as anti-LKM antibodies, or a fully developed AIH-like pattern is another type of immunoallergic reaction. These findings imply some dependence on the host immune response. Importantly, the above categories are not mutually exclusive. Some chemicals can act as more than one type of hepatotoxin. For example, high-dose acetaminophen acts as an intrinsic hepatotoxin. By contrast, low-dose acetaminophen, normally nontoxic, acts as a contingent hepatotoxin in persons in whom CYP2E1 is induced, as in chronic alcoholics. If the biochemical mechanism of hepatic biotransformation is established for a given chemical, then it is possible to predict circumstances in which that chemical would function as a contingent hepatotoxin. Currently, it is often impossible to identify abnormal hepatic biotransformation due to a pharmacogenetic defect until after drug-induced hepatotoxicity has occurred. For the affected individual, the hepatotoxicity appears to be a chance aberration.
Table 22.2 Classification of Hepatotoxins
Nomenclature Herein | Previous or Alternate Usage |
---|---|
Intrinsic hepatotoxin | Intrinsic toxin |
Contingent hepatotoxin (toxic only if biotransformation* is abnormal, because of pharmacogenetics or acquired cause) | Metabolic idiosyncrasy; “idiosyncratic” |
Hepatotoxin eliciting immunoallergic response† (implies some degree of dependence on host immunogenetics) | Drug hypersensitivity |
* Generation and/or detoxification of toxic metabolite.
† Fever, eosinophilia, atypical lymphocytosis; or nonspecific autoantibodies; or “AIH-like”; or hepatic granulomatosis.
The clinical presentations and liver pathology of drug hepatotoxicity are extremely diverse. Drug-induced liver disease encompasses the entire spectrum of liver disease. Selected drugs and environmental toxins illustrate this broad range of drug hepatotoxicity affecting adults or children (Table 22.3). Classes of drugs which predominate in causing hepatotoxicity in children, apart from acetaminophen, are antimicrobials, antiepileptics, psychoactive drugs, treatments for attention deficit disorder, and antineoplastic agents. Of note, antineoplastic agents are underrepresented in surveys of pediatric drug hepatotoxicity [1, 36]. Since drug hepatotoxicity in children is underdiagnosed and/or underreported, quantitative epidemiological approaches are of limited utility: series are often absolutely or relatively small. Prominent “problem” drugs in children are well known: acetaminophen, valproic acid, and phenytoin. In pediatric practice, even one or two cases of drug hepatotoxicity may be informative, if only as proof-of-principle. Drugs selected for detailed commentary here include some already encountered in clinical pediatric practice and some which may prove important in the pediatric age-bracket in the future. Since drug hepatotoxicity reflects drug utilization, where possible, herbal remedies and drugs for specific pediatric treatment needs are included.
Table 22.3 Range of Clinical and Pathologic Findings in Hepatotoxicity from Drugs or Environmental Toxins
Finding | Typical Causative Agents |
---|---|
Acute hepatitis | Isoniazid, halothane |
Zonal liver cell necrosis | Acetaminophen |
Hepatitis-cholestasis | Erythromycin, chlorpromazine, azathioprine, nitrofurantoin |
Bland cholestasis | Estrogens/oral contraceptive pill, cyclosporine, haloperidol |
Steatonecrosis (mimicking alcoholic hepatitis) | Perhexiline, amiodarone |
Phospholipidosis | Amiodarone |
Microvesicular steatosis | Valproic acid, tetracycline |
Macrovesicular steatosis | L-asparaginase |
Macrovesicular fat plus fibrosis | Methotrexate |
AIH-like (mimicking autoimmune hepatitis) | Minocycline, nitrofurantoin, α-methyldopa |
Granulomatosis | Sulfonamides, phenylbutazone, carbamazepine |
Biliary cirrhosis | Practolol, chlorpropamide |
Sclerosing cholangitis | Floxuridine (administered via hepatic artery), infliximab, sevoflurane |
Gallstones | Ceftriaxone, dipyridamole |
Peliosis | Estrogens, androgens |
Hepatic vein thrombosis | Estrogens/oral contraceptive pill |
Veno-occlusive disease | Pyrrolizidine alkaloids (”bush tea”), 6-thioguanine, busulfan, oxaliplatin |
Nodular regenerative hyperplasia | Azathioprine, didanosine, 6-thioguanine |
Noncirrhotic portal hypertension | Arsenic, vinyl chloride |
Liver cell adenoma | Estrogens/oral contraceptive pill, anabolic steroids |
Malignant tumors (hepatocellular carcinoma, angiosarcoma) | Estrogens/oral contraceptive pill, anabolic steroids, vinyl chloride, arsenic |
Porphyria | 2,3,7,8-Tetrachlorodibenzo-p-dioxin, chloroquine |
Acetaminophen
Acetaminophen is an effective antipyretic and analgesic. Taken in a single large dose, however, it is a potent hepatotoxin. The clinical course of this acute acetaminophen toxicity is distinctive. Immediately after the drug is taken, nausea and vomiting occur. These symptoms subside and then there is an asymptomatic interval before liver injury becomes clinically apparent. At that point, jaundice, abnormal serum aminotransferases, and coagulopathy develop. Finally, liver failure may supervene with progressive coma. Serum aminotransferases may be extremely high in this condition, and the degree of abnormality is not necessarily predictive of outcome. In a large retrospective series of children (mainly girls) with acetaminophen overdose, prothrombin time >100 seconds (INR >7), hypoglycemia (<2.6 mmol/L), serum creatinine >200 μmol/L, acidosis (pH<7.3) at any time and progressive encephalopathy (grade 3 or 4) predicted bad outcome (death or need for liver transplant) [37]. These prognostic factors are similar to those in adults [38]. Late presentation is always problematic.
Treatment of acute acetaminophen hepatotoxicity involves the use of N-acetylcysteine as an antidote. Whether to use N-acetylcysteine can be decided on the basis of the Rumack nomogram, where the patient’s plasma acetaminophen concentration is plotted against time on a semilogarithmic graph [39]; if it falls in the zone for probable (or possible) hepatic toxicity, N-acetylcysteine should be given. N-Acetylcysteine is most effective if given within ten hours of acetaminophen ingestion, and it may be less beneficial given >24 hours after ingestion of the acetaminophen. However, even if there is doubt as to its usefulness, it should be given anyway. Late administration of N-acetylcysteine has been associated with greater survival in adults with acute acetaminophen intoxication; no adverse side effects of the N-acetylcysteine were observed [40, 41]. A 72-hour regimen of oral N-acetylcysteine appears to be as effective as the 20-hour intravenous regimen; the oral regimen may be more effective if treatment is delayed [39, 42]. The dose of N-acetylcysteine must be appropriate for body weight because an inappropriately high dose may be toxic, causing respiratory compromise or hypotension. Oral charcoal seems to have a limited role: it may provide additional benefit if administered very early, that is, within one hour of ingestion. Acetaminophen ingestion itself typically causes vomiting. Hemodialysis may be used early if plasma concentrations of acetaminophen are extremely high [43]: otherwise it is not effective. Treatment in adolescents, whose metabolism of acetaminophen resembles that of adults, should be aggressive; however, younger children also require N-acetylcysteine and supportive treatment, even when the timing and total amount of acetaminophen taken are uncertain. Liver transplant may be required for those children in liver failure who show no improvement despite full supportive treatment. Recent experience suggests that the prognosis is good in a child if after 48 hours of treatment with N-acetylcysteine the prothrombin time and serum aminotransferases are all normal [44].
In addition to this acute type of hepatotoxicity, which is encountered in toddlers invading the medicine cabinet and in suicidal teenagers, acetaminophen hepatotoxicity in children can present more subtly, as therapeutic misadventure. This occurs through various sorts of unintentional error: actual dosing error through misunderstanding the dose or using the wrong measuring device, substitution of one formulation for another, failure to appreciate how often acetaminophen turns up in various over-the-counter medications, general belief that acetaminophen is “safe” for children. In typical cases, rather large doses of acetaminophen (approximately 30–70 mg/kg, less in small infants) are administered at regular intervals (usually every two to four hours) for two to three days, or longer, before hepatotoxicity becomes evident. This is sometimes described as “chronic” overdose, but the actual time frame is comparatively short (days, not months). The liver disease presents as acute liver failure, and the systemic signs of toxicity and the asymptomatic period do not occur. Perhaps they are not prominent. Serum concentrations of acetaminophen are frequently not in a toxic range. Diagnosis is difficult unless a very meticulous drug history is taken to determine exactly what preparation of acetaminophen was used and how often. Getting the actual drug containers, even if they were discarded, may be critically important. The acute liver failure is frequently attributed to another etiology, usually acute viral hepatitis or it may be “nondescript.” Since its first description in this chapter, numerous cases of this type of acetaminophen hepatotoxicity have been documented [45–48]. The threshold for liver injury is highly variable from child to child. A suggested threshold on the order of 90 mg/kg/day for more than one day of drug administration is controversial because it impinges on the standard dosage of 15 mg/kg administered every four hours around the clock. Indeed some caution about this routine dose is appropriate: taking the “safe” dose of acetaminophen daily can cause asymptomatic elevations of ALT in adults [49] and has occurred in children [50]. In another infant the “safe” dosage schedule was exceeded [51]. Thus for children a more conservative maximum total daily dose of 75 mg/kg/day is advised. The Rumack nomogram for treatment with N-acetylcysteine does not apply in the situation of an infant or young child with high-dose chronic dosing. Importantly, finding a measurable serum concentration of acetaminophen 24 hours or more after the last dose should suggest the possibility of acetaminophen hepatotoxicity. The elimination half-life can be estimated from two drug levels obtained at a reasonable interval apart: if it is greater than four hours, hepatotoxicity is likely. Detecting an acetaminophen protein adduct, 3-(cystein-5-yl)-acetaminophen, formed by the binding of the reactive metabolite N-acetyl-p-benzoquinoneimine (NAPQI) to glutathione, may be informative [52]. Additional biomarkers are being developed [53, 54]. In general, it seems prudent to treat these patients with N-acetylcysteine as soon as possible. Anorexia and food avoidance that may have accompanied the underlying illness for which acetaminophen was used may exacerbate the hepatotoxicity by causing acute depletion of glutathione stores. These children tend to present for medical assessment late in the disease course, and this may be an important reason for the poor prognosis.
The primary mechanism for acetaminophen hepatotoxicity involves the formation of a toxic metabolite as reported by Mitchell and co-workers in a series of classic papers in the early 1970s. The important role of drug metabolism in this hepatotoxicity is reflected in the predominance of hepatocellular injury in Rappaport zone 3. Acetaminophen is usually metabolized via sulfation and glucuronidation (Figure 22.3). If a very large amount is taken, these pathways are saturated, and an otherwise minor pathway through cytochromes P450, including CYP2E1, CYP1A2, and CYP3A4, becomes quantitatively important. The product of this pathway is a highly reactive species, NAPQI, a potent electrophile. It is conjugated by glutathione, as long as sufficient glutathione is available, to form mercapturic acid, which is excreted in the urine. Otherwise NAPQI reacts with cellular proteins, causing cell damage and cell death. Intracellular processes amplifying cellular damage contribute to the liver injury, which may seem to be disproportionate to the amount of toxic metabolite produced. It appears that NAPQI initiates oxidative stress caused by reactive-oxygen and reactive-nitrogen species. This entails mitochondrial damage, apparently down-regulating the oxidative-phosphorylation chain, that itself becomes self-perpetuating and results in failure to produce ATP [55, 56]. An intracellular antioxidant response mediated by the nuclear transcription factor NF-E2-related factor (Nrf2) is also activated [57]. Apart from glutathione, other cytoprotective metabolic pathways dependent on diet may be important [58]. As part of an extracellular enhancement mechanism, various cytokines can increase or decrease the liver injury. CD44 plays an important role in the hepatotoxic mechanism: subnormal expression of CD44 may tip the balance toward increased pro-inflammatory cytokine signaling [59]. Polymorphonuclear leukocytes do not appear to enhance the injury, perhaps in part because of less migration due to decreased CD44 signal. Recent work suggests that the protein High Mobility Group Box 1 (HMGB1) plays an important role in coordinating the inflammatory response [60]. Interleukin-10 may act as an immunological cytoprotective factor interfering with nitric oxide production [61]. Dendritic cells may also play a protective role [62]. N-acetylcysteine can minimize hepatotoxicity if given early enough, but it does not reverse the injurious effects of the toxic metabolite once they have occurred. N-acetylcysteine may also promote hepatocellular recovery by enhancing oxygen delivery to the liver tissue.
Figure 22.3 Hepatic metabolism of acetaminophen.
In summary, intracellular amplification mechanisms include mitochondrial damage downregulating the oxidative phosphorylation chain and inhibiting ATP production, as well as generation of reactive oxygen and reactive nitrogen species. Extracellular amplification mechanisms include action of pro-inflammatory cytokines, possibly enhanced by subnormal expression of CD44.
Young children appear to be resistant to hepatotoxicity due to acute acetaminophen overdose and tend to recover when it does occur [63]. The incidence of hepatotoxicity was 5.5% in a study of 417 children aged five years or younger, compared with 29% in adolescents and adults at comparable toxic blood levels [64]. Various studies of acetaminophen pharmacology and toxicity in children suggest a biochemical basis for this difference. The elimination half-life is essentially the same in children and adults, although with interindividual variation, it ranges as much as 1–3.5 hours [65]. The elimination half-life is somewhat longer (2.2–5.0 hours) in neonates. The profile of metabolites differs greatly in early childhood from adolescence and adulthood: sulfation predominates over glucuronidation. The switch to the adult pattern seems to occur around 12 years of age. However, even in newborns, urinary metabolites reflecting cytochrome P450-generated intermediates can be found; thus, the capacity for producing toxic metabolites seems to be present from an extremely early age. In vitro studies with fetal human hepatocytes have shown that the cytochrome P450-generated intermediates can be formed and conjugated to glutathione as early as at 18 weeks of gestation, but the rate of formation is approximately 10% of that in adult human hepatocytes; sulfation, but not glucuronidation, of acetaminophen also can be detected in the human fetal liver cells. Human infants may also have a greater capacity for synthesis of glutathione than adults and thus can produce enough new glutathione to inactivate toxic metabolites of acetaminophen more effectively.
Despite this relative resistance to this type of hepatotoxicity, very young children can develop severe hepatotoxicity from acetaminophen. Some of these reports represent acute poisoning [66]. Therapeutic misadventure due to inappropriate dosing is strikingly more frequent in this age group: children <3 years old predominate. Some of this acetaminophen-associated hepatotoxicity might be avoided by clear instructions to parents about dosing and use of conservative dosage guidelines.
An emerging pediatric focus is acetaminophen in neonates, due to its important role for pain management. Hepatotoxicity and extreme prolongation of the elimination half-life of acetaminophen have been found in infants born after maternal self-poisoning with acetaminophen. Studies of acetaminophen pharmacokinetics in neonates show predominance of sulfation in its metabolism, substantial interindividual variation, and body weight as an important determinant [67, 68]. Data regarding preterm infants are sparse: one study showed extensive sulfation of acetaminophen but did not address potential toxicity in any detail [69]. Hepatotoxicity has been reported after oral [70, 71] and intravenous administration of acetaminophen; equally, good tolerability has been reported. The combination of variable susceptibility to hepatotoxicity, potentially disastrous outcome, and insufficient pharmacological data mandates a cautious approach to treating neonates with acetaminophen. Results in one study supported prudent dose reduction if the infant has unconjugated hyperbilirubinemia [72].
Some children may have innate defects in acetaminophen detoxification, but this has been difficult to pinpoint mechanistically. Children with 5-oxoprolinuria, who cannot produce glutathione efficiently, are at increased risk of liver injury due to acetaminophen. The possibility that patients with Crigler-Najjar syndrome or Gilbert syndrome are more susceptible to acetaminophen hepatotoxicity is a complex pharmacologic question based on data from the Gunn rat: it requires further investigation. Whether drugs such as zidovudine, phenytoin and phenobarbital increase acetaminophen hepatotoxicity is disputed [73]. Mercury poisoning through exposure to elemental mercury apparently enhanced acetaminophen hepatotoxicity in one child; however, mercury is itself hepatotoxic [74]. The experience in adults of chronic alcohol abuse with consequent induction of CYP2E1 may be relevant to acetaminophen hepatotoxicity in some adolescents [75]. The sum-effect of drug interactions over the entire range of acetaminophen hepatotoxicity is likely complex and highly individualistic. Attention to these modifiers may be important in the pediatric age-bracket.
The implications for liver injury of new formulations of acetaminophen, such as sustained-release tablets, remain uncertain. Confusing the sustained-release tablet dosage schedule (every eight hours) with the conventional dosage schedule (every four to six hours) might result in hepatotoxicity. Combining acetaminophen with a potentially habituating analgesic might result in excessive chronic use of acetaminophen, including at 375 mg per tablet. The plethora of over-the-counter medications that contain acetaminophen increases risk of liver injury. Acetaminophen rectal suppositories are used in children, and these pose unique problems of drug absorption and bioavailability depending on the composition of suppository matrix. Severe liver damage in a child was reported attributed to high-dose acetaminophen exposure with rectal suppositories [76]. Although several other potentially hepatotoxic drugs were also administered, this attribution is credible. Oro-dispersible tablets (which melt in the mouth) are designed for children’s use and may increase the risk of childhood hepatotoxicity [77]. Some brightly colored tablets may look like candy to a child.
Recently an intravenous preparation of acetaminophen has become available. Although it is restricted to the hospital setting and approved only for children at least two years old, reports of hepatotoxicity in children and infants have involved dosage errors. Intravenous acetaminophen has different pharmacokinetics from oral acetaminophen. The Rumack nomogram can apply but requires meticulous data [78]. It is essential to get a blood level at four hours after the dose and to note the exact time the blood sample was drawn. It is reasonable to regard a dose of 150 mg/kg or more as potentially hepatotoxic: N-acetylcysteine treatment would be appropriate. Chronically undernourished children and those acutely refusing to eat may be at special risk [79]. Management of affected infants is particularly complex. Where IV acetaminophen is available for administration to infants, a reduced dose for infants <10 kg in weight is recommended, specifically 7.5 mg/kg [79].
Amiodarone
Amiodarone is an iodinated benzofuran derivative used for the treatment of cardiac arrhythmias. Although reserved for more severe disease, it is used from time to time in children. Asymptomatic elevations of serum aminotransferases may occur. Phospholipidosis is associated with progressive liver damage in some patients. Although iodine accumulation in the liver may produce striking hepatic parenchymal density on computerized tomography (Figure 22.4), it is not in itself a sign of hepatotoxicity.
With oral administration, amiodarone-induced hepatotoxicity, characterized by hepatomegaly and abnormal aminotransferases, may develop within a month of treatment or after one year of treatment. Asymptomatic elevations in aminotransferases are frequent, occurring in one-quarter to one-half of patients treated; these abnormalities may return to normal spontaneously, even if the drug is not discontinued. Alternatively, progressive chronic liver disease occurs in some patients. Severe amiodarone hepatotoxicity has been reported in a child [80]. It presented as rapidly progressive hepatic failure beginning after two months of treatment at a relatively high dose of amiodarone (9 mg/kg/day): clinical presentation was described as Reye-like because of vomiting and progressive stupor but no jaundice.
The mechanism of amiodarone hepatotoxicity remains undetermined, but it may involve abnormal hepatic biotransformation. Amiodarone may also interfere with mitochondrial β-oxidation and oxidative phosphorylation. Acute hepatotoxicity with intravenous administration has been reported in adults, possibly ameliorated by prompt administration of N-acetylcysteine.
Anti-Neoplastic Agents
Many drugs used to treat neoplasia can cause hepatotoxicity [81]. Potential for liver damage in children may vary from experience in adults. Moreover, these drugs are rarely used separately: patients receiving them are usually at risk for multiple types of liver injury. A hepatitic pattern, often asymptomatic with elevation in serum aminotransferases and no other evidence of severe liver toxicity, is common. Anti-neoplastic agents, which frequently produce this reaction, include nitrosoureas, 6-mercaptopurine, cytosine arabinoside, cisplatin, and dacarbazine. With cisplatin the mechanism of liver injury appears to involve oxidative stress [82, 83]. For both cisplatin and dacarbazine, induction of CYP2E1 might enhance the risk of liver damage. Thrombocytopenia and acute liver failure were reported in an 18-year-old patient receiving carboplatin [84]. Cyclophosphamide may cause a dose-related drug hepatitis [85]. Carmustine and 6-mercaptopurine can also cause severe cholestasis [86]. Adriamycin and vinca alkaloids are infrequently associated with hepatotoxicity. Adriamycin given together with 6-mercaptopurine may increase the hepatotoxic potential of 6-mercaptopurine.
Dactinomycin is reputed as an infrequent cause of liver injury. However, several patients treated with dactinomycin at the Hospital for Sick Children, Toronto, have developed severe hepatic dysfunction, with extremely elevated serum aminotransferases and coagulopathy, all of which resolved spontaneously when off the drug. Similar experience has been reported in treatment of Wilms tumor [87]. Irradiation may enhance hepatotoxicity of dactinomycin. With respect to microvascular injury, children may be more susceptible to this hepatotoxicity than adults [88].
L-asparaginase is associated with severe hepatic injury characterized by severe steatosis, hepatocellular necrosis, and fibrosis, which is usually reversible after the L-asparaginase is stopped [89–91]. Severe microvesicular steatosis has been reported. The most likely mechanism for this hepatotoxicity is a profound interference with hepatocellular protein metabolism. Hepatotoxicity has been reported with pegasparase, the pegylated form of L-asparaginase [36]. Steatosis or portal fibrosis has been found on liver biopsies from children with acute lymphoblastic leukemia, treated with various anticancer drugs [92]. Recent observations give the impression that acute lymphoblastic leukemia is often complicated by hepatotoxicity. This is mainly associated with 6-mercaptopurine. Hepatic accumulation of 6-mercaptopurine metabolites was postulated in four children who developed hepatotoxicity from 6-mercaptopurine during treatment for acute lymphoblastic leukemia; one child had severe cholestatic hepatitis [86]. Chronic administration of 6-thioguanine as maintenance treatment for acute lymphoblastic leukemia in children has been associated with mild reversible microvascular injury presenting as isolated thrombocytopenia [93] or non-cirrhotic portal hypertension [94]. A long-term follow-up study of children with acute 6-thioguanine hepatotoxicity revealed that seven of ten developed clinically significant portal hypertension; however, the nature of the underlying chronic lesion was not determined [95]. 6-Thioguanine has been found to cause nodular regenerative hyperplasia in patients with inflammatory bowel disease, and in one patient veno-occlusive disease (VOD) was also present [96].
Toxic microvascular injury, mainly reported as VOD, is an important pattern of hepatotoxicity associated with anti-neoplastic drugs. An alternate term to VOD, sinusoidal obstruction syndrome, draws attention to the role of damage to sinusoidal endothelial cells in its etiology [97]. Acute presentation of VOD is with an enlarged tender liver, ascites or unexplained weight gain, and jaundice; serum aminotransferases may be elevated. In surviving patients, the liver disease may progress to cirrhosis with hepatic venular sclerosis and sinusoidal fibrosis. Patients with a more subacute course may show splenomegaly and thrombocytopenia. Although 6-thioguanine is a classic cause of VOD, other antineoplastic drugs such as cytosine arabinoside, busulfan, dacarbazine, carmustine, dactinomycin, and oxaliplatin have been associated with VOD at conventional or high doses, and drug interactions may enhance their propensity for causing this type of liver injury [98]. Currently, VOD most frequently develops after allogeneic bone marrow (stem cell) transplantation [99]; however, it has been reported with other regimens as treatment for childhood solid tumors [100, 101]. It has been disputed whether VOD is a consequence of chemotherapeutic conditioning regimens or part of the spectrum of liver injury due to graft-versus-host disease (GVHD), and in some individual patients it may, in fact, be difficult to make this distinction. In some cases, cholestasis or cytokines released as part of GVHD might contribute to the development of VOD or increase its severity. Irradiation by itself can lead to VOD possibly because endothelial cells lining hepatic sinusoids are more sensitive to radiation than hepatocytes. The combination of irradiation and chemotherapy in conditioning regimens appears to accelerate development of VOD compared to the effect of a single injurious agent (irradiation or chemical) [102]. Methotrexate plus cyclosporine (used as prophylaxis for graft-versus-host disease) in patients prepared for bone marrow transplant by a regimen using busulfan and cyclophosphamide led to a higher incidence of jaundice and VOD disease than methylprednisolone and cyclosporine prophylaxis in similarly prepared patients [103]. In general, most antineoplastic drugs carry some risk of provoking VOD.
Clinical predictors of likelihood for development of VOD in children have not yet been identified; however, ongoing hepatitis, such as chronic viral hepatitis, before transplant increases susceptibility to hepatic damage [104]. Development of multiorgan failure presages poor survival. Polymorphisms in glutathione S-transferases appear to correlate with increased risk [105]; risk associated with other phase II enzymes is less established [93]. In many patients, the process resolves, but in a sizable proportion, the process is fatal or leads to chronic liver damage [106, 107]. Treatment has generally been aimed at interfering with thrombosis, such as defibrotide [108] or other agents [109], but given the disease mechanism, early treatment with glutathione replacement might be appropriate. Anecdotal evidence suggests its efficacy [110, 111].
The pathogenesis of VOD involves primary injury to sinusoidal endothelial cells. There is secondary subendothelial hemorrhage, hepatocellular necrosis, and hepatic vein obliteration. Liver injury progresses from congestion in the sinusoids to parenchymal extinction. With dacarbazine, VOD involves damage to sinusoidal endothelial cells by toxic metabolite(s) produced in the endothelial cells; glutathione appears to protect against toxicity [112]. In contrast, in VOD caused by cyclophosphamide the toxic metabolites are produced in hepatocytes [113]. In a rat model in which monocrotaline was the toxic chemical, glutathione depletion and decreased hepatic production of nitric oxide contribute to the disease mechanism [114]. Among cytokines that may play a role in the disease mechanism, vascular endothelial growth factor has been shown to be elevated in children who develop severe VOD [115].
Although not yet reported in children, immune checkpoint inhibitors, such as ipilimumab and tremelimumab which are both active against CTLA-4, have been associated with hepatotoxicity in adults [116]. The histological appearance may resemble autoimmune hepatitis.
Aspirin
Hepatotoxicity has been associated with high-dose aspirin treatment. The hepatotoxicity appears to be dose-dependent, and patients without rheumatoid disease can develop hepatotoxicity. However, most cases are reported in patients with rheumatoid diseases. Approximately 60% of the 300 reported cases have been in patients with juvenile rheumatoid arthritis (not necessarily all children), and a further 10% have occurred in children with acute rheumatic fever. A prospective study of aspirin hepatotoxicity in adult patients with rheumatoid arthritis or osteoarthritis revealed that 5% of those taking aspirin developed asymptomatic elevations of serum aspartate aminotransferase. The preponderance of cases in patients with rheumatologic diseases, however, raises the possibility that these patients are predisposed to this toxicity. A single case of apparent hepatotoxicity associated with low-dose aspirin therapy in a young child after liver transplant has been reported; there were essentially asymptomatic elevations of serum aminotransferases, and liver biopsy revealed zonal, but periportal, hepatocellular necrosis [117].
In most cases, salicylate hepatotoxicity has hepatitic features with anorexia, nausea, vomiting, abdominal pain, and elevated serum aminotransferases [118]. The liver is usually enlarged, and it may be tender. Progressive signs of liver damage such as jaundice and coagulopathy are rare. Even in uncomplicated cases, serum aminotransferase levels may be >1,000 IU/L. In some cases encephalopathy (not related to Reye syndrome) has been present. Clinical and laboratory abnormalities resolve when aspirin is stopped. Liver histology typically shows a nonspecific picture with acute, focal hepatocellular necrosis.
Reye syndrome may be considered a type of aspirin-associated hepatotoxicity, although the mechanistic connection has never been fully proved, and its incidence has dropped precipitously as treatment of febrile illnesses with aspirin has been abandoned. A two-year-old developed fatal acute liver failure after receiving acetaminophen and willow tree bark as treatment for an upper respiratory infection: findings suggested a synergy between acetaminophen and ASA (component of willow tree bark) with features suggestive of a Reye-like syndrome [119].
Given its dose-dependency, aspirin may be an intrinsic toxin. Additionally, given the role of viral infection in some patients and disordered immune function in others and Gilbert syndrome [120], it may also be classified as a contingent hepatotoxin. Aspirin appears to inhibit mitochondrial function.
Atomoxetine
Atomoxetine is a possible treatment for attention deficit disorder in children. It undergoes extensive hepatic biotransformation, mainly by CYP2D6 but also by other cytochromes P450, notably when the “poor metabolizer” polymorphism is present [121]. Reversible elevation of serum aminotransferases was sometimes found. Severe liver injury with features of hepatitis has occurred in adults and children [122], including acute liver failure in a child [123]. An AIH-like pattern of injury has been reported [124]. The hepatotoxic mechanism is not known, but it appears to involve metabolic idiosyncrasy. “Poor metabolizers” in the CYP2D6 polymorphism may be at increased risk for hepatotoxicity, but given the complex individual variation in atomoxetine biotransformation, this is not entirely clear [125]. Atomoxetine is a contingent hepatotoxin capable of eliciting an immunoallergic response.
Azathioprine
Azathioprine is a potent immunosuppressive drug that consists of 6-mercaptopurine linked to an imidazole side-chain. In effect, it is a prodrug for 6-mercaptopurine. Since its introduction in the 1960s, azathioprine has been associated with hepatotoxicity, including in children, but these early studies are confounded by underdiagnosed concomitant viral liver disease. In adults, azathioprine hepatotoxicity has been characterized mainly by cholestasis or a hepatitic-cholestatic picture. Liver biopsy in one case showed centrilobular ballooning of hepatocytes and canalicular cholestasis [126]. Azathioprine hepatotoxicity has been described in orthotopic liver transplant recipients: endothelial cell damage, as well as hepatocyte damage and cholestasis, was noted [127]. In addition, several cases of nodular regenerative hyperplasia associated with azathioprine have been reported [128]. Nodular regenerative hyperplasia is more common than previously appreciated and is an important pattern of injury with azathioprine. Chronic use has been associated with cirrhosis in some cases. Recent evaluation of 22 cases, including at least one child, showed that azathioprine or 6-cercaptopurine tended to a hepatitic illness within three months of treatment initiation or dose escalation; in some cases the disorder was a cholestatic hepatitis [129]. Polymorphisms affecting thiopurine methyltransferase are associated with myelosuppression and may predispose to hepatotoxicity. In East Asian populations the NUDT15 polymorphism predisposes to intolerance including hepatotoxicity [130, 131].
6-Mercaptopurine has been associated more directly with liver toxicity and causes a mixed hepatitic-cholestatic reaction [132]. High concentration of the 6-mercaptopurine metabolite 6-methylmercaptopurine nucleotide predicts hepatotoxicity [133]. Complex metabolic pathways affected by multi-locus genetic polymorphisms, and possibly age, are important.
Whether azathioprine (or 6-mercaptopurine) predisposes to the development of a rare lymphoma arising in the liver, hepatosplenic T-cell lymphoma (HSTCL), in adolescents and young adults continues to be an important but contentious clinical issue [134–136]. This treatment is prevalent among HSTCL patients, but other factors such as genetic predisposition may be important; combination with a TNFα-inhibitor does not appear to be essential for development of this lymphoma.
Bosentan and Related Drugs
Pulmonary hypertension, while generally rare, is a feature of Eisenmenger syndrome, thus relevant to pediatric practice. Three different endothelin receptor antagonists have become available for treating pulmonary hypertension: bosentan, ambrisentan, and macitentan [137]. Both bosentan and ambrisentan interact with the bile salt export pump [138]; macitentan undergoes hepatic biotransformation via CYP3A4. Hepatotoxicity mainly reflected by abnormal serum aminotransferases has been reported [139]; more severe hepatotoxicity may occur [140]. Children may tolerate bosentan better than adults [141]. One child has been reported who developed “autoimmune hepatitis” while taking bosentan: this may have been an AIH-like hepatotoxicity [142]. Bosentan is a sulfonamide analogue whereas ambrisentan is not: this difference may account for differences in hepatic tolerability. It raises the possibility of cross-reactivity for those who do not metabolize sulfa drugs effectively.
Carbamazepine
Carbamazepine is a dibenzazepine derivative, similar structurally to imipramine in that it has fundamentally a tricyclic chemical structure. Hepatotoxicity is uncommon. In children the usual clinical picture has been hepatitis, typically associated with a drug hypersensitivity syndrome similar to that of phenytoin (thus also similar to DRESS). Two children presented with a mononucleosis-like illness consisting of rash, lymphadenopathy, hepatosplenomegaly, and neutropenia. A child treated at the Hospital for Sick Children, Toronto, presented with fever, rash, incipient liver failure, lymphopenia, and eosinophilia. Rechallenge of her lymphocytes in vitro with metabolites of carbamazepine provided evidence of defective detoxification mechanisms [143]. Numerous reports describe carbamazepine hepatotoxicity in children presenting as the systemic drug hypersensitivity/DRESS syndrome; most instances have occurred in children 6 to 14 years old.
Even more severe hepatotoxicity has been reported in children. One child died of progressive liver failure when carbamazepine was not stopped [144]. Four children with fatal acute liver failure were taking carbamazepine, phenytoin, and primidone [145]. More recently severe hepatitis was reported in three children taking only carbamazepine: one recovered with corticosteroid treatment, but the others died or required liver transplantation [146]. Another child developed severe hepatitis with coagulopathy five months after beginning treatment with carbamazepine; she survived with prednisone treatment [147].
Hepatic biotransformation of carbamazepine is complicated. Like phenytoin and phenobarbital, carbamazepine may be metabolized via arene oxides. Carbamazepine 10,11-epoxide is known to be an important electrophilic metabolite of carbamazepine, and can serve as a toxic metabolite forming protein adducts. These metabolites are ordinarily detoxified by the phase II enzyme epoxide hydrolase. Persons with an inherited defect in biotransformation, possibly involving an abnormal epoxide hydrolase, may be unable to neutralize toxic metabolite(s) of carbamazepine and thus develop hepatotoxicity. The same abnormality in biotransformation creating susceptibility to carbamazepine hepatotoxicity may set up cross-susceptibility to phenytoin and phenobarbital hepatotoxicity [148, 149]. This may explain the fatal hepatotoxicity reported in some children on multiple antiepileptics because primidone contains phenobarbital. A recent report indicates that carbamazepine can undergo microsomal (that is, CYP450-associated) biotransformation to produce reactive metabolite(s) capable of forming protein adducts [150]. Another proposed mechanism is that carbamazepine is metabolized to a reactive iminoquinone species which can react with glutathione [151]. Immunogenetics also influences susceptibility to the carbamazepine hypersensitivity syndrome including liver injury: namely, HLA-A*3101 allele in Northern European ethnicity [152]. HLA-B*1502 allele in Han Chinese and other Asian ethnicities appears related mainly to dermatological reactions. Although there are few data in the pediatric age-bracket about these susceptibilities, it seems prudent to take similar precautions, as is the practice in adults. [153]. Carbamazepine treatment for liver disease associated with α-1-antitrypsin deficiency may encounter typical hepatotoxicity, possibly modulated by underlying liver injury.
Oxcarbazepine, a keto analogue of carbamazepine, can cause similar hepatotoxicity in children [154], and in one case acute liver failure occurred [155].
Cocaine
Cocaine hepatotoxicity has not yet been reported in children or adolescents. A clinically severe hepatitic reaction was reported in five young adults: the predominant histologic finding was extensive zonal necrosis of hepatocytes in Rappaport zone 3 with zone 1 steatosis [156]. The mechanism of this hepatotoxicity remains undetermined. The histologic pattern of hepatic injury in humans is consistent with generation of a toxic metabolite. Cytochromes P450 in the CYP3A subfamily are important in biotransformation of cocaine.
Infants born to cocaine-addicted mothers may be small for gestational age; rarely, spontaneous intestinal perforation has been reported. The possibility of adverse effects on hepatic structure or function in the newborn has received scant attention.
Cyclosporine
Cyclosporine has a novel cyclic structure composed of 11 amino acids and is extremely lipophilic. It is metabolized in humans by CYP3A4 and is, therefore, susceptible to predictable drug interactions that might affect its hepatotoxic potential. Although at high dosage a mixed hepatitic-cholestatic picture may develop, the more frequent hepatic abnormality is bland cholestasis: conjugated hyperbilirubinemia without other evidence of hepatocellular damage [157]. The possibility that basiliximab combined with cyclosporine is more hepatotoxic in children than either alone has been raised [1].
Ecstasy
The synthetic amphetamine 3,4-methylenedioxymethamphetamine (MDMA) is generally known as “Ecstasy” and continues to be a popular “recreational” drug, despite being potentially very hazardous. Deaths have occurred with ingestion of only one tablet. It can cause severe hyperthermia with rhabdomyolysis, cardiac damage with arrhythmias, disseminated intravascular coagulation, and acute renal failure. Hepatotoxicity, reported mainly in young adults, led to death or liver transplantation in several; recent reports of severe hepatotoxicity included some adolescents [158–161]. A few days may elapse after taking Ecstasy before becoming unwell, or patients may be found “collapsed” within hours of taking it. Some patients have coagulopathy and hypoglycemia without developing full-blown acute liver failure. Liver histology is variable in Ecstasy hepatotoxicity. The spectrum ranges from focal to extensive hepatocellular necrosis, with variable degrees of cholestasis, sometimes microvesicular steatosis. Interindividual variation in susceptibility is a prominent feature of Ecstasy hepatotoxicity; hyperthermia itself, impure drug, or co-administered recreational drugs or ethanol may contribute to the liver damage in some cases. Genetic predisposition involves the CYP2D6 variants with decreased activity. Certain drugs (such as paroxetine and fluoxetine) inhibit CYP2D6, and indeed Ecstasy inhibits its own biotransformation by CYP2D6. The mechanism of liver injury includes CYP3A4 as well as CYP2D6, interaction of drug with PXR regulating CYP3A4 and cellular stress responses, and glutathione as the principal phase II detoxifier; in vitro data indicate that Ecstasy or its metabolites can mobilize cytokine-mediated immune responses [162]. The occurrence of Ecstasy hepatotoxicity with acute liver failure in adolescents depends on emerging trends for teenage usage. Public education on the risks of using Ecstasy continues to be required.
Other less acute or less severe patterns of clinical liver disease may occur [163]. A teenager with chronic hepatitis due to Ecstasy showed giant cell hepatitis on liver biopsy, possibly a manifestation of an AIH-like process [164]. Several cases of toddlers taking an Ecstasy pill by accident have been reported, without allusion to liver disorder [165].
Recently other drugs have become available as alternatives to Ecstasy. These include piperazine-derived “designer drugs,” cathinones and other synthetic amphetamines, and methamphetamine [166]. Hepatotoxicity may not be prominent. Hepatic biotransformation and potential hepatotoxicity of these substances are subjects of ongoing research. Importantly, some street drugs are cut with hepatotoxic agents.
A single case of hepatitis associated with the amphetamine derivative lisdexamfetamine, a relatively new treatment for ADHD, was reported in a 14-year-old boy [167]. It resolved when the drug was stopped; however, corticosteroids were given briefly because the initial working diagnosis was AIH.
Erythromycin
All forms of erythromycin, not just erythromycin estolate, are potentially hepatotoxic [168–171]. The clinical presentation is similar regardless of which erythromycin ester is involved: anorexia, nausea, jaundice, and abdominal pain, predominantly in the right upper quadrant. Pruritus due to cholestasis has been reported in adults. The overall clinical appearance is that of a mixed hepatitic-cholestatic process, although the cholestatic component may be prominent enough to suggest biliary tract obstruction. Hepatomegaly, sometimes with splenomegaly, appears to be common in children. A single report of erythromycin ethylsuccinate hepatotoxicity in a child indicated relatively mild, self-limited disease [169]. Histologic findings include prominent cholestasis and focal necrosis of hepatocytes, both of which tend to be worse in acinar zone 3. Eosinophils are prominent in portal infiltrates and in the sinusoids [168]. The zonality suggests the action of a toxic metabolite.
The mechanism of erythromycin hepatotoxicity remains obscure. Erythromycin and other macrolide antibiotics are metabolized in the liver by the CYP3A subfamily. Hepatocellular damage may be caused by a toxic metabolite, but this is by no means proved. New modeling methodology may point to critical differences in hepatotoxic mechanisms among various macrolides [172]. For erythromycin, inhibition of bile acid transporters appears to be important.
Other macrolide antibiotics are also associated with hepatotoxicity. Numerous cases of azithromycin hepatotoxicity have been reported in children [1, 173]. Azithromycin was associated with cholestatic liver injury in two children: in one of these it was associated with a systemic drug hypersensitivity and ductopenia [24]. Azithromycin does not inhibit cytochromes P450, and the hepatotoxic mechanism has not been determined. It is likely a contingent hepatotoxin, certainly capable of eliciting immunoallergic response. Notably, it has limited clinical utility, despite convenient dosing and good palatability. Severe cholestatic liver injury associated with clarithromycin was reported in a 15-year-old girl; it was unimproved by treatment with ursodiol but subsided with prednisone [174]. Some adult experience suggests that clarithromycin can generate a drug hypersensitivity syndrome. Whether nimesulide contributed to this hepatotoxicity in this case is uncertain; however, cholestatic liver injury has been reported in adults [175]. Telithromycin, a ketolide antibiotic active against resistant pneumococci, may cause early-onset severe hepatitis with abdominal pain, jaundice and ascites, which was fatal in four and required liver transplantation in one of 42 adults reported [176]. It is contraindicated in myasthenia gravis. Its safety in children has not been established. Telithromycin has been withdrawn from some markets, notably in North America.
Estrogens: Oral Contraceptive Pill
Cholestasis is a well-recognized complication of estrogens administered in oral contraceptive pills. Estrogen inhibits the bile salt export pump (BSEP); mutations or polymorphisms in ABCB11 may render the pump more susceptible to inhibition [23]. Estrogen-induced changes in bile composition may lead to gallstone formation and diminished gallbladder function.
Hepatic vein thrombosis (Budd–Chiari syndrome) has been associated with use of oral contraceptives. Importantly, it occurs in adolescents taking the oral contraceptive pill. Other disorders associated with hepatic vein thrombosis should be excluded. Early diagnosis may be difficult because clinical presentation can be subtle: gradual increase in abdominal girth due to ascites and non-specific changes in liver function tests.
Liver cell adenoma is the principal neoplasm associated with prolonged use of oral contraceptives. Hepatocellular carcinoma is rare [177]. Oral contraceptive-associated adenomas may progress to hepatocellular carcinoma. Uncomplicated liver cell adenomas may regress when the oral contraceptive pill is stopped. Oral-contraceptive-associated adenomas have diverse molecular features: either inactivation of HNF1A or β-catenin activation, or they may be classified as inflammatory adenomas [178]. Germ-line mutations in CYP1B1 may contribute to the development of some liver cell adenomas associated with HNF1A mutation by affecting estrogen metabolism [179]. Peliosis hepatis, which is focal dilatation of the hepatic sinusoids, is another lesion associated with chronic use of oral contraceptives.
Felbamate
Felbamate is sometimes used for treating seizures in Lennox–Gastaut syndrome in children. It has been associated with serious adverse effects: aplastic anemia and, less commonly, acute liver failure. Severe hepatotoxicity, including acute liver failure, has occurred in young children [180]. The mechanism of this hepatotoxicity may involve P450-generated toxic metabolite(s), which then bind to and modify cellular proteins and initiate an immune reaction [181]. CYP2E1 and CYP3A4 appear to play an important role in hepatic biotransformation of felbamate. One toxic metabolite, 2-phenylpropenal, is detoxified by glutathione but is also capable of inhibiting certain glutathione-S-transferases. Felbamate is apparently a contingent hepatotoxin capable of causing an immunoallergic reaction, dependent in part on both the pharmacogenetic and immunogenetic complexion of the individual taking the drug. Serum aminotransferases should be monitored during treatment, and treatment should be terminated if they rise. Although it may be effective for refractory seizure disorders, felbamate is not suitable for anyone with underlying liver disease.
Haloperidol
Haloperidol may be associated with hepatotoxicity, indicated by elevated serum aminotransferases [182]. Cholestasis may dominate the clinical picture, although some degree of hepatocellular damage and eosinophilia may be present. A prolonged severe bland cholestatic reaction, mimicking extrahepatic bile duct obstruction, may develop in children.
Halothane
Halothane hepatotoxicity is classically hepatitic. It may manifest as asymptomatic hepatitis indicated only by abnormal serum aminotransferases in the first or second week after the anesthetic exposure or as severe hepatitis with extensive hepatocyte necrosis and liver failure. Predictors for developing halothane hepatotoxicity in adults include older age, female sex, obesity, and multiple exposures to halothane. Hepatitis associated with halothane is infrequent in children although halothane is often used in pediatric anesthetic practice. Based on large retrospective studies in children, the incidence is approximately 1:80,000–200,000, in contrast to an incidence of 1:4,000–30,000 in adults. A more recent study suggests that halothane hepatotoxicity is less common in both adults and children [183]. Certainly halothane hepatitis occurs in children. Ten cases have been documented in detail in children aged 11 months to 15 years, all of whom had multiple exposures to halothane. Three children died of acute liver failure but all the others recovered [184, 185]. In addition, three cases of halothane hepatitis were found retrospectively [186, 187] as well as three additional children who succumbed to acute liver failure after halothane [188]. Other reports of hepatitis or hepatic failure in children after halothane anesthesia are difficult to evaluate because of inadequate data or the presence of complicated, and thus confounding, systemic disease; these may amount to an additional nine cases.
Halothane is metabolized by various cytochromes P450 and toxic metabolites are generated [189, 190]. Oxidative or reductive metabolic pathways predominate, depending on the prevailing tissue oxygen tension (Figure 22.5). Reductive metabolism generates a toxic intermediate identified as a chlorotrifluoroethyl radical that leads to lipid peroxidation, and oxidative metabolism generates a trifluoroacetyl intermediate that can acetylate cellular membranes, thus generating trifluoroacetyl adducts. The contribution of these complex metabolic systems to human hepatotoxicity remains a matter of some dispute. However, the oxidative pathway is probably predominant in humans. Recent work shows that CYP2A6 and CYP3A4 are associated with the reductive metabolism and CYP2A6 and CYP2E1 (mainly the latter) are associated with the oxidative pathway.
The mechanism of halothane hepatotoxicity involves a connection between cytotoxic damage from toxic metabolites and immunologic phenomena often associated with this hepatotoxicity. The oxidative pathway appears to be associated with hepatocellular membrane damage and immune phenomena typical of the clinical hepatotoxicity syndrome. Patients surviving halothane hepatotoxicity were found to have an antibody to altered hepatocyte membrane constituents. Antibodies to these neoantigens have now been identified in sera from patients with halothane hepatitis [191]. Further studies showed that neoantigens, analogous to neoantigens derived from halothane-treated animals, are expressed in human liver in individuals exposed to halothane [192]. Only one of these neoantigens has been purified and identified; this particular trifluoroacetylated protein is a microsomal carboxylesterase [193]. Kupffer cells may play a role in the process by which the trifluoroacetyl adducts initiate an immune response.
Other volatile anesthetic agents, such as isoflurane and enflurane, are chemically dissimilar to halothane. Occurrence of hepatotoxicity has been reported mainly in adults. A single case desflurane hepatotoxicity in an infant [194] is disputed [195]. A similar case in a 12-year-old with enflurane was reported [196]. In both, acetaminophen hepatotoxicity might have been responsible. Sevoflurane has been implicated twice [197, 198]. Thus these agents cannot be disregarded, but the incidence of hepatotoxicity in children is very low. Underlying metabolic disorder or complex medical conditions may signal increased risk. A contemporary issue is that halothane costs less than these agents. It may be employed preferentially for pediatric anesthesia in healthcare settings where cost containment is a key consideration. Halothane hepatotoxicity may continue to occur.
Herbal Medications
The hepatotoxic potential of herbal medications (also known as CAM: complementary alternative medicine; HDS: herbs and dietary supplements) is important [199, 200]. The pharmacology of these drugs (active ingredient, metabolism, potential drug interactions) is frequently not well understood. Some herbals alter hepatic drug metabolism. The purity and strength of the actual drug used may not be known. How the herbal preparation is actually manufactured can affect potency and potential toxicity. Patients, including adolescents, may take herbal medications intermittently, and they may not report using herbals to physicians. Some may have underlying liver disease: for example, children with chronic hepatitis C are known to take herbal drugs frequently. Since some herbals are administered to promote weight loss, increased use of these agents in children may be encountered, given the current “epidemic” of childhood obesity. Little is known about the effect of hepatic steatosis on the biotransformation of these drugs. Use of herbal medications also displays cultural biases. Herbals may be administered to children to promote good health. Finally, herbal medications can be bought nowadays over the Internet such that a wide range of agents is available, especially to adolescents.
Among herbal preparations the toxicity of certain bush teas containing pyrrolizidine alkaloids, which can cause VOD, are best known. Comfrey is also hepatotoxic because it contains pyrrolizidine alkaloids [201]. Fatal VOD associated with an unspecified herbal medication has been reported in a child [202]. A 13-year-old survived [203]. Tussilago, otherwise known as “coltsfoot,” is noteworthy due to a report of severe fetal liver damage (in effect, neonatal VOD) after the mother took tussilago tea daily during the entire pregnancy [204]. Pyrrolizidine hepatotoxicity in young infants has been reported with other herbs. Importantly, these herbs may be misidentified. An infant with chronic liver disease attributed to tussilago had actually been given alpendost daily over >1 year: this herb has a much higher concentration of pyrrolizidine alkaloids [205]. Chinese herbs including zicao, kuandonghua (= tussilago), qianliguang (Senecio scandens), peilan (Asteracae family) contain pyrrolizidine alkaloids.
Kava kava, which is used to treat anxiety and promote relaxation, has been removed from the market in some countries because of severe hepatotoxicity [206]. In one instance, a 14-year-old girl required liver transplantation [207]. Some controversy persists as to the real risk of hepatotoxicity, which may depend in part on how the kava is extracted. Kavalactones are capable of inhibiting certain hepatic cytochromes P450 [208].
Other herbals established as hepatotoxic include chaparral leaf [209, 210], jin bu huan [211], and ma huang (ephedra) [212]. Green tea extract, taken to promote weight loss, was implicated in acute liver failure in an adolescent male [213]. Germander hepatotoxicity [214] appears to be mediated by diterpenoid toxic metabolites formed through biotransformation by CYP3A4 [215, 216]. Echinacea may have some hepatotoxic potential: acute severe hepatitis was reported in a two-year-old girl [217]. Willow tree bark contains potentially toxic salicylate. Acute liver failure was reported in a child who received a medication containing multiple herbs plus metals [218]. Recent report(s) document that children may develop significant drug hepatotoxicity after administration of traditional Chinese medications [219]. Polygonum multiflora (renamed as Reynoutia multiflora), a treatment for skin disorders, can cause a cholestatic hepatitis [219].
Infliximab
Infliximab is a humanized monoclonal antibody against tumor necrosis factor-α (TNFα) that is increasingly recognized as causing hepatotoxicity in adults: it causes hepatitis often with features of an AIH-like liver disorder or cholestatic hepatitis [220–223]. Occasionally liver injury is severe enough to require liver transplantation. Recently, at least two pediatric cases have been reported with an AIH-like liver injury [224, 225]. It is possible that infliximab-associated AIH-like hepatotoxicity could be mistakenly diagnosed as autoimmune hepatitis sometimes associated with inflammatory bowel disease, an important distinction since management differs. Retrospective review of 659 children with IBD who received anti-TNFα treatment showed that spontaneously resolving serum aminotransferase elevations occurred in 7.7% [225].
Etanercept, another anti-TNFα agent, was associated with development of apparent AIH in a nine-year-old child with juvenile rheumatoid arthritis (JRA) after ten months’ treatment [226]. This may have been AIH-like hepatotoxicity. Clinical features of severity (jaundice and coagulopathy) were present; however, the patient recovered on conventional treatment for AIH, plus immediate discontinuation of the etanercept. Other cases of apparent hepatotoxicity from etanercept and also adalimumab in adults have been reported [221].
Isoniazid
Isoniazid has been associated with a wide spectrum of hepatotoxicity in adults. It is an important cause of drug hepatotoxicity wherever tuberculosis is highly prevalent. The most frequent abnormality is asymptomatic elevation of serum aminotransferases. Overt symptoms of hepatitis (fatigue, anorexia, nausea, and vomiting) indicate severe disease; mortality is greater than 10% in patients with jaundice [227]. On histologic examination, isoniazid hepatotoxicity frequently looks exactly like acute viral hepatitis. Submassive hepatic necrosis or an AIH-like pattern can occur. Hepatocellular damage sometimes has a zonal pattern, which suggests hepatotoxicity involving drug biotransformation.
Isoniazid hepatotoxicity is generally considered to be more common in adults than in children: this assessment is probably inaccurate. Children appear to be at similar or possibly greater risk of significant hepatotoxicity. Numerous reports worldwide describe isoniazid hepatotoxicity, including fatal hepatitic necrosis, in children either being treated for tuberculosis or receiving prophylaxis [228]. In early studies of children receiving isoniazid alone as TB prophylaxis, hepatotoxicity (indicated by abnormal serum aminotransferases) had a 7% incidence in a series of 369 children [229] and a 17.1% incidence in 239 patients aged 9 to 14 years [230]. In adults the incidence of transiently elevated serum aminotransferases is estimated at 10–20%. The overall incidence of symptomatic isoniazid hepatitis in children has been estimated at 0.1–7.1% [231]. Recent reports are highly disparate: some signaling uncommon hepatotoxicity and others drawing attention to significant risk of severe liver injury [27, 232–234]. Factors accounting for some of this variability include isoniazid monotherapy as prophylaxis, severity of the tuberculosis itself, general health and age of the child, availability/effectiveness of medical care, and possibly ethnicity.
A further complication is that active infection demands multi-drug treatment. Evidence from small studies suggests that hepatic dysfunction occurs in children being treated with isoniazid and rifampicin for tuberculosis, with severe hepatitis in approximately 30–40%. Children under two years old may be at greater risk. The contribution of brief sequential courses of streptomycin and ethambutal to hepatotoxicity of isoniazid plus rifampicin is difficult to determine. Inducers of cytochromes P450 may enhance isoniazid hepatotoxicity. Severe isoniazid hepatotoxicity has been found in children treated concurrently with isoniazid and carbamazepine [235]. As in adults, hepatotoxicity typically develops in the first two to three months of treatment; in most children, mild biochemical disturbance may resolve with either no change in dose or else a modest dose reduction. Symptomatic hepatitis (with or without jaundice) necessitates drug withdrawal. Children with more severe tuberculosis seem to be at greater risk for hepatotoxicity, especially if tuberculous meningitis is present. Malnourished children may be at greater risk of hepatotoxicity. The role of dose remains unclear. Comparison of the revised higher dose regimen with previous disclosed no significant increase in hepatotoxicity; however, some children who developed hepatotoxicity were also on antiepileptic drugs [236].
Isoniazid hepatotoxicity appears to be caused by a toxic metabolite. Candidate toxic metabolites include acetylhydrazine and hydrazine; isoniazid itself may cause hepatocellular damage [237]. Acetylation via NAT-2 is important in isoniazid metabolism. Acetylisoniazid or its derivatives have been proposed as toxic, and recent studies indicate that acetylhydrazine, derived from acetylisoniazid, undergoes biotransformation by cytochromes P450, principally CYP2E1, to produce these toxic metabolites. However, NAT2 is involved in multiple steps of isoniazid biotransformation. Consequently, it may be difficult to predict how rate of acetylation contributes to isoniazid hepatotoxicity. Early reports suggest greater risk of hepatotoxicity in rapid acetylators, but recent reports suggest that slow acetylators in the NAT-2 polymorphism appear to be a greater risk [238]. Reports in children have failed to show a clear pattern of hepatotoxicity in relation to acetylator status, but most were underpowered. Other data suggest that CYP2E1 activity is a more important determinant for hepatotoxicity than acetylator status [239]. Chronic use of alcohol leading to induction of CYP2E1 is known to increase the risk of isoniazid hepatotoxicity. Moreover, isoniazid acting as a nucleophile can activate macrophages. Thus isoniazid is a classic contingent hepatotoxin capable of causing an immunoallergic reaction.
Monitoring with direct inquiry for hepatitic symptoms (anorexia, nausea, vomiting, fatigue) and frequent measurement of serum aminotransferases is necessary during at least the first three months of treatment. If typical hepatitic symptoms develop, isoniazid must be discontinued promptly. Continuing isoniazid treatment until jaundice develops is perilous. Extra caution appears to be required if the child is taking an antiepileptic drug concurrently.
Ketoconazole
In contrast to amphotericin, which is rarely associated with hepatotoxicity [240], the oral antifungal drug ketoconazole was found to cause significant hepatotoxicity soon after it was introduced for general use. The initial large review of ketoconazole hepatotoxicity in the USA included two children (a 17-year-old boy and a five-year-old boy, both with chronic mucocutaneous candidiasis) among 54 reported occurrences of which 33 (including both children) were judged as probable or possible cases of ketoconazole-induced hepatotoxicity [241]. Similar series from the UK and the Netherlands included no children. In all series, the hepatic lesion was mainly hepatocellular necrosis, often with a centrilobular predominance, or a mixed hepatocellular-cholestatic injury, but some patients had mainly cholestatic features. The prevailing interpretation of these clinical observations is that ketoconazole-induced hepatotoxicity results from metabolic idiosyncrasy involving a toxic metabolite. The oral preparation has been withdrawn from the market or highly restricted. A topical preparation is still available.
In contrast to both amphotericin and ketoconazole, fluconazole is available for oral dosing over-the-counter and for treatment of clinically severe fungal infection as an intravenous preparation. Fluconazole inhibits CYP2C19 strongly and it also inhibits CYP2C9 and CYP3A4: thus the potential for adverse drug interactions is important. Hepatotoxicity is uncommon: mainly as transient elevations of serum aminotransferases but sometimes as acute liver failure. Systematic review of the published experience with pediatric patients revealed that hepatotoxicity was the most frequent adverse effect reported, often presenting as elevated aminotransferases (typically resolving off drug) [242]. In infants, conjugated hyperbilirubinemia also occurred, notably in preterm infants [242, 243]. Although fluconazole prophylaxis against fungal infection in critically ill neonates remains contentious, the drug appears well-tolerated in these infants [244]. In general, intravenous administration predisposed to toxicity, but the ready availability of oral fluconazole might factor into some drug hepatotoxicity.
Lamotrigine
Lamotrigine is an anticonvulsant drug that can cause the drug hypersensitivity syndrome classically associated with phenytoin [245–247], thus also similar to DRESS. Severity of liver damage is variable, but severe acute hepatitis, including acute liver failure [248], can occur. Severe ductopenia was the predominate lesion in one case [249]. Lamotrigine can be shown to generate an arene oxide intermediate, possibly the toxic metabolite mediating this hepatotoxicity. Children may be more likely to produce this intermediate via cytochromes P450 especially with rapid dose escalation. Therefore, it is a contingent hepatotoxin that is capable of eliciting an immunoallergic response. Cross-sensitivity to phenytoin, carbamazepine and phenobarbital may be present. Ingestion of lamotrigine by a three-year-old resulted in rash and elevated serum aminotransferases [250].
Methotrexate
Methotrexate is a folic acid antagonist which inhibits dihydrofolate reductase. In children and adults it is a therapeutic option for rheumatoid arthritis, psoriasis and inflammatory bowel disease, given orally in a comparatively low dosage over years. In children with ALL, it may be utilized as part of treatment regimens where it is administered in a comparatively high dose intravenously. The potential for hepatotoxicity has to be considered separately for these differing regimens.
Chronic low-dose treatment with methotrexate, as used in psoriasis or certain connective tissue diseases, may cause hepatic fibrosis with steatosis [251]. The histologic appearance may be similar to that of alcoholic hepatitis with fibrosis. Hepatic fibrosis has occasionally been found in children with juvenile rheumatoid arthritis. It is difficult to screen for liver damage by biochemical testing. Serum aminotransferases may not reflect ongoing liver damage and may be normal even after the development of cirrhosis or fibrosis. Using an aggregate of aminotransferase determinations (percentage abnormal over six or 12 months) may compensate for the relative insensitivity of these measurements [252]. Risk factors for the likely development of liver disease proposed for adults (advanced age, chronic ethanol use, obesity, diabetes mellitus, and renal insufficiency) have limited utility for children, except obesity. Although daily administration of methotrexate appears more prone to cause hepatotoxicity, weekly pulse doses are also associated with the development of hepatic fibrosis. Higher cumulative doses are more likely to be associated with hepatotoxicity, but liver damage has sometimes been found at low cumulative doses. The cumulative dose at which hepatotoxicity becomes likely in children has not been determined.
Because of the difficulty in predicting the likelihood of methotrexate-induced liver damage and in detecting it biochemically, regular histologic examination of the liver by liver biopsy has been customary. Liver biopsy before treatment and at regular, often yearly, intervals during prolonged treatment has been advised, but this may involve a considerable number of invasive procedures for a child. The need for such stringent surveillance has been questioned, especially for children with juvenile rheumatoid arthritis. The risk of methotrexate hepatotoxicity in this group appears to be comparatively low. No child in a cross-sectional study of 14 children with juvenile rheumatoid arthritis who had received a methotrexate cumulative dose >3,000 mg or >4,000 mg/1.73 m2 body surface area had significant fibrosis and only one had moderate-to-severe hepatocellular, fatty, inflammatory, or necrotic changes on liver biopsy. In a larger study of 37 liver biopsies from 25 patients with juvenile rheumatoid arthritis, most were normal or near-normal, four had moderate-to-severe fatty or inflammatory changes, and two had mild portal fibrosis. Weak but significant correlations were found between abnormal histology and percent of aminotransferase elevations and body mass index [253]. In similar previous studies normal or near-normal liver histology was found [254, 255]. Two patients with juvenile rheumatoid arthritis treated with methotrexate were reported as developing liver fibrosis [256].
The surveillance strategy must be individualized for each patient. Children with juvenile rheumatoid arthritis on methotrexate should have serum aminotransferases checked frequently (monthly or bimonthly). Those with elevated aspartate aminotransferase or alanine aminotransferase on 40% or more tests in one year should be considered for liver biopsy. Those with other risk factors such as obesity or diabetes mellitus should also be considered for liver biopsy surveillance. Performing a liver biopsy after a large cumulative dose of methotrexate has been taken may be judicious, especially if continued treatment is anticipated. The merits of a pre-treatment liver biopsy should not be disregarded because several studies indicate that hepatic abnormalities may be present before treatment, which would otherwise be (wrongly) attributed to methotrexate hepatotoxicity. This is especially important if the child is overweight or obese.
Children with other indications for methotrexate, such as psoriasis or inflammatory bowel disease, may require closer surveillance, because these guidelines may not apply to other diseases. Critical review of published experience with methotrexate treatment in pediatric inflammatory bowel disease reveal that some degree of hepatotoxicity, indicated by elevated serum aminotransferases, was common [257]. In approximately half of the identified cases, methotrexate was stopped. Evidence-based protocols for using methotrexate in these patients are still needed.
Transient elastography might be an effective modality for monitoring liver injury in patients on chronic methotrexate treatment. It has obvious advantages of being non-invasive and easy to perform. However, reported experience is limited to adults, and those studies involve relatively few patients. Data in children are needed.
Methotrexate is also associated with acute hepatitis. High-dose methotrexate treatment used in some anti-neoplastic treatment regimens may produce acute hepatitis as shown by a sudden rise in aminotransferases [258]. After chronic treatment for malignancy, usually at comparatively low doses, hepatic damage may be relatively mild, with some steatosis and fibrosis; however, severe liver disease with ascites, hepatosplenomegaly, and transient jaundice has been reported.
The mechanism of methotrexate toxicity remains undetermined although a toxic metabolite has been postulated. The poorly soluble metabolite 7-hydroxymethotrexate has been detected after treatment with high-dose regimens and may be associated with renal toxicity from methotrexate. Direct cytotoxicity is another possibility. Studies in rats suggest that methotrexate can damage the bile canalicular transporter multidrug resistance-associated protein-2 (MRP2), possibly contributing to hepatotoxicity. The C677T polymorphism in methylenetetrahydrofolate reductase (MTHFR) may predispose to hepatotoxicity [259]: the utility of this determination in children requires further investigation. Speculation that obesity may predispose to methotrexate hepatotoxicity also needs pediatric data. The mechanism of chronic hepatotoxicity may differ from that of acute toxicity.
Minocycline
Minocycline, a tetracycline derivative used for treating acne in adolescents, has greater potential for causing liver damage than generally appreciated. Specifically, minocycline hepatotoxicity causes AIH-like liver injury. Several cases have been reported in teenagers: hepatitic symptoms, jaundice, elevated serum aminotransferases, and positive antinuclear antibodies were common [260–262]. Another typical presentation was polyarthritis with biochemical hepatitis. A national retrospective review from 2000 to 2010 found ten cases of such injury along with 159 with classic AIH. Close comparison revealed that the minocycline patients (M:F = 3:7) were somewhat younger, tended to have arthralgias at presentation and were all positive for anti-nuclear antibody whereas only 50% were positive for anti-smooth muscle antibodies; 90% achieved remission at one year, with oral corticosteroid treatment [263]. Histologic features resembling AIH may be found on liver biopsy. In many patients, liver damage resolved when the drug was discontinued. Oral prednisone may be administered analogous to treatment for typical AIH: response is usually favorable and steroids can be tapered and stopped completely after adequate treatment (ordinarily one to two years). The rare HLA allele HLA-B∗35:02 appears to be associated with increased risk of developing minocycline hepatotoxicity [264]. Alternatively, acute hepatotoxicity may occur. Two cases of acute liver failure in adolescents have been reported [265, 266]: one died before transplant, and the other underwent liver transplant. Careful monitoring of liver function is indicated whenever minocycline is used chronically. The mechanism of hepatotoxicity is undetermined but includes immunoallergic features.
Microvesicular steatosis caused by tetracycline administered intravenously is currently rare because this treatment modality is uncommon.
Nevirapine
The anti-retroviral drug nevirapine, a non-nucleoside reverse transcriptase inhibitor, has been utilized as prophylaxis against mother-to-infant transmission of HIV. It can cause significant hepatotoxicity in pregnant women receiving it. Though open to some dispute [267], nevirapine can also cause liver injury in the neonate [268]: longer treatment protocols are more likely to cause hepatotoxicity and a classic drug hypersensitivity syndrome may be present. A genetic abnormality in ABCB1, encoding P-glycoprotein, namely c.3435C>T SNP, was associated with hepatotoxicity, as well as variations in CYP2B6 and CYP3A5 [269]. An association between slow-metabolizing genotype CYP2B6 516G>T and nevirapine hepatotoxicity has been found in some studies [270] and not in others [271], highlighting contrasts between different ethnicities.
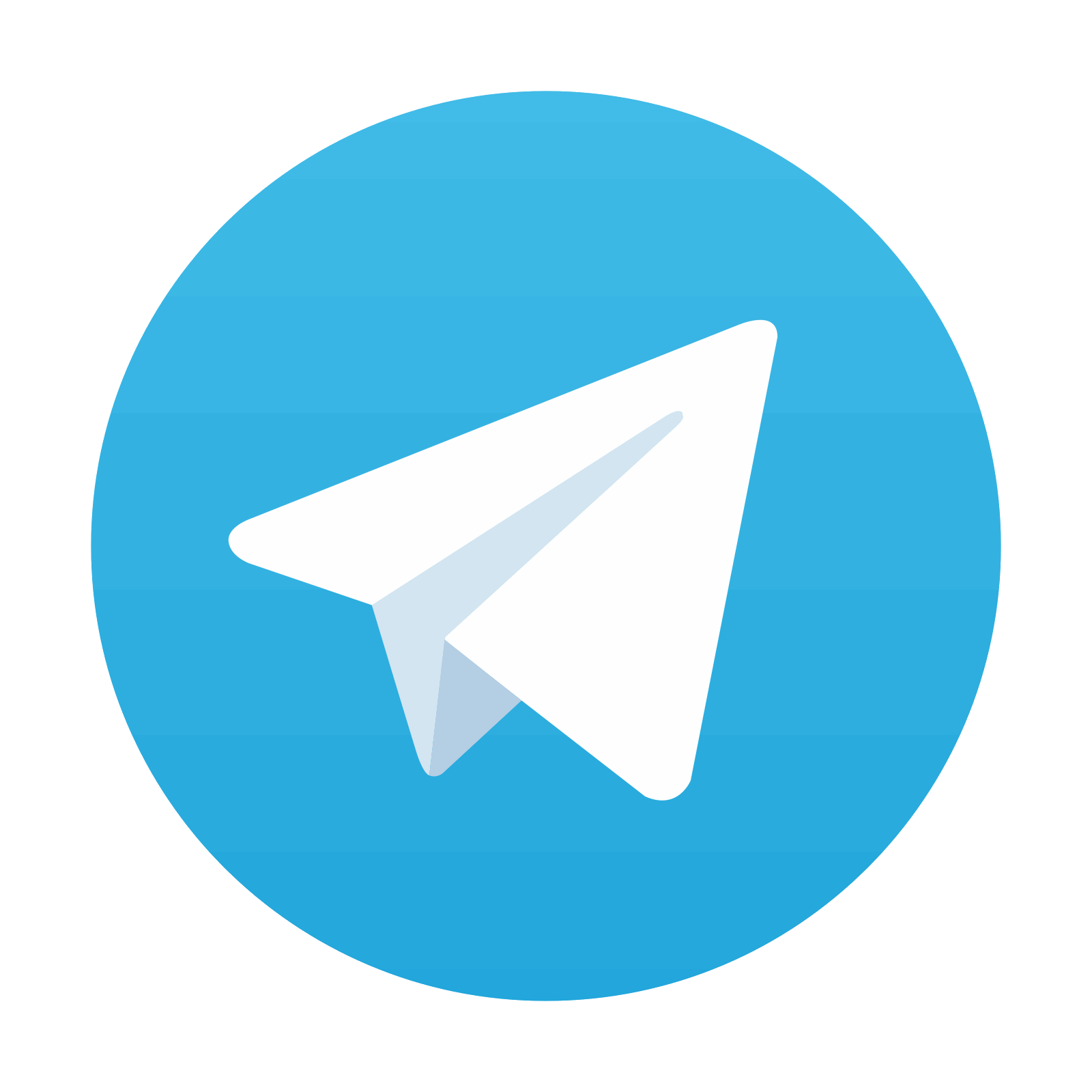
Stay updated, free articles. Join our Telegram channel

Full access? Get Clinical Tree
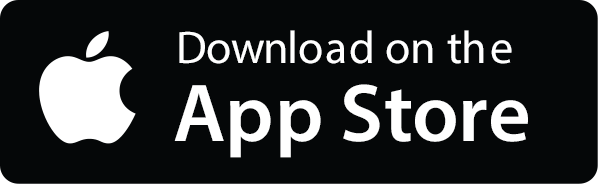
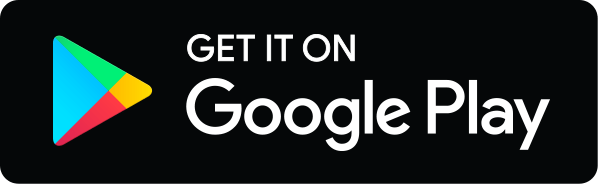
